Abstract
Major depressive disorder (MDD) is a common mental disorder associated with significant suffering and disability. Recent evidence has highlighted the role of the gut-brain axis in the pathogenesis of MDD. Enteric glial cells are a structurally and functionally diverse population that plays a key role in regulating enteric nervous function and maintaining intestinal mucosal integrity. These cells may be implicated in the origin of several digestive and extra-digestive disorders, known as enteric neuro-gliopathies (ENG). This paper reviews the evidence that MDD may also belong to the category of ENG. Animal models suggest that environmental adversity can lead to enteric glial dysfunction and depressive-like behaviors. Conditions that are highly comorbid with MDD, both intestinal and extra-intestinal, have been linked to enteric glial alterations. Peripheral blood markers linked to glial integrity and function are altered in patients with MDD, and certain treatments for MDD may have beneficial effects on enteric glial functioning. Though much of this evidence is indirect and provisional, it suggests that MDD may belong to the group of ENG. Further investigation of enteric glial functioning in MDD may yield valuable insights into the pathophysiology and treatment of this disorder.
Keywords
Major depression, gut-brain axis, gut microbiota, inflammation, stress, enteric glial cells, enteric neuro-gliopathyIntroduction
Major depressive disorder (MDD), also known as major depression, depressive disorder, or clinical depression, is a common mental disorder characterized by pervasive low mood and associated changes in thought, behavior, and biological functions [1]. At least 350 million individuals suffer from MDD, and it is projected to become the leading contributor to the global burden of disease burden at a global level by 2030 [2]. The etiology and pathogenesis of MDD are complex and incompletely understood. Broadly, MDD can be seen as arising from an interaction between genetic vulnerability and exposure to stressful life events [3]. However, MDD is associated with dysregulation of several biological systems, such as the immune system, the hypothalamic-pituitary-adrenal (HPA) axis, and cardiac autonomic regulation [4–6].
In the past two decades, several researchers have found an association between MDD and dysfunction of the gut-brain axis [7]. The term “gut-brain axis” refers to a complex system of two-way communication between the central nervous system (CNS) and the enteric nervous system (ENS) [8]. The ENS is made up of over 500 million neurons, arranged in thousands of ganglia, and an even larger number of glial cells. These function together as a complex system that integrates a wide range of signals and regulates various aspects of intestinal function. For this reason, the ENS has been referred to as a “second brain” [9, 10]. More recently, it has been suggested that depression is related to a three-way interaction between the CNS, ENS, and the intestinal microbial flora, collectively known as the “microbiota-gut-brain axis”. Communication between these three components involves several molecules, including conventional neurotransmitters, immune-inflammatory mediators and metabolites produced by gut bacteria. These send and transmit signals via the vagus nerve and the immune system [11, 12]. External factors such as stress and diet can influence gut-microbiome-brain “cross-talk” and alter the risk of developing major depression [13, 14].
Enteric glial cells and enteric neuro-gliopathies
When considering gut-brain axis interactions, it is important to note that the ENS is composed both of enteric neurons and of a much larger number of enteric glial cells. Though sharing a common origin from the neural crest during embryonic development, enteric glia are structurally and functionally diverse. Four subtypes (types I–IV) have been characterized in mammalian species, with specific localizations in the gut wall. These subtypes can be well characterized in biopsy specimens in terms of morphology, location, and expression of specific proteins. Type I enteric glia are “protoplasmic”, with shorter processes, and express the glial fibrillary acidic protein (GFAP) as a marker, while type II glia are “fibrous”, with longer processes that come into contact with enteric neurons but do not envelop them. Type III glia are seen in the extra ganglionic region, and their processes have been found to cover blood vessels. Type IV glia have a “bipolar” shape and are located in the smooth muscle layers of the gut, along the length of enteric nerve fibers [15]. In living organisms, enteric glia exhibit “phenotypic plasticity”, and can alter their functioning in response to stimuli such as gut peptides or mucosal injury [16]. In the past, it was thought that the main function of these cells was to provide structural and nutritional support to neurons. It is now understood that enteric glia responds to external stimuli, regulate neuronal functioning, and influence the structural integrity and functioning of epithelial cells [17]. This “bidirectional” communication between enteric glia and neurons appears to modulate gut motor functioning [18]. In addition, enteric glia have wider roles in gut homeostasis, including interactions with intestinal stem cells and immune cells such as lymphocytes and macrophages [19].
In light of these findings, it has been suggested that enteric glial cells play an important role in the pathophysiology of certain intestinal disorders, such as inflammatory bowel disease (IBD), irritable bowel syndrome (IBS), and chronic constipation. Bassotti et al. [20, 21] have suggested that conditions previously described as “functional” bowel disorders may in fact be related to structural or functional alterations in enteric glia and their interactions with enteric neurons. Research in patients with functional bowel disorders has provided preliminary support for this hypothesis. In IBS, there is evidence of altered expression of cellular markers on enteric glia, and of altered interactions between these cells and mast cells [22, 23]. In chronic idiopathic constipation (CIC), reduced numbers of enteric glial cells have been demonstrated in both Meissner’s and Auerbach’s plexuses [24, 25]. Thus, these disorders could be considered “enteric neuro-gliopathies” (ENG) [20].
