Abstract
Aim:
Diabetic polyneuropathy is the most described complication in patients with diabetes mellitus. A significant percentage of these patients experience disabling neuropathic pain (painful diabetic polyneuropathy). Small nerve fibers are primarily responsible for peripheral nociception, but objectively assessing its function is challenging. The primary objective of this study was to explore the task execution and outcomes of intra-epidermal electrical stimulation technique that combines nociceptive detection thresholds (NDT) and evoked potentials (EPs) in patients with diabetes. We compared the results of diabetic patients, both with and without painful diabetic polyneuropathy, with those of healthy controls to explore potential clinically relevant information.
Methods:
The NDT-EP method was applied to 38 patients with diabetes (18 with and 20 without chronic painful neuropathy) and 38 age- and sex-matched healthy controls. Individual mean NDTs, psychometric slopes, EP amplitudes, and the effect of the stimuli on EP amplitudes were analyzed and compared between groups using linear regression.
Results:
The findings revealed significantly lower detection rates, higher NDTs, and lower psychometric slopes in patients with painful diabetic polyneuropathy than in healthy controls. Both patient groups significantly exhibited lower mean EP amplitudes than healthy controls, which were not linked to pulse amplitudes but influenced by stimulus detection.
Conclusions:
This study showed altered NDT-EP outcomes in patients with painful diabetic polyneuropathy. Whereas the task execution, NDTs, and psychometric slopes may provide valuable insights into small fiber dysfunction, pulse amplitudes seemed not differently encoded in neurophysiological responses to intra-epidermal electrical stimulation near the detection threshold compared to controls. Future studies should investigate whether the altered NDT-EP outcomes could quantify small fiber dysfunction in patients with diabetes mellitus. We recommend further exploration of NDT-EP measures in other patient groups with nociceptive dysfunction.
Keywords
Nociceptive detection threshold, evoked potential, diabetes mellitus, diabetic polyneuropathyIntroduction
Over the last decades, the prevalence and global disease burden of diabetes mellitus increased worldwide [1]. Persistent hyperglycemia plays a fundamental role in causing microvascular complications, of which diabetic polyneuropathy (DPN) is the most common [2]. Up to 50% of diabetic patients will develop neuropathy during the course of their disease [3]. Symmetrical, length-dependent, sensorimotor neuropathy characterizes DPN [1]. Approximately 20–30% of patients with DPN experience neuropathic pain in varying degrees of intensity [4, 5]. Whether painful DPN should be considered a variant of DPN or a pathophysiological disease is a topic of ongoing debate [6]. Moreover, it is unknown why some patients with diabetes experience severe neuropathic pain while others with a similar degree of neuropathy do not [7]. Treatment of late-stage DPN and painful DPN is often unsuccessful, emphasizing the importance of identifying early-stage diabetes mellitus and associated abnormalities in the peripheral somatosensory system, as early glycemic control prevents later-stage complications [6, 8–11].
The most common presentation of DPN and painful DPN is as a small fiber neuropathy [2]. Small nerve fibers (i.e., Aδ- and C-fibers) are primarily responsible for peripheral nociception. While peripheral mechanisms are known to contribute to the development of nerve-related dysfunction, increasing evidence shows the involvement of central mechanisms [12]. Changed nociceptive input due to damaged small nerve fibers induces maladaptive alternations in the central nervous system that cause central sensitization (i.e., altered central nociceptive function) [7].
Conventional neurophysiological testing methods, such as nerve conduction studies, primarily measure large fiber function and thus are limited in detecting small fiber dysfunction [10]. Several specialized diagnostic instruments have been introduced to identify small fiber dysfunction, including corneal confocal microscopy, laser evoked potentials (EPs), contact-heat EPs, plantar sympathetic skin response, assessing sudomotor axon reflex, quantitative sensory testing (QST) and intra-epidermal nerve fiber density (IENFD) measurement [10, 11, 13, 14]. The combination of QST and IENF examination and at least two abnormal clinical signs has been proposed as a diagnostic “gold standard” to confirm a clinical suspicion of small fiber neuropathy [15].
Our group has developed a measurement technique [nociceptive detection thresholds-EP (NDT-EP) method] for observing psychophysical and neurophysiological responses to intra-epidermal electrical stimulation (IES) [16–18]. Nociceptive Aδ-nerve fibers can be selectively recruited using IES [19–24]. The controlled induction of nociceptive input by manipulating electrical stimulus properties such as the strength, number of pulses, and inter-pulse interval (IPI) [23, 25, 26] can temporally increase or decrease neural activity through central mechanisms (e.g., temporal summation or short-term synaptic plasticity [27]) or peripheral mechanisms (e.g., subthreshold or suprathreshold super-excitability [28]).
