Abstract
Melatonin is widely available as a dietary supplement and/or medicine for sleep. It is an endogenous hormone produced in the pineal gland of the brain, with metabolites providing additional beneficial mechanisms such as supporting long-term memory. Melatonin is well known as a hormone that plays a role in the circadian rhythm (sleep cycle), but additional mechanisms such as antioxidant, and anti-inflammatory activity are elucidated from animal research models. This article discusses melatonin supplementation and the current understanding of how it may provide benefits beyond the use as a sleep aid including a review of the evidence in how it may aid in mitigating components of cognitive decline.
Keywords
Melatonin, memory, sleep, neurodegeneration, dementia, antioxidant, anti-inflammatoryIn humans, melatonin is a hormone predominantly produced by the pineal gland in the brain [1, 2]. Melatonin is also, to a lesser extent, produced in the bone marrow, the gastrointestinal tract, the retina, lymphocytes, and thymus [1]. Melatonin is involved with numerous physiological roles including sleep circadian rhythm, neuronal and immune functions, and pubertal development [2–4]. In addition to the physiologic functions listed, melatonin is gaining evidence regarding neuroprotective properties specific to antioxidant and anti-inflammatory mechanisms occurring in the central nervous system (CNS) [1, 5].
Melatonin is sold throughout the world either as a supplement or medication (depending on the country) [3]. As individuals age, a decrease occurs in the production of endogenous melatonin [6, 7]. This decrease in the production of endogenous melatonin may be a reduction in exposure to a neuroprotective molecule. Humans are living longer, and the living do not wish to suffer through the side effects evidenced with older age such as decreased cognition, decreased sleep, decreased muscle mass, decreased energy, etc. Can exogenous melatonin play a role in improving some of the decline associated with aging?
Sleep
It has been hypothesized that sleep is the time in which the brain is best able to remove metabolic waste [8]. As a clinician, this brings to mind the immediate question: What type of sleep? Does drug-induced sleep impact the quantity of ‘waste’ removal? These questions are important as many individuals utilize medications to support sleep, but is there a difference in quality based on what is used to support sleep? Will melatonin promote sleep that supports beneficial mechanisms suggested to occur with sleep?
Currently, many questions remain regarding the role sleep provides in the removal of toxins or metabolic waste from the brain (or CNS). Fascinating studies evaluating the activity of the brain in mice while sleeping and awake have led scientists to identify coordinated neuronal activity that was exhibited while under ketamine anesthesia [8]. The authors highlight that neurons act as effective pumps as evidenced by their powering of sodium/potassium ATPases [8]. Furthermore, the coordinated neuronal activity exhibited while under anesthesia was not exhibited when mice were awake [8]. In addition to the exhibition of significant coordinated neuronal activity, changes in interstitial and cerebral spinal fluid dynamics led authors to suggest that the coordinated brain activity exhibited during sleep may exhibit the time in which the most efficient clearance of brain waste (metabolic byproducts) occurs. However, another study identified evidence supporting the most significant times of metabolic waste clearance occurred during times in which the mice were awake [9]. These studies pose new questions such as: Does the quality of sleep impact the level of clearance? Even if less (clearance) occurs while sleeping (than awake), what enables the highest level of removal while sleeping?
The blood-brain barrier protects the brain from being exposed to much of the chemicals consumed or present in the blood. However, since it blocks chemicals from getting into the brain, it also blocks them from getting out [10]. As previously noted, it is not fully understood how clearance of metabolic waste occurs in the brain. Jiang-Xie et al. [8] determined that during sleep a coordinated effort of neuronal activity likely results in large coordinated neuronal activity that supports the removal of metabolic waste. Miao et al. [9] countered that when evaluating fluorescent molecules in the brains of mice, the clearance occurring during sleep or anesthesia-induced sleep was less than that exhibited when the mice were awake. Interestingly, Miao et al. [9] found that the reduction of clearance from the brain (in comparison to times in which mice were awake) was 50% during anesthesia and 30% when sleeping without anesthesia. Does this provide evidence that drug-induced sleep negatively impacts clearance? Future studies are needed to determine the average daily clearance of metabolic brain waste to evaluate confounders such as changes in sleep and changes in activity levels while awake to identify what equates to the best overall ‘metabolic waste clearance’.