Extra-digestive ENG
The concept of ENG has primarily been applied to disorders of gut functioning. However, there is evidence that enteric glia could contribute to the pathophysiology of disorders not primarily involving the gut. Such conditions could be considered “extra-digestive” ENG [26]. Given the intimate and complex connections between the functioning of the ENS and the CNS, it is possible that some disorders with primarily neuropsychiatric manifestations could be influenced, at least in part, by the dysfunction of enteric glial cells. Enteric glia have been shown to release a number of neurotrophic factors in vitro, including brain-derived neurotrophic factor (BDNF), glial cell line-derived neurotrophic factor (GDNF), and nerve growth factor (NGF) [27–29]. These molecules are essential in promoting neural survival, differentiation, and connectivity [30]. Enteric glia have been shown to exert beneficial effects in cellular or animal models of several neurological disorders, including spinal cord injury [30], Alzheimer’s disease (AD) [31], and multiple sclerosis [32]. It has been suggested that signaling between enteric glia and astrocytes in the brain is implicated in the pathogenesis of some neurodegenerative disorders, such as Alzheimer’s dementia and Parkinson’s disease (PD), that are strongly associated with MDD [33, 34]. It is plausible that enteric glia are implicated in the pathogenesis of depression, but this hypothesis has not been extensively investigated to date [35]. The purpose of the current review is to examine and critically evaluate pre-clinical and clinical evidence for a possible role of enteric glia in the etiology and pathophysiology of MDD, including the possibility that MDD may be an “extra-digestive” ENG.
Enteric glia in animal models relevant to depression
Though no studies have examined alterations in enteric glia in human patients with MDD, there is evidence that these cells may be linked to the genesis of depressive-like behaviors in animal models. In rats, exposure to chronic experimental stress was associated with increases in the number of enteric glial cells expressing GDNF. This led to changes in the activity of specific neurons in the ENS, leading to altered intestinal barrier function and alterations in the gut microbiome that could be relevant to the pathogenesis of MDD [36]. A study of rats exposed to a similar stressor found evidence both of increased GDNF expression and of immune activation in enteric glia. This could be attenuated by pre-treatment with the neuropeptide hormone oxytocin [37]. In mice fed a high-fat diet for five months, enteric glia showed altered expression of GDNF and of the immune receptor toll-like receptor 4 (TLR-4). These changes were associated with reduced BDNF levels, reduced numbers of dendritic spines in ENS neurons, and depressive-like behaviors. All these changes could be prevented by pre-treatment with the drug fluorocitrate, which is toxic to enteric glia [38]. In a more detailed investigation carried out in mice, it was found that stress-induced release of glucocorticoids was associated with increased expression of genes related to inflammation in enteric glia. This was associated with microscopic and behavioral evidence of colonic inflammation. Such changes were not seen in mice where the glucocorticoid receptor gene nuclear receptor subfamily 3 group C member 1 (NR3C1) was deleted, or in transgenic mice with lower numbers of enteric glia [39]. It has also been found that exposure to more severe forms of stress causes greater functional changes in enteric glia. This was demonstrated in a study of male rats exposed to maternal separation in childhood, experimental stress in adulthood, or both. Rats exposed to both forms of stress had a two-fold increase in morphological changes in enteric glia compared to those exposed to a single stressor [40].
Overall, these studies suggest that chronic stress or unhealthy diets can lead to morphological and pro-inflammatory changes in enteric glia, leading to intestinal inflammation, gut dysbiosis, and reduced structural and functional integrity of the ENS. In turn, this can cause alterations in CNS functioning leading to depressive-like symptoms. Moreover, the effect of stress appears to be dose-dependent and can be reversed by pharmacological manipulation. In animals not exposed to stress, enteric glia do not exhibit pro-inflammatory activity; instead, they protect enteric neurons against oxidative stress [41, 42]. Though the results of these studies require replication and extension to human subjects, they provide a plausible physiological pathway through which enteric glia act as “stress transducers” and induce depression via alterations in gut-to-brain signaling. This process is illustrated graphically in Figure 1 below.