During the measurement, NDTs are tracked using a Multiple Threshold Tracking method [29]. This psychophysical procedure involves stimulus selection and threshold estimation for single- and double-pulse stimuli, with IPIs of 10 ms or 40 ms [29]. Simultaneously, electroencephalography (EEG) is recorded to measure EPs time-locked to the stimuli, providing insights into the subject’s neurophysiological response. Results showed that EP amplitudes were significantly influenced by stimulus detection, while the detection probability was affected by the pulse amplitude of each stimulus type and the number of trials [16, 17].
Outcome measures such as the NDT, psychometric slopes, and EP components (specifically N1 and P2 amplitudes) may allow indirect observation of the nociceptive function [16, 17, 30]. While NDTs are thought to represent the sensory system’s sensitivity [25, 31, 32] and psychometric slopes illustrate stimulus detection reliability [33], the N1 and P2 amplitudes may serve as neurophysiological biomarkers for evaluating central nociception (e.g., central sensitization) [34] or attentional modulation [35]. However, whether and how NDT-EP outcomes can provide insight into the nociceptive function of patients expected to have altered nociceptive function remains to be discovered.
Our study group provided preliminary evidence supporting the feasibility of using the NDT-EP method in a small sample of diabetic patients (with painful DPN) [36], revealing potential altered nociceptive function in patients compared to healthy subjects. Although promising, the observed differences appeared to be possibly confounded by age and sex. Furthermore, the merits of EEG measures were inconclusive due to the small sample size and potentially impaired task execution of the psychophysical procedure by patients [36].
The primary objective of this study is to further explore the task execution and NDT-EP phenomena in a larger sample of diabetic patients, including comparison with age- and sex-matched healthy controls. Detection rates, response times, NDTs, psychometric slopes, and EP components (e.g., N1 and P2 amplitudes) will be studied.
Materials and methods
Study design
This explorative mono-center case-control study was conducted in the outpatient pain clinic of the St. Antonius Hospital Nieuwegein in the Netherlands. This study consisted of a single measurement session. On the measurement day, subjects completed (pain) questionnaires, including a hand’s numeric rating scale (NRS) score and central sensitization inventory-part A (CSI-A) score. Clinical features, such as the duration of diabetes mellitus (i.e., the time elapsed since the date of diagnosis), the duration of the DPN symptoms (i.e., the time elapsed since the date of DPN diagnosis), and medication intake were reported in a case report form. Afterward, two measurements using the NDT-EP method (for more information, see the procedure and [16, 17, 36, 37]) were conducted on the dorsum of each hand. Whether the first measurement in each subject started on the dominant or non-dominant hand was determined by randomization. NDTs and cortical EPs were obtained for IES.
Subjects
Twenty-two patients with diabetes mellitus diagnosed with chronic painful peripheral polyneuropathy (DMp) and 20 patients with diabetes mellitus without pain or other clinical symptoms of polyneuropathy (DM) were included in this study. No formal sample size calculation was performed because of its explorative character. The patients were recruited at the Department of Internal Medicine and the Department of Anesthesiology, Intensive Care, and Pain Medicine at the St. Antonius Hospital Nieuwegein in the Netherlands. Each patient was matched by a healthy control (HC) based on age and sex from an internal database of 64 healthy subjects who executed the same procedure using the NDT-EP method at St. Antonius Hospital [36].
Patients in the DMp group experienced pain for more than three months. A neurologist made the diagnosis based on clinical examination. Patients in the DM group and HC groups did not experience peripheral neuropathy or pathological pain in history. Possible other causes of polyneuropathy or severe (non-neuropathic) pain complaints were defined as exclusion criteria for diabetic patients. The remaining general exclusion criteria were pregnancy, co-existing central or peripheral nerve disorders, subjects’ refusal during the measurements, incompetence to follow the instructions, alcohol or drug consumption 24 hours before the measurement session, and an implanted electrical stimulation device. The age of patients with diabetes mellitus (i.e., type 1 and 2) and HCs ranged between 18 years and 75 years. The subjects were allowed to take their usual analgesics. The study was approved by the Medical Research Ethics Committees United (MEC-U, file number NL66136.100.18). Verbal and written informed consent were obtained from all subjects.
Procedure
The procedure of the NDT-EP method was equal to that of the previous study by Berfelo et al. [36]. Subjects sat in a comfortable chair and focused on a spot on the wall. The cathodic IES-5 (five 0.2 mm needles) electrode [38] was placed on the dorsum of the hand, which was positioned distal to a 9 × 5 cm anodic transcutaneous electrical nerve stimulation (TENS) electrode. The electrodes were connected to the constant current stimulator (NociTRACK AmbuStim 1-channel, University of Twente, Enschede, the Netherlands), held in the other hand. Aδ-fibers were preferentially activated using the concentric IES-5 electrode. Three types of 210 µs square-wave pulse stimuli were applied: 1) single-pulse stimuli, 2) double-pulse stimuli with 10-ms IPI, and 3) double-pulse stimuli with 40-ms IPI.