The clearance of metabolic waste is suggested to be one hypothetical model for the development of Alzheimer’s disease (AD) due to the evidence of buildup of aggregate proteins, such as the toxic proteins β-amyloid (Aβ) and neurofibrillary tangles (referred to as tau) [1]. Sadly, eliminating these toxic proteins as provided with donanemab, an amyloid-beta directed antibody, exhibited a decrease in the proteins without exhibiting significant improvement in symptoms except in APOE ε4 carriers [11]. Further clinical trials determined significant improvement in decreasing cognitive decline, however with significant risks associated with treatment including cerebral edema (24% treatment vs 2% placebo), and intracranial bleeding (31% treatment vs 13% placebo) [12, 13]. Should the mechanism of improved clearance of metabolic waste be the targeted mechanism, or do other mechanisms play a larger role in the development of AD?
Melatonin vs other sleep medications
Melatonin is a hormone that is produced endogenously to support several mechanisms in the body. It orchestrates the circadian sleep cycle by promoting sleep and inhibiting wake signals via activation of melatonin 1 receptor and melatonin 2 receptor (MT1/MT2) [1, 14]. Endogenous melatonin declines as individuals age and this may play a role in the decreased sleep (and sleep quality) that occurs in the aging population [6, 7]. Most medications utilized to aid with sleep are associated with cognitive decline [7, 15, 16]. Medications that are commonly used for sleep and associated with cognitive decline and/or dementia include chronic use of the following: alcohol, first-generation antihistamines (chlorpheniramine, diphenhydramine, dimenhydrinate, hydroxyzine), tricyclic antidepressants (amitriptyline, desipramine), and anti-psychotics (olanzapine, quetiapine) [17–19]. This list is far from complete but provides a good depiction of some of the most common medications currently understood to be associated with cognitive decline, while also inducing sleep. Importantly, all these medications (except for alcohol) are on this list because of their “cholinergic burden” meaning that drugs with high levels of anticholinergic activity are associated with cognitive decline, and the more anticholinergic activity exhibited by a medication, the more cholinergic burden. Additionally, taking more than 1 medication with anticholinergic activity augments association with cognitive decline [7, 15, 18, 19]. In contrast, exogenous melatonin does not exhibit cholinergic burden, is not associated with cognitive decline, and has evidence supporting improved sleep efficacy [7, 20, 21].
Melatonin promotes sleep, but the cognitive benefits seen with melatonin are supported by mechanisms beyond improving the quality of sleep [5, 22, 23].
What roles (other than sleep) may melatonin offer to support cognitive health?
Many studies performed in rodents have identified neuro-beneficial mechanisms occurring with melatonin supplementation [5, 6, 22–25]. Additionally, the metabolites of melatonin, N1-acetyl-N2-formyl-5-methoxykynuramine (AFMK), and N1-acetyl-5-methoxykynuramine (AMK) also exhibit evidence supporting cognitive benefit [6, 22].
See Figure 1 for the depiction of melatonin and corresponding metabolites.