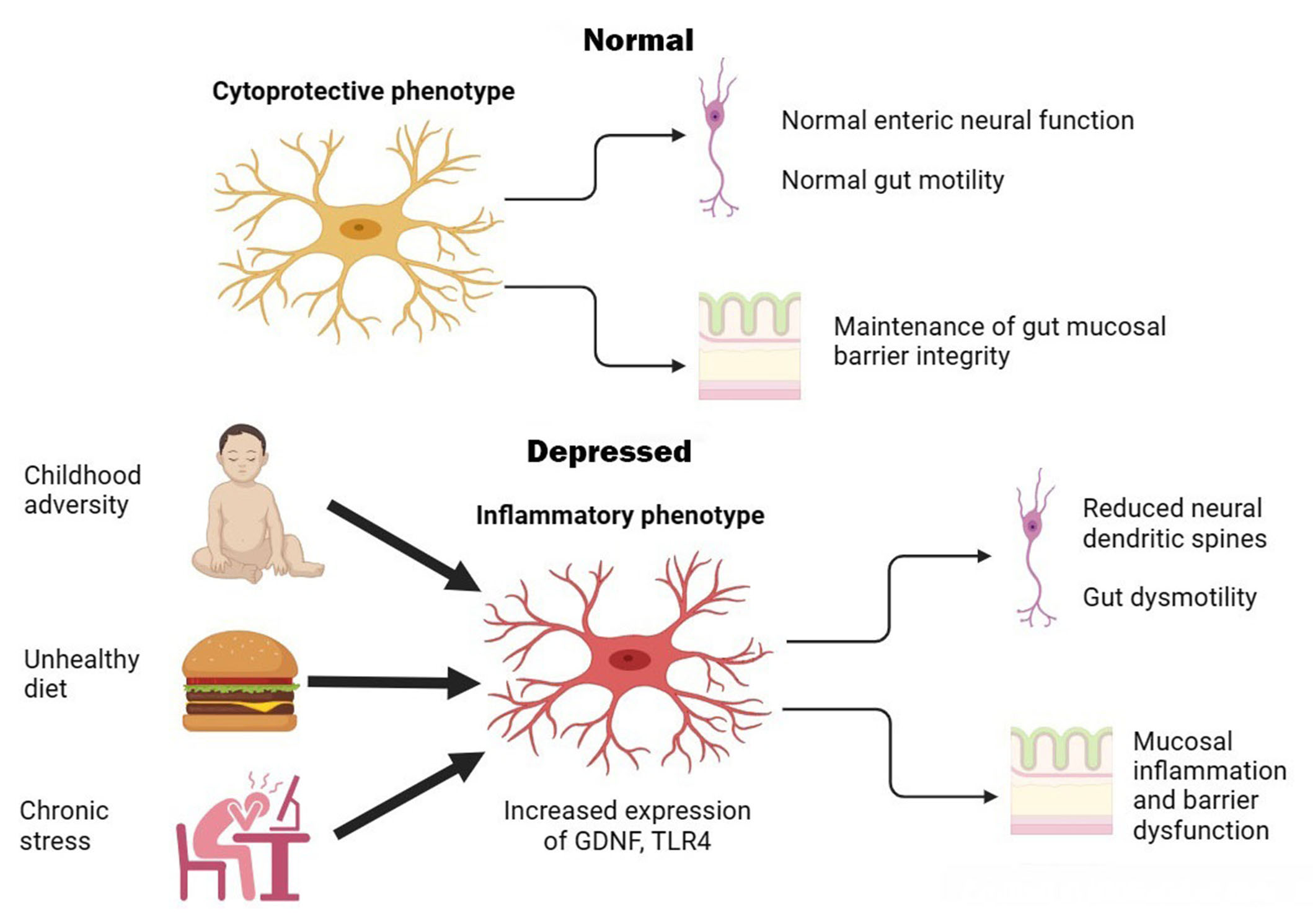
Graphical illustration of putative enteric glial changes in MDD. In health, enteric glia exerts regulatory effects on enteric neurons and protects neurons and epithelial cells against oxidative stress. Exposure to environmental factors can shift enteric glia to an activated or inflammatory phenotype, leading to gut dysmotility, inflammation, and barrier dysfunction that are associated with symptoms of MDD
Enteric glial dysfunction in disorders commonly associated with depression
There is evidence to suggest that enteric glial structure and functioning are altered in several clinical disorders that are highly comorbid with depression. These associations have been demonstrated in both intestinal and extra-intestinal disorders, and are considered in detail in this section.
Intestinal disorders
IBS
IBS is a functional gastrointestinal disorder that is highly comorbid with MDD. About 23% to 29% of patients with IBS have symptoms of MDD, and the risk of MDD is increased 3–7 fold in patients with IBS when compared to the general population [43, 44]. This relationship is bidirectional: patients with MDD are 2–2.5 times more likely to have IBS, and this association is specific to MDD and not to other mood disorders, such as bipolar disorder [45]. As noted in the introductory section, there is some evidence of altered enteric glial physiology in patients with IBS [22, 23]. In a rat model of IBS, exposure to multiple stressors was associated with increased overlap between enteric glia and neurons, and this was associated with greater IBS-like symptoms. These changes are similar to those noted in rodents where IBS was not the focus of the investigation, and could be partially blocked by administration of fluorocitrate [46]. Similarly, exposure to stress in male rats was associated with a decrease in enteric glia positive for S100 calcium-binding protein B (S100B), a neurotrophic factor; this was associated both with symptoms of IBS and with reductions in specific groups of submucous and myenteric neurons [47].