Subjects were familiarized with these stimulus types before the start of the NDT-EP method. An upward staircase procedure was performed, starting at 0 mA with 0.05 mA increments, to determine an initial stimulus amplitude for each stimulus type. During the NDT-EP method, subjects were instructed to hold the push button and release at any sensation ascribed to a stimulus. Stimuli were selected using a threshold tracking method that kept the delivered stimulus amplitude around the detection threshold [29]. The stimulus response was labeled as “detected” or “non-detected,” prompting the subsequent stimulus amplitude to decrease or increase with a fixed step of 0.025 mA, respectively. A set of 450 stimuli (i.e., 150 stimuli per stimulus type) was administered during the entire experiment.
Stimulus-related EPs were recorded with a sampling frequency of 1,000 Hz using an ANT Neuro Waveguard EEG cap with 64 Ag/AgCl electrodes (modified international 10-20 system) connected to a TMSi 72-channel Refa EEG amplifier with a common average reference. Electrode impedances were kept below 5.0 kOhm.
Data analysis
Psychophysical and neurophysiological responses to nociceptive stimuli were analyzed in MATLAB (version 2021b; The MathWorks Inc, Natick, Massachusetts, US). The detection rate, response time, and NDT-EP outcomes were calculated per measurement and analyzed for the four subject groups: the DM group, its age- and sex-matched HC group, the DMp group, and its age- and sex-matched HC group.
NDTs and psychometric slopes
A generalized linear regression model was used to estimate the detection probability (Pd) as an outcome variable. The model included the intercept, the amplitude of the first pulse (PU1), the amplitude of the second pulse with 10 ms IPI (PU210), the amplitude of the second pulse with 40 ms IPI (PU240), and the trial number (TRL) as fixed effects (Equation 1).
Mean NDTs and psychometric slopes were calculated per measurement using the estimated model coefficients. NDTs reflected the sensitivity to nociceptive stimulation [25, 31, 32], and psychometric slopes described the reliability of stimulus detection [33].
Evoked potentials
The EEG data were pre-processed using FieldTrip [39], a MATLAB (version 2015b; The MathWorks Inc, Natick, Massachusetts, US) toolbox. A band-pass filter of 0.1 Hz to 40 Hz was applied to the EEG signal. An EP window was selected between 500 ms pre-stimulus and 1,000 ms post-stimulus around the trial trigger points. EEG artifacts due to eye blinks and muscular activity were removed by independent component analysis. The EP was further analyzed in the CPz-A1A2 and T7-F4 derivations, which showed the highest signal-to-noise ratio in the previous study [17]. Grand average EPs for detected and non-detected stimuli were calculated for each group.
Individual mean N1 and P2 amplitudes were computed based on the subject average EEG at T7-F4 (N1 peak) and CPz-A1A2 (P2 peak) for each measurement. The individual mean N1 and P2 amplitudes consisted of detected and non-detected stimuli. The amplitude of the N1 peak was determined by selecting the most negative potential between 130 ms and 200 ms post-stimulus. In addition, the amplitude of the P2 peak was determined by choosing the most positive potential between 300 ms and 500 ms post-stimulus.
For each measurement, the EP components (i.e., N1 and P2 amplitudes) were modeled as a function of an intercept, the amplitude of the PU1, the amplitude of the second pulse with 10 ms IPI (PU210), the amplitude of the second pulse with 40 ms IPI (PU240), and the interaction between the TRL and stimulus detection (D) (Equation 2). TRL explained the potential effect of habituation on brain activity. D described the additional EEG activity elicited by stimulus detection (i.e., how many microvolts the EP amplitude increases or decreases when a stimulus is detected), which could also increase or decrease over the number of trials (TRL * D).
Statistical analysis of NDT-EP outcomes in patients compared to HCs
Subject characteristics (i.e., age, sex, body mass index) and patient-reported outcome measures (i.e., CSI-A score and NRS score) were tested between groups using a t-test. P < 0.05 is considered statistically significant. The effect of the diagnosis state on the NDT-EP outcome (i.e., detection rate, response time, NDTs, psychometric slopes, mean N1 and P2 amplitudes, and effect sizes of the model coefficients on the N1 and P2 amplitudes) was evaluated using linear regression (Equation 3). The NDT-EP outcome was modeled as a function of an intercept and diagnosis group (DM or DMp). The linear model was applied to the entire dataset of each diagnosis group with its respective HC group.
Results
Study population
Thirty-eight of 42 patients with diabetes mellitus completed two measurements using the NDT-EP method (Figure 1). Four patients (two from the DM group and two from the DMp group) could not execute the procedure tasks because they did not feel the stimuli up to a maximum strength of 1.5 mA. Subject characteristics, patient-reported outcome measures, and clinical features of 20 DM with 20 age- and sex-matched HCs and 18 DMp with 18 age- and sex-matched HCs are shown in Table 1. None of the participants in the DM group were diagnosed with DPN. The mean duration of the diagnosis of DPN was 8.4 years in the DMp group. Seven of 18 DMp patients (CSI-A score: 34.1 ± 15.7) scored a CSI-A equal to or higher than 40 and, therefore, seemed to suffer from central sensitization syndrome. All DMp patients experienced chronic pain (NRS score: 3.7 ± 2.0), and five took pain-relieving medication on the measurement day.