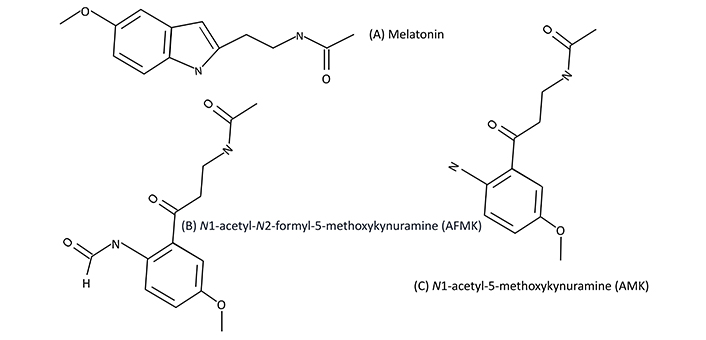
Melatonin, AFMK, and AMK. Melatonin (A) and corresponding melatonin metabolites, AFMK (B), and AMK (C), respectively
Cognitive decline and neurodegenerative diseases may be, in part, due to declining endogenous levels of melatonin [23, 26]. Additionally, like melatonin, endogenous levels of AMK decline with age, thus leading to a decrease in neuroprotective activity as individuals age [6, 25].
Studies evaluating melatonin for mechanisms involved with cognition/memory have identified signaling pathways involved with memory formation. Proteins that are considered “memory related” include extracellular signal-regulated kinase (ERK), calcium/calmodulin-dependent kinases (CaMKs), and cAMP-response element binding protein (CREB) [6, 25]. Long-term memory requires phosphorylation of CREB [6, 22].
Iwashita et al. [27] identified that object memory was disrupted in mice when the metabolism of melatonin to the AMK metabolite was inhibited. Additionally, AMK supplementation was shown to improve age-related memory decline [27]. Sano et al. [25] investigated melatonin, AMK, ramelteon (an MT1/MT2 agonist), and a placebo vehicle on memory formation and phosphorylation of memory-related proteins in male mice. AMK, melatonin, and ramelteon all resulted in the phosphorylation of memory-related proteins at doses of 1 mg/kg, specifically increases in the phosphorylation of CREB and ERK in the hippocampus [25]. The placebo vehicle did not lead to any activity that exhibited memory development, nor did phosphorylation occur with memory-related proteins [25].
Figure 2 provides a depiction of ramelteon, melatonin, and melatonin’s corresponding metabolites. Importantly, melatonin neuroprotective benefit is not solely associated with MT1/MT2 agonist activity.
Bocheva et al. [23] provide an eloquent review of the numerous mechanisms in which melatonin exhibits evidence of providing neuroprotective mechanisms, including prevention of inflammatory processes specific to neurogenerative diseases, antioxidant activity, and specific pathways in which melatonin has exhibited protective effects such as with the inhibition of the NLRP3 inflammasome, a protein complex that initiates cell death and subsequent cytokine release.
Why isn’t every older adult using melatonin as a sleep aid and cognitive protectant?
An important caveat to consider is that most studies evaluating melatonin for cognitive or neuroprotective benefit do so utilizing animal models and at doses significantly exceeding what is currently approved for use in humans. Zakaria et al. [24] reviewed 24 rodent studies evaluating the effect of melatonin supplementation on memory and learning. Of the 24 studies analyzed, only 2 of the studies included melatonin doses that are known to be safe in humans. Rudnitskaya et al. [28] performed a study utilizing an AD model at a dose of 0.04 mg/kg per day. This dose of melatonin exhibited diminished progression of amyloid-beta levels, and decreased neuronal cell death in animals at doses that would equate to what is considered safe in humans [25]. Other AD animal model studies utilized doses from 0.5 mg/kg per day to 100 mg/kg per day [24]. For a 160-pound person that would be a range of melatonin from 36 mg to 7,373 mg daily. In humans, melatonin is recommended between 0.1 mg to 10 mg [14]. A trial evaluating melatonin for sleep in older adults with doses of 0.3 mg and 5 mg, found that the “high dose” melatonin (5 mg) exhibited significant improvement in sleep efficacy [20]. The evidence supporting the use of melatonin for cognitive benefit is strong in rodents, but weak in humans. Additional human studies are needed. Studies should evaluate what doses of melatonin are needed (in humans) to provide neuroprotective benefits rather than simply improvement in sleep measures. When performing a scan on PubMed for clinical trials utilizing melatonin (in humans) that included (cognition) as a search term, the majority of studies evaluate melatonin for indications that stray from evaluating the impact of melatonin on memory or cognitive improvement. There are numerous human studies evaluating melatonin for use in the prevention of delirium for various types of surgeries, the use as a sleep aid in children with concussions, in the setting of