A study of colonic biopsy specimens in human patients with IBS yielded results very similar to those seen in animal models: immunoreactivity for S100B in enteric glia was markedly reduced, and this was correlated with symptom severity. It is noteworthy that 40% of IBS patients in this sample suffered from MDD [48]. In a study of human patients reporting IBS after exposure to the anti-platelet drug clopidogrel, drug-induced IBS was associated with higher levels of pain symptoms considered to be related to psychological factors such as stress, depression, or anxiety. Female patients with these symptoms showed a higher level of expression of the clopidogrel receptor platelet adenosine diphosphate (purinergic) receptor (P2Y12R) in enteric glia than in enteric neurons on biopsy. This suggests that altered enteric glial function may be related both to IBS and to its psychological concomitants in women [49].
Though much of this evidence is at a preliminary level, it suggests that both IBS and its association with MDD could be linked to dysfunction of enteric glial cells. This could be due to reductions in specific glial cell populations, or to altered enteric glial physiology caused by stress- or depression-induced inflammation [50].
IBD
MDD is specifically associated with an increased risk of IBD and often predates the onset of IBD by up to five years [45]. Given the role played by enteric glial cells in gut functioning, structural integrity, and inflammation, it is plausible that they are relevant to the pathophysiology of IBD. In a mouse model, experimental ablation or immune-mediated damage to enteric glia was associated with extensive small bowel inflammation, similar to that seen in Crohn’s disease [51, 52]. Mice with experimentally-induced depressive behaviors were susceptible to IBD-like colonic inflammation following vagotomy, and these changes were reversed following treatment with a tricyclic antidepressant [53]. This effect was found to be mediated by intestinal macrophages, whose functioning is regulated by enteric glia [54, 55].
Direct evidence linking enteric glia to depression in IBD in humans is not yet available. However, markers of inflammation have been associated with elevated depressive symptoms in patients with IBD. This link was observed only in patients with a prior history of MDD [56]. Intestinal biopsies of patients with IBD have shown evidence of impaired enteric glial functioning, resulting in reduced intestinal barrier integrity [57, 58]. Recent research in patients with MDD has found evidence of intestinal barrier damage [59, 60].
Overall, these findings suggest that both IBD and depression are associated with impaired intestinal barrier integrity and that this may be related to impairments in enteric glial regulation of immune-inflammatory responses. Enteric glial dysfunction could explain the bidirectional link between these disorders.
Chronic constipation
About 6–14% of the general population suffers from chronic constipation for which no clear cause can be found. This condition is termed CIC [61, 62]. Like IBS, CIC is considered to be a “functional” gastrointestinal disorder related to stress or lifestyle factors. CIC is one of the first conditions to be considered an ENG, in view of preliminary evidence of glial dysfunction in biopsy specimens [20, 24, 25]. CIC is strongly associated with MDD [63]. The presence of severe depressive symptoms is associated with a 2.5-fold increase in the prevalence of CIC [64], while prior CIC is associated with a 2-fold increase in the risk of MDD [65]. Around 30–40% of patients with CIC have significant symptoms of MDD [66, 67]. In patients with MDD and no formal diagnosis of CIC, 8–25% have chronic constipation [68–70], and an association between severe (“psychotic” or “melancholic”) MDD and constipation is well-documented in the psychiatric literature [71].
Alterations in enteric glial cells in relation to constipation have been documented by several researchers. Enteric glia plays a key role in animal models of chronic constipation induced by opioids. At the cellular level, this effect appears to be mediated through increased purinergic receptor activity, connexin-43 expression, and increased release of pro-inflammatory cytokines; at the tissue level, this is associated with low-grade colonic inflammation [72, 73]. Mice with chronic constipation induced in this way exhibit depressive-like behaviors, which improve when constipation is treated [74].
In human subjects, reduced numbers of S100B-positive enteric glia in the myenteric plexus have been associated with age-related idiopathic constipation [75]; these changes are similar to those documented in IBS associated with depression [48]. In patients with CIC, evidence of chromosomal anomalies in enteric glia, and of increased proximity between glia and degranulated mast cells, has been obtained [76, 77].
The available evidence on enteric glia and their relationship to depression in CIC does not lend itself to a straightforward synthesis. However, it is still possible that the enteric glial dysfunction seen in CIC may lead both to colonic inflammation and reduced intestinal motility. Both these alterations can increase the risk of MDD.
Extra-intestinal disorders
PD
PD is a neurodegenerative disorder characterized by symptoms of resting tremor, bradykinesia, rigidity, and postural instability, with associated cognitive, behavioral, and somatic changes. Classically, PD has been considered to arise from the abnormal accumulation of alpha-synuclein in Lewy bodies, with associated inflammation and degeneration of nigrostriatal dopaminergic pathways [78]. However, recent evidence has implicated the gut-brain axis in the pathophysiology of PD [79, 80].