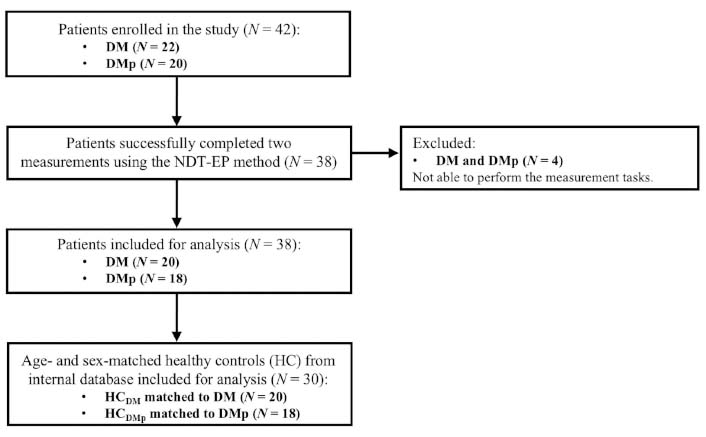
Study recruitment and inclusion procedure for the patient (DM and DMp) and their age- and sex-matched healthy control (HCDM and HCDMp) groups. DM: patients with diabetes mellitus without pain or other clinical symptoms of polyneuropathy; DMp: patients with diabetes mellitus diagnosed with chronic painful peripheral polyneuropathy
Means and standard deviations of subject characteristics, patient-reported outcome measures, and clinical features (i.e., duration of the diagnosis of diabetes mellitus (DM) and diabetic polyneuropathy (DPN), and medication intake)
Characteristics | HCDM(N = 20) | DM(N = 20) | P value | HCDMp(N = 18) | DMp (N = 18) | P value |
---|---|---|---|---|---|---|
Age (years) | 53.1 ± 16.6 | 53.4 ± 16.7 | 0.956 | 58.2 ± 10.7 | 64.6 ± 8.2 | 0.059 |
Sex (M/F) | 9/11 | 9/11 | - | 15/3 | 15/3 | - |
BMI (kg/m2) | 24.2 ± 3.2 | 26.7 ± 4.0 | < 0.05 | 25.4 ± 2.9 | 30.3 ± 4.7 | < 0.001 |
CSI-A score | 15.2 ± 8.0 | 17.6 ± 8.1 | 0.360 | 15.3 ± 8.2 | 34.1 ± 15.7 | < 0.001 |
NRS score | 0.0 ± 0.0 | 0.0 ± 0.0 | - | 0.0 ± 0.0 | 3.7 ± 2.0 | < 0.001 |
Duration DM (years) | - | 17.2 ± 10.2 | - | - | 19.1 ± 10.7 | - |
Duration DPN (years) | - | - | - | - | 8.4 ± 5.4 | - |
Medication intake (N) | 0 | 0 | - | 0 | 5 | - |
Age, sex, body mass index (BMI), central sensitization inventory-part A (CSI-A) score, and numeric rating scale (NRS) score were tested between patient (DM and DMp) and their age- and sex-matched healthy control (HC) groups using a t-test. DM: patients with diabetes mellitus without pain or other clinical symptoms of polyneuropathy; DMp: patients with diabetes mellitus diagnosed with chronic painful peripheral polyneuropathy
Detection rates and response times
The detection rate was monitored to assess whether the stimulus detection task execution of the NDT-EP method in patients with diabetes mellitus and painful DPN was equal to HCs (Figure 2). The detection rate in the DM group ranged from 0.35 to 0.50, similar to the HC group’s range of 0.36 to 0.56. However, the detection rate in the DMp group was significantly (P < 0.01) lower, ranging from 0.08 to 0.51, compared to the HC group’s range of 0.24 to 0.49. Low detection rates led to increasing stimulus strengths in the psychophysical procedure.
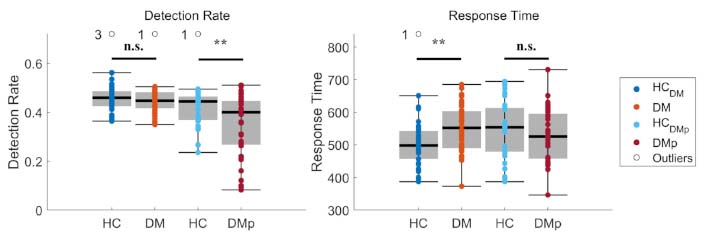
The mean detection rate and mean response time were calculated for each measurement. The effect of diagnosis on the detection rate and response time was tested between patient (DM and DMp) and their age- and sex-matched healthy control (HCDM and HCDMp) groups using linear regression (see Equation 3). Significance levels were indicated as * P < 0.05, ** P < 0.01, *** P < 0.001, and n.s. (not significant). DM: patients with diabetes mellitus without pain or other clinical symptoms of polyneuropathy; DMp: patients with diabetes mellitus diagnosed with chronic painful peripheral polyneuropathy
The response time was screened to evaluate whether Aδ-fibers were stimulated (Figure 2). The response time in DM (range 373–685 ms) was significantly (P < 0.01) higher than in HC (range 387–650 ms). In contrast, the response time in DMp was similar to that of HC.