late-stage breast cancer, and in patients of post-chemotherapy. One study evaluated melatonin in those with Parkinson’s disease, which resulted in significant improvement in subjective sleep scores, but other measures did not attain significance [29]. This may be a result of the small sample size of 18 participants [29]. Of the 104 studies resulting from a PubMed search with the selection criteria described above, only two studies truly engage with the hypothesis that melatonin may provide improvement in cognitive health in humans with exogenous melatonin administration. Xu et al. [30] evaluated the lamina cribrosa thickness (LCT) and hippocampus volume of individuals with mild cognitive impairment. This placebo-controlled trial resulted in individuals in the melatonin group (0.15 mg/kg of melatonin for 6 months), exhibiting significantly larger LCT and larger hippocampus volume than those in the placebo group (p < 0.001) [27]. This is important because thinner LCT and smaller hippocampus volume are associated with a lower Mini Mental State Examination (MMSE) score. MMSE is a validated tool used to assess cognitive impairment in older adults. This study identified objective measures of change following a rather large melatonin dose (for humans), which is worthy of appreciation considering the significant pre-clinical studies that support higher dosing of melatonin for the indication of improvement in cognitive outcomes. Wade et al. [21] evaluated a 2 mg extended-release dose of melatonin in individuals with mild to moderate AD. The findings indicated statistically significant improvement in MMSE, instrumental activities of daily living, and sleep efficiency [21]. Additionally, the side effects reported were similar to placebo [21]. Studies evaluating the utilization of oral melatonin (at doses between 1–10 mg) indicate that melatonin is well tolerated, and side effects are minimal. Dizziness and headaches have been reported, but certainly less concerning than the side effects listed with other commonly used sleep medications (such as risk of falls and dementia).
Importantly, additional clinical trials in humans that evaluate mechanisms indicative of improvement in cognitive health are needed, and monitoring for safety beyond passive reporting of side effects is necessary. Any medication that should aid with sleep should include heart rate (HR), and pulse oximetry monitoring, at a minimum. In the clinical trial evaluating ramelteon for efficacy in patients with chronic insomnia, participant vital signs were evaluated for 2 nights prior to continuing with the trial [31]. It is important to note that this paper discusses the use of exogenous melatonin in adults, not children. In children, melatonin overdoses have occurred, with some resulting in the need for mechanical ventilation, and even death [32]. This paper evaluates the evidence for melatonin supplementation in adults at the time in which endogenous melatonin declines, and not during stages of development.
Conclusions
There is fascinating evidence supportive of melatonin being a neuro-protective hormone, but additional studies are needed to support whether these neuroprotective mechanisms manifest into clinical benefit in adult humans when provided exogenously. There is no doubt that neuroprotective mechanisms occur with endogenous melatonin, but confirmation that exogenous melatonin can provide such mechanisms remains to be determined. Furthermore, what doses may exhibit such benefits and remain safe to consume in humans remains to be answered.
Abbreviations
AD: | Alzheimer’s disease |
AMK: | N1-acetyl-5-methoxykynuramine |
CNS: | cerebral nervous system |
CREB: | cAMP-response element binding protein |
ERK: | extracellular signal-regulated kinase |
LCT: | amina cribrosa thickness |
MMSE: | Mini Mental State Examination |
MT1/MT2: | melatonin 1 receptor and melatonin 2 receptor |
Declarations
Author contributions
LAS: Conceptualization, Data curation, Writing—review & editing.
Conflicts of interest
The author of this article does not have any conflicts of interest to disclose.
Ethical approval
Not applicable.
Consent to participate
Not applicable.
Consent to publication
Not applicable.
Availability of data and materials
Not applicable.
Funding
Not applicable.
Copyright
© The Author(s) 2024.