There are significant epidemiological and pathophysiological links between PD and MDD. Patients with MDD have a 2-fold increase in the risk of subsequent PD [81], and 30–38% of patients with PD have symptoms of MDD [82, 83]. Gastrointestinal symptoms, particularly constipation, are frequently seen as “non-motor” symptoms of PD, and the presence of constipation in patients with MDD may predict subsequent PD [84, 85]. Gastrointestinal and depressive symptoms are reciprocally and positively correlated in patients with PD [86].
Most research on gut-brain axis dysfunction in PD has focused on alterations in gut microbial flora. Over the past decade, researchers have also identified evidence of alterations in enteric glial functioning. In a mouse model of toxin-induced PD, enteric glial changes included shortening and thickening of cell processes and increased immunoreactivity, which appeared to be correlated with increases in inflammatory markers [87]. In a primate model of PD using the same toxin, increased glial cell density in the small bowel was noted, and this was associated with impaired intestinal contractility [88]. Rodent models using a different toxin to induce PD-like phenomena also exhibited enteric glial changes in the form of reduced immunoreactivity to GFAP and S100B, mitochondrial dysfunction, and production of pro-inflammatory mediators [89, 90]. Administration of aggregates of alpha-synuclein was found to cause enteric glial activation both in vitro and in mouse models, and this was associated with intestinal inflammation and behavioral changes. In mice genetically predisposed to alpha-synuclein accumulation, colonic administration of these aggregates caused both gut inflammation and degeneration of nigrostriatal dopaminergic neurons, with more severe behavioral changes [91]. In a transgenic mouse model of PD, alpha-synuclein deposition in the gut was associated with glial cell activation, increased levels of interleukin-1β and tumor necrosis factor-α, impaired intestinal barrier functioning, and both motor and non-motor manifestations of PD [92]. Exposure to experimental stress exacerbated both enteric glial cell inflammation and CNS changes in a toxin-induced mouse model of PD [93].
In human patients, PD is associated with elevation and hypo-phosphorylation of GFAP in enteric glia [94]. In more advanced stages of PD, these cells are increased in number and density, a histological picture suggestive of reactive gliosis [95]. Serum levels of GDNF, which is produced by enteric glia and has protective effects on both the ENS and the mucosal barrier, are reduced in patients with PD who have constipation as a prominent symptom [96]. Constipation in PD is associated with more severe motor symptoms and depression [97].
On the basis of animal and human evidence, enteric glia seems to be significantly involved in the pathogenesis of PD, particularly its non-motor symptoms. There is an apparent bidirectional link between neuropathological changes and alterations in enteric glia. Though it is not yet clear whether depression in PD is related to enteric gliopathy, or whether enteric glial changes associated with MDD predispose to subsequent PD, both possibilities are consistent with the available data.
Other neurodegenerative disorders
Apart from PD, MDD is also associated with an increase in the subsequent risk of dementia, particularly AD and vascular dementia (VaD) [98]. Recent evidence has implicated gut-brain axis dysfunction in the pathogenesis of both these disorders [34, 99, 100]. There is as yet no human evidence linking depression, enteric glial dysfunction, and these conditions. Enteric glial cells treated in vitro with β-amyloid showed increased release of interleukin-1β and TLR4, and increased glial activation has been seen in a mouse model of AD [101]. These findings suggest a link between enteric glial dysfunction, intestinal inflammation, and neurodegeneration that may be directly or indirectly related to MDD, but this possibility requires further testing.
Other evidence implicating enteric glia in gut-brain axis dysfunction relevant to depression
Similarity between toxin-induced and stress-induced changes in enteric glial function
In a study of human enteric glial cells, exposure to the bacterial toxin lipopolysaccharide (LPS) or to the pro-inflammatory cytokine interferon-gamma (IFNγ) led to these cells shifting to a “reactive” phenotype, characterized by up-regulation of pro-inflammatory genes [102]. These changes are akin to those induced by stress in a murine model [39]. Early life adversity, which is also strongly linked to subsequent MDD, has been shown to alter enteric glial responsiveness to mast cell signals, increase GFAP expression, and reduce the length of glial cell processes in mice [103]. The implications of these findings are two-fold. First, intestinal inflammation may itself shift enteric glia to a pro-inflammatory phenotype, causing a vicious cycle of increased inflammation, barrier dysfunction, and dysbiosis which can lead to depression. Second, early life stress may lead to subtle enteric glial dysfunction, which may predispose both to MDD and to associated digestive and extra-digestive disorders. Stress may act synergistically on enteric glial cells with other factors, such as diet and infection, in the pathogenesis of ENGs, including depression.
Treatment effects
Vagal nerve stimulation
The vagus nerve acts as a key neural link between the gut and the brain [104]. Vagal nerve stimulation (VNS), which is an effective treatment for MDD, has been shown to increase the expression of GFAP in enteric glia, which was associated with protective effects on gut mucosa in a mouse model [105]. In contrast, vagal ablation increased susceptibility to intestinal inflammation in a mouse model of depression, and this was reversed by antidepressant treatment [53]. This suggests that at least some effective treatments for MDD may act through vagal-mediated stimulation of enteric glia and reduction in intestinal inflammation.