NDTs and psychometric slopes
Mean psychophysical NDTs and psychometric slopes were calculated for each measurement in response to the stimulus types to evaluate the function of the nociceptive system (Figure 3). NDTs were observed below 2.0 mA for each stimulus type in DM, which was equal to NDTs in HC. Psychometric slopes in DM were not different compared to those in HC. In DMp, NDTs were significantly higher and slopes lower than HC.
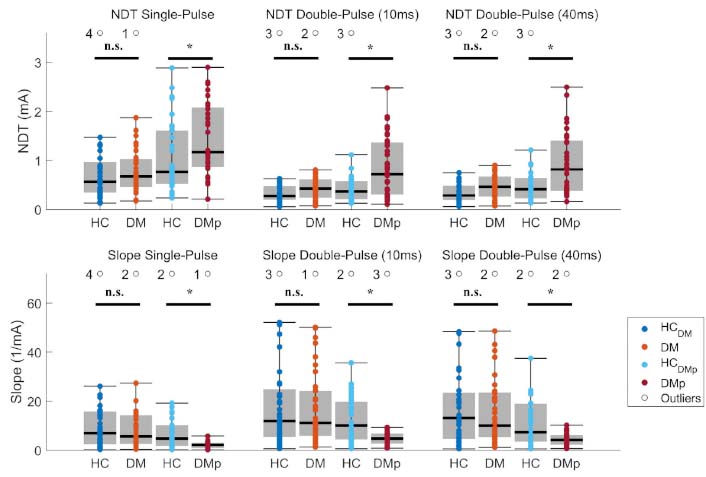
Mean nociceptive detection thresholds (NDTs) and psychometric slopes of each measurement were calculated using a generalized linear regression model (see Equation 1). The effect of diagnosis on NDTs and psychometric slopes was tested between patient (DM and DMp) and their age- and sex-matched healthy control (HCDM and HCDMp) groups using linear regression (see Equation 3). Significance levels were indicated as *P < 0.05, **P < 0.01, ***P < 0.001, and n.s. (not significant). DM: patients with diabetes mellitus without pain or other clinical symptoms of polyneuropathy; DMp: patients with diabetes mellitus diagnosed with chronic painful peripheral polyneuropathy
Evoked potentials
The grand average EPs and individual mean P2 and N1 amplitudes are illustrated for the CPz-A1A2 (Figure 4) and T7-F4 derivations (Figure 5). Grand average EPs show the neural response for detected and non-detected stimuli applied near the detection threshold (Figures 4A, 4B, 5A, and 5B). Detected stimuli elicited clear P2 and N1 amplitudes, whereas non-detected stimuli did not. Individual mean P2 and N1 amplitudes were significantly lower in the DM and DMp groups compared to the HC groups (Figures 4C and 5C). These mean P2 and N1 amplitudes are associated with stimulus detection (Figures 4E and 5E) and the number of detected stimuli (i.e., detection rate) (Figure 2).
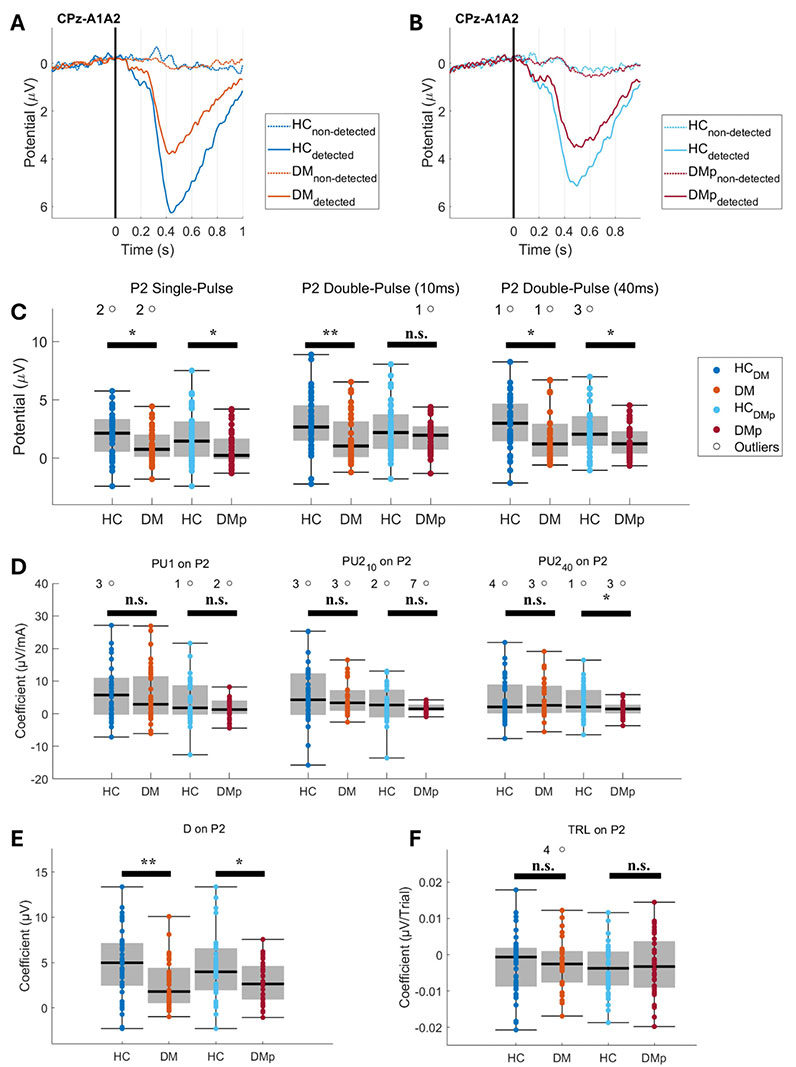
Grand average evoked potentials (EP) at the CPz-A1A2 derivation, their individual mean P2 amplitudes, and the effect of model coefficients on the P2. Grand average EPs were calculated for detected and non-detected stimuli from DM (panel A), DMp (panel B), and their age- and sex-matched healthy control (HCDM and HCDMp) groups. The mean P2 amplitude was calculated per stimulus type for each measurement (panel C). The effect coefficient of the stimulus detection (D) (panel E), the amplitude of the pulse types (PU1, PU210, and PU240) (panel D), and the trial number (TRL) (panel F) on the P2 were estimated using linear regression (see Equation 2). Individual NDT-EP outcomes for each patient group (DM and DMp) were statistically compared with the healthy control group (HCDM and HCDMp). The effect of the diagnosis on the NDT-EP outcome was evaluated using linear regression (see Equation 3). Significance levels were indicated as * P < 0.05, ** P < 0.01, *** P < 0.001, and n.s. (not significant). DM: patients with diabetes mellitus without pain or other clinical symptoms of polyneuropathy; DMp: patients with diabetes mellitus diagnosed with chronic painful peripheral polyneuropathy