Probiotics
The intestinal microbiome plays a key role in regulating the integrity and functioning of enteric glial cells [106, 107]. Patients with MDD have evidence of altered gut microbial flora in the form of altered beta diversity, and treatment with adjunctive probiotics has been shown to reduce depressive symptoms in MDD [108, 109]. Treatment with probiotics in patients with PD is associated with improvements in depression, motor symptoms, and gastrointestinal motility [110]. Though none of the trials of probiotics in these disorders have evaluated enteric glial functioning either directly or indirectly, it is possible that these treatments may exert their beneficial effects at least partly through restoration of enteric glial functioning.
Links between MDD and peripheral biomarkers related to enteric glia
Certain compounds that are measurable in peripheral blood may reflect enteric glial integrity or functioning. For example, serum S100B is significantly reduced in patients with ulcerative colitis (UC), which may reflect enteric glial inflammatory activation [111], while serum GDNF has been associated with constipation in patients with PD and may reflect impaired enteric glial functioning [96]. GFAP has also been proposed as a putative marker of enteric glial activation, though it is not detectable in the serum of patients with UC [111].
There is evidence of altered serum S100B levels in MDD, though the direction of findings has varied across studies. Some researchers have found elevated levels of S100B that correlate with the number and severity of depressive episodes [112, 113]; however, serum S100B may be reduced in youth with initial episodes of MDD, in adult patients with multiple episodes of MDD, or in those on antidepressant treatment [114–116]. There are fewer studies evaluating serum GFAP in depression, but there is some evidence that this biomarker may be reduced in untreated patients with MDD [117]. These findings have been interpreted in terms of brain neuronal integrity or plasticity, but they could also reflect changes in enteric glial number, integrity, or functioning and associated comorbidities over the course of MDD.
No significant alterations in serum GDNF have been identified in patients with MDD, suggesting that this neurotrophin may not be a reliable marker of either depressive symptoms or enteric glial function [118].
Possible serotonergic links between enteric glial functioning and depression
Serotonin plays a key role in the pathophysiology of depression, as well as in the actions of most antidepressant medications [119–122]. This neurotransmitter also plays a key role in the regulation of gastrointestinal functioning: it is estimated that over 90% of plasma serotonin originates from enterochromaffin (EC) cells in the gut [123]. Though serotonin is synthesized independently in the CNS and in the gut, there is evidence of a moderate correlation between central (cerebrospinal fluid) and peripheral (plasma) levels of serotonin [124]. Given that most plasma serotonin is synthesized by EC cells, this suggests a degree of functional coordination between brain and gut serotonergic systems [125]. Alterations in gut serotonergic functioning have been hypothesized to play a role in many of the disorders putatively linked to enteric glial dysfunction, including IBS [126], IBD [127], AD [128], and multiple sclerosis [129]. It has also been suggested that alterations in gut serotonergic transmission may be related to the changes in mood seen in MDD [130, 131]. Like enteric glial cells, the serotonergic division of the ENS shows functional alterations in relation to the composition of the gut microbiome, and may show meaningful responses to probiotic treatments [132–134]. It has been suggested that intestinal serotonin may act as a “continuous regulatory signal” for various organs, particularly for the brain [135].
Evidence linking serotonin to enteric glial function or dysfunction is still preliminary. Enteric serotonergic neurons have extensive contact with glial cells [136]. A subset of enteric glia shows increased intracellular signaling and expression of GFAP when treated with serotonin [137–139]. This activation of enteric glia by serotonin has been shown to occur in response to acute infection or inflammation, where it may represent an attempt to restore homeostasis [139–141]. In addition, activation of the serotonin type 1A (5HT1A) receptor has been shown to protect enteric glia from apoptosis in vitro and animal models of intestinal inflammation [142]. This receptor is of particular interest because functional variations in the gene encoding it have been associated with susceptibility to MDD [143, 144].
Overall, though there is no direct evidence linking serotonergic transmission to enteric glial cell functioning in depression, various lines of evidence suggest that this pathway merits further investigation.
Summary and limitations of the available evidence
The evidence reviewed in this paper is summarized in Table 1 and Figure 2. It provides preliminary support for the concept that enteric glial dysfunction is involved in the pathogenesis of MDD—in other words, MDD may represent, at some level, an extra-digestive ENG.
Evidence of possible enteric glial dysfunction associated with MDD
Source of evidence | Findings |
---|---|
Animal models of environmental adversity | Increased expression of GDNF, TLR-4, inflammatory mediators in enteric glia;Associated reduction in neuronal dendritic spines and mucosal inflammation. |
IBS-animal models | Increased overlap between enteric glia and neurons;Reduced S100B positivity;Reductions in neuronal subpopulations. |
IBS-human studies | Reduced enteric glial S100B immunoreactivity in IBS with comorbid MDD;Increased P2Y12R expression in enteric glia in women with clopidogrel-induced IBS and associated psychogenic pain. |
IBD-animal models | Enteric glial ablation or damage associated with small bowel inflammation;Mice with experimentally induced depression sensitive to colonic inflammation after vagotomy;Reversible with tricyclic antidepressant. |
IBD-human studies | Elevated intestinal inflammatory markers associated with depressive symptoms in IBD with prior MDD;Impaired enteric glial functioning in biopsies; Linked to mucosal barrier impairment similar to that seen in MDD. |
CIC-animal models | Drug-induced chronic constipation associated with altered protein expression and inflammatory mediator release by enteric glia;Associated with depressive-like behaviors. |
CIC-human studies | Reduced numbers of S100B-positive enteric glia;Chromosomal anomalies in enteric glia;Increased proximity between enteric glia and degranulated mast cells. |
PD-animal models | Increased glial cell density;Reduced positivity for GFAP and S100B;Increased release of inflammatory mediators;Associated with gut dysmotility, mucosal barrier impairment, and PD symptoms;Glial changes exacerbated by exposure to stress. |
PD-human studies | Elevated levels and hypo-phosphorylation of GFAP in enteric glia;Reactive gliosis;Reduced serum GDNF;Associated with constipation is linked to depression in PD. |
Effects of VNS | Increased GFAP expression by enteric glia;Associated with mucosal barrier integrity in a mouse model. |
MDD | Elevated serum S100B in adult patients with MDD;Reduced S100B in adolescents with MDD and after antidepressant treatment;Reduced serum GFAP in drug-naïve patients with MDD. |
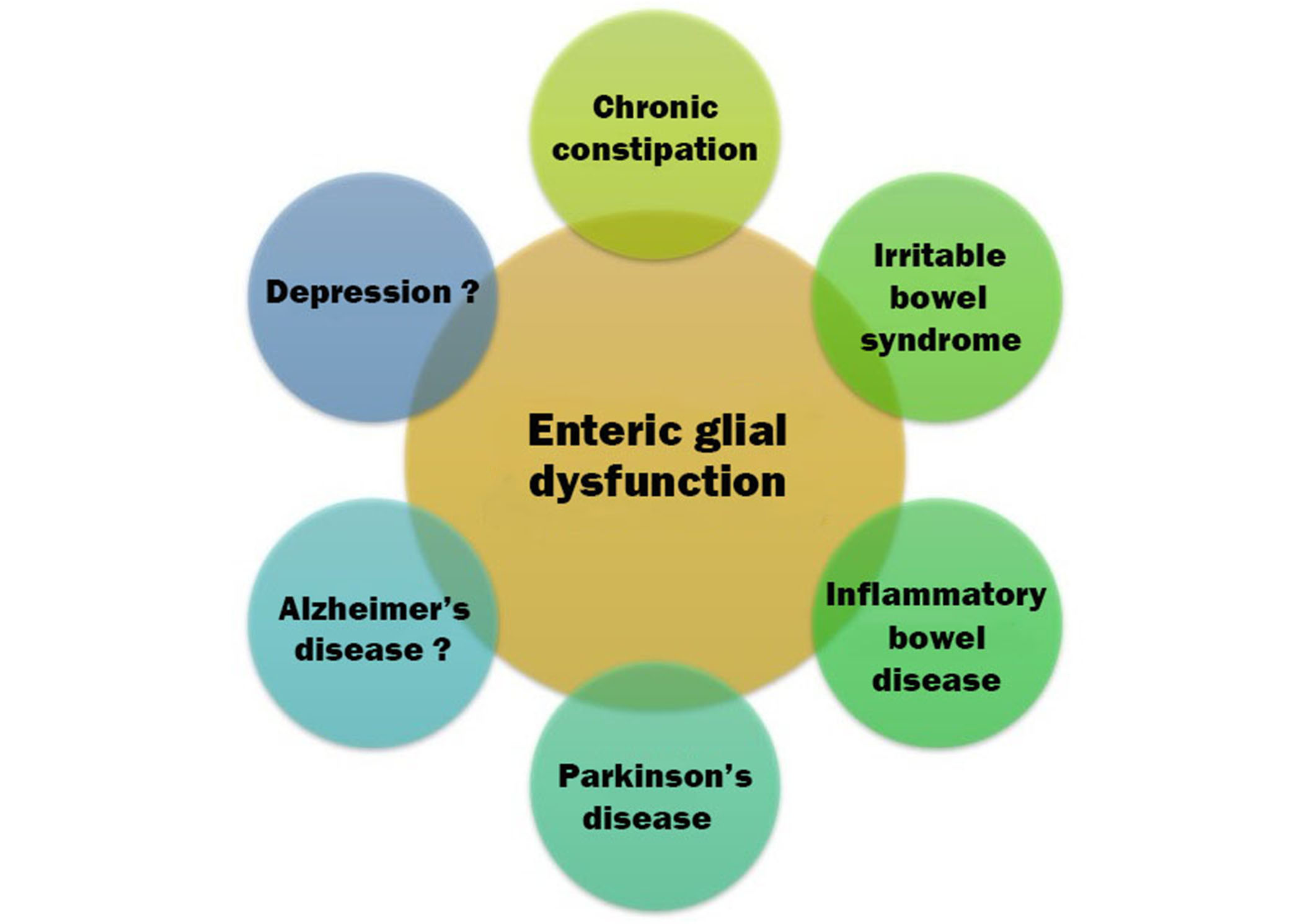
Graphical illustration of disorders that may be ENG. Firm associations have been established with gastrointestinal disorders. As one moves clockwise, toward the neuropsychiatric disorders, associations are more tentative but still plausible
However, certain limitations should also be acknowledged. First, much of this evidence is indirect in nature. There are no human studies that have directly evaluated enteric glial function in MDD, and studies of peripheral blood markers such as GFAP and S100B may not necessarily reflect enteric glial dysfunction. Second, even though there is evidence of enteric glial changes in animal models related to depression, it is not known if these changes are causal or merely correlated with behavioral changes. Third, it is not certain to what extent findings in rodent models can be applied to human subjects. Fourth, in spite of enteric glial dysfunction occurring in many disorders linked to depression, none of the human research on these disorders has specifically linked enteric glial dysfunction to depressive symptoms in these patients. Fifth, it is still not known if these findings apply to enteric glial cells in general, or to only some of the subtypes of enteric glia. Finally, it is possible that even if enteric glial dysfunction is demonstrated in depression, this may be epiphenomenal in nature and the result of more fundamental alterations in gut microbiota or immune-inflammatory functioning. Despite these limitations, the available evidence suggests that enteric glia could act as a link between gut and brain dysfunction in MDD, and this hypothesis needs to be investigated further.
Implications for practice and research