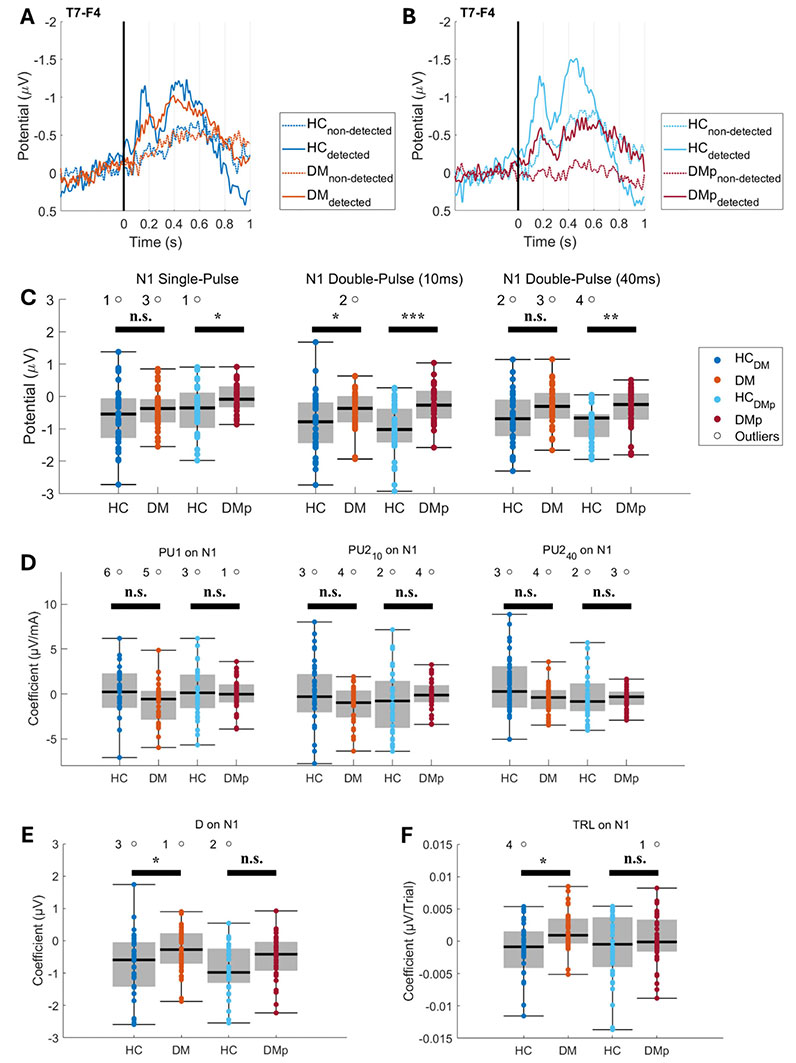
Grand average evoked potentials (EP) at the T7-F4 derivation, their individual mean N1 amplitudes, and the effect of model coefficients on the N1. Grand average EPs were calculated for detected and non-detected stimuli from DM (panel A), DMp (panel B), and their age- and sex-matched healthy control (HCDM and HCDMp) groups. The mean N1 amplitude was calculated per stimulus type for each measurement (panel C). The effect coefficient of the stimulus detection (D) (panel E), the amplitude of the pulse types (PU1, PU210, and PU240) (panel D), and the trial number (TRL) (panel F) on the N1 were estimated using linear regression (see Equation 2). Individual nociceptive detection thresholds-EP (NDT-EP) outcomes for each patient group (DM and DMp) were statistically compared with the healthy control group (HCDM and HCDMp). The effect of the diagnosis on the NDT-EP outcome was evaluated using linear regression (see Equation 3). Significance levels were indicated as *P < 0.05, **P < 0.01, ***P < 0.001, and n.s. (not significant). DM: patients with diabetes mellitus without pain or other clinical symptoms of polyneuropathy; DMp: patients with diabetes mellitus diagnosed with chronic painful peripheral polyneuropathy
Effects of the model coefficients on the P2 and N1 amplitudes are presented in Figures 4 and 5. The patients had significantly smaller effect sizes of stimulus detection on the P2 and N1 amplitudes (Figures 4E and 5E), indicating that EP amplitudes were lower for detected stimuli in patients than in HC. Furthermore, the effect of stimulus types on the P2 and N1 amplitudes was not different in patients compared to the HCs (Figures 4D and 5D) when corrected for stimulus detection (Figures 4E and 5E) and TRL (Figures 4F and 5F).
Discussion
This study provided insights into NDT-EP phenomena in diabetic patients with and without chronic painful peripheral polyneuropathy by exploring measures that potentially describe the functional integrity of the nociceptive system. Altered NDT-EP outcomes are found in diabetic patients with neuropathic pain compared to age- and sex-matched HCs.
The stimulus detection task execution of the psychophysical procedure was different in the DMp compared to the HC group, revealed by its significantly lower detection rate (Figure 2). The lower detection rate indicates that patients in this group responded less accurately to nociceptive stimuli, manifesting habituation of stimulus strengths in the procedure. Low detection rates in patients do not directly indicate increased habituation since it can also illustrate poor task execution. While the underlying reason for the impaired detection task execution remains unclear, it may be caused by either neural dysfunction or behavioral limitations (e.g., loss of attention). Feldman et al. [40] showed a loss of sensory function due to damaged small nerve fibers. Epidermal denervation in diabetes causes changes in IENF density with parallel psychophysical changes [41].
Moreover, we observed higher NDTs and lower psychometric slopes in DMp compared with HC (Figure 3). The findings emphasized that these patients were less sensitive to nociceptive stimulation and had less reliable stimulus detections. In contrast, no significant psychophysical differences were found in DM patients without clinical symptoms compared with HC (Figure 3). The preliminary results’ altered NDTs and psychometric slopes disappeared after matching patients based on age and sex [36]. These findings corroborate the suspicion about the confounding effects of age and sex in the preliminary analysis [36]. The results in DM may indicate that loss of sensory function was less in most of these patients than in patients with painful DPN [42]. Although higher NDTs and lower psychometric slopes in DMp may be caused by small fiber dysfunction, it can also be related to features of chronic neuropathic pain, including increased spontaneous neural activity in injured afferent neurons and altered central nociceptive function [43–45]. The lower psychometric slopes in DMp emphasize unreliable responses to nociceptive stimuli, of which altered habituation is one of the indicators [46].
The neurophysiological responses to nociceptive stimuli demonstrated lower mean P2 and N1 amplitudes in both patient groups compared to HC groups (Figures 4 and 5). The mean P2 and N1 amplitudes in our near-threshold stimulation procedure are affected by the task execution (i.e., the number of detected stimuli) because EP amplitudes in response to detected stimuli are higher than in response to non-detected stimuli (Figures 4 and 5) [17]. To our knowledge, this is the first study exploring near-threshold intra-epidermal electrical EPs in patients with diabetes. Since stimulus strength modulates the EP amplitude [16], it is not justified to compare these near-threshold EPs to lower supra-threshold (pain-related) EP amplitudes in patients with neuropathic pain [47–51].
Our linear regression models disclosed that the effect of pulse strengths on the P2 and N1 amplitudes was generally not different between the patient groups and HC (Figures 4D and 5D). These results imply that the differences in grand average EPs are not strongly associated with an altered coding of the pulse strength on the P2 and N1 in patients in response to near-detection threshold stimulation because the pulse amplitudes were corrected for stimulus detection and TLR in the linear regression model (Equation 2). Altered grand average EP amplitudes are more likely related to other factors (e.g., attention, arousal, or emotions) [50, 51]. Furthermore, the effect size of stimulus detection on the N1 and P2 amplitudes was significantly smaller in both patient groups than in HC (Figures 4E and 5E), implying a lower N1 and P2 amplitude when a stimulus is recognized by patients.