From a clinical point of view, there is no established treatment for depression that is known to affect enteric glial dysfunction. However, evidence from animal models and human subjects with other disorders suggests that four treatment approaches may be of value. First, stress reduction or stress management techniques may reduce the synergistic interaction between inflammation due to stress and other causes that result from enteric glial dysfunction. This approach may be useful in patients with mild MDD occurring in response to life events. Second, probiotics with evidence of beneficial effects on enteric glial structure and function could be used as adjuncts to standard antidepressant therapy or psychotherapy [145]. Third, treatments that directly or indirectly stimulate vagal function (e.g., VNS, some antidepressants) may have a positive effect on enteric glia, shifting them from an “inflammatory” to a “cytoprotective” phenotype. Finally, immune-based therapies could reduce the intestinal inflammation and barrier dysfunction that are putatively linked to “activated” enteric glia, leading to reduced peripheral and central inflammation and alleviating depressive symptoms [146].
From a research perspective, the results summarized in this review highlight the need for specific investigations of enteric glial function in patients with MDD. This could involve either direct examination of intestinal tissue using small bowel or colonic biopsies, or the use of fecal or peripheral blood markers that may reflect enteric gliopathy. If evidence of enteric glial dysfunction is demonstrated and replicated in MDD, it should be studied in association with the onset and course of depression, the presence of comorbid diagnoses or symptoms, and its response to various forms of treatment. Finally, studying the molecular mechanisms associated with enteric gliopathy in MDD-for example, using studies of gene or protein expression could yield new clues about the pathophysiology of this disorder, and could aid the development of novel gut-based therapies in patients who do not respond to conventional treatment.
Conclusions
The concept of extra-digestive ENG is relatively new. Though much of the evidence available is at a preliminary stage, there are several indications that MDD may be related to structural or functional alterations in different populations of enteric glia. While it may be premature to label MDD an ENG, it is highly likely that enteric glia may be a key “player” in the gut-brain interactions that underlie this disorder. Further clinical and translational investigations of enteric glial dysfunction in MDD, and its relationship to the gut and brain changes seen in this disorder, could shed new light on novel molecular and cellular processes linking gut dysfunction to mental health. This, in turn, could lead to the development of safer and more efficacious treatments for MDD.
Abbreviations
AD: | Alzheimer’s disease |
CIC: | chronic idiopathic constipation |
CNS: | central nervous system |
ENG: | enteric neuro-gliopathies |
ENS: | enteric nervous system |
GDNF: | glial cell line-derived neurotrophic factor |
GFAP: | glial fibrillary acidic protein |
IBD: | inflammatory bowel disease |
IBS: | irritable bowel syndrome |
MDD: | major depressive disorder |
PD: | Parkinson’s disease |
S100B: | S100 calcium-binding protein B |
TLR-4: | toll-like receptor 4 |
VNS: | vagal nerve stimulation |
Declarations
Author contributions
RPR: Conceptualization, Data curation, Formal analysis, Investigation, Methodology, Resources, Supervision, Validation, Visualization, Writing—original draft, Writing—review & editing.
Conflicts of interest
The author declares that he has no conflicts of interest.
Ethical approval
Not applicable.
Consent to participate
Not applicable.
Consent to publication
Not applicable.
Availability of data and materials
Not applicable.
Funding
Not applicable.
Copyright
© The Author(s) 2024.