Our study had a few limitations to consider when interpreting the results. First, the study included 38 diabetic patients, a relatively small sample size that may limit the generalizability of the findings and the statistical significance of the results. Still, the outcomes of this explorative study could aid in determining the sample size for future studies. Second, the potential influence of behavioral factors, such as attention and arousal, on task execution and EP outcomes is not profoundly explored or controlled. This could be a limitation, particularly of the NDT-EP method given the duration of the psychophysical procedure. Shortening the procedure reduces potential loss of attention and influence of arousal levels on the NDTs and EPs. In addition, it minimizes the potential co-activation of tactile fibers. Given the higher stimulus strengths applied to diabetic patients, it could not be ruled out that Aβ-fibers were co-activated. Although the stimulus strengths that trigger Aδ-fibers in patients are unknown, Aδ-fibers are thought to be recruited at stimulus strengths up to twice the initial detection threshold in healthy subjects [19, 51]. Nevertheless, the response times (Figure 2) and N1- and P2-latencies (Figures 4 and 5) fell within the range typical for Aδ-fiber stimulation [23, 52], suggesting that nociceptive fibers were likely recruited.
Further research on NDT-EP outcomes in patients with painful DPN is recommended for improved observation of nociceptive dysfunction and eventually to detect early-stage small fiber neuropathy. Future studies may validate how malfunctioning neural mechanisms are associated with altered NDT-EP outcomes by probing the effect of experimental interventions (e.g., lidocaine) on NDT-EP outcomes in healthy subjects. A combination of QST and IENF examination is proposed to ensure selective recruitment of nociceptive fibers and evaluate small fiber dysfunction. We also suggest studying the task execution of the NDT-EP method in other syndromes with small fiber neuropathies, such as chemotherapy-induced polyneuropathy and sarcoidosis. Furthermore, future studies using the NDT-EP method might focus on sensory characteristics and small fiber function during a follow-up in patients diagnosed with painful DPN and compare outcomes in responders and non-responders to spinal cord stimulation.
In conclusion, we explored intra-epidermal electric NDT and EP outcomes in diabetic patients with and without neuropathic pain. Our findings showed significantly higher NDTs and lower psychometric slopes in diabetic patients with neuropathic pain compared with age- and sex-matched HCs. In addition, we observed lower mean EP amplitudes in both patient groups, which are not associated with an altered coding of the stimulus strength. These variations in EP amplitudes are influenced by stimulus detection and impaired task execution of the procedure. Future studies should establish whether the altered NDT-EP outcomes quantified small fiber dysfunction in patients with diabetes mellitus. Moreover, further research on the responsiveness to nociceptive stimuli in other patient groups is warranted.
Abbreviations
CSI-A: | central sensitization inventory-part A |
DM: | patients with diabetes mellitus without pain or other clinical symptoms of polyneuropathy |
DMp: | patients with diabetes mellitus diagnosed with chronic painful peripheral polyneuropathy |
DPN: | diabetic polyneuropathy |
TRL: | trial number |
EP: | evoked potential |
HC: | healthy control |
IENF: | intra-epidermal nerve fiber |
IES: | intra-epidermal electrical stimulation |
IPI: | inter-pulse interval |
NDT: | nociceptive detection threshold |
NRS: | numeric rating scale |
QST: | quantitative sensory testing |
EEG: | electroencephalography |
Declarations
Author contributions
TB: Conceptualization, Data curation, Formal analysis, Investigation, Methodology, Project administration, Resources, Validation, Visualization, Writing—original draft, Writing—review & editing. IPK: Conceptualization, Investigation, Methodology, Project administration, Resources, Writing—original draft, Writing—review & editing. TB and IPK contributed equally to this work. BvdB: Formal analysis, Methodology, Software, Validation, Writing—review & editing. SRG: Investigation, Project administration, Resources, Writing—review & editing. JRB: Conceptualization, Funding acquisition, Methodology, Supervision, Writing—review & editing. All authors read and approved the submitted version.
Conflicts of interest
The authors declare that they have no conflicts of interest.
Ethical approval
All experiments were approved by the Medical Research Ethics Committees United (MEC-U, file number: NL66136.100.18) and in accordance with the 1964 Helsinki Declaration and its later amendments.
Consent to participate
All participants provided written informed consent and were rewarded for participation in the experiment.
Consent to publication
Not applicable.
Availability of data and materials
A limited dataset of the experiments reported here is available on request.
Funding
This project was funded by the TKI High Tech Systems and Materials (HTSM) via the Dutch Ministry of Economic Affairs and Climate Policy’s PPS allowance scheme for Research and Innovation. The funders had no role in study design, data collection and analysis, decision to publish, or preparation of the manuscript.
Copyright
© The Author(s) 2024.