Abstract
Small fiber neuropathy (SFN) is a relatively common, but largely understudied neurological syndrome which has affected the lives of many globally. The common symptoms of SFN include pain, dysesthesia, and autonomic dysfunction, which are caused by damage to small nerve fibers. Due to its heterogeneous nature, SFN causes a multitude of symptoms which makes the disease and its subtypes difficult to diagnose. Furthermore, as the pathophysiology of SFN remains largely enigmatic, no cause is found in around 50% of the cases and these are classified as idiopathic SFN (iSFN). The difficult task of diagnosing SFN, and the even more elusive feat of hunting for the underlying etiology, demands accurate, precise, preferably noninvasive, and affordable tools, or a combination of them. Accordingly, appropriate biomarkers for SFN are needed to stratify patients and develop cause-centered treatments in addition to symptomatic treatments. As peripheral axons grow and repair, identifying underlying causes of SFN and intervening early may spur axonal regeneration in young patients, which can greatly improve their symptoms and improve quality of life. This narrative review aims to objectively highlight functional, histological, and molecular biomarkers to aid clinicians in discerning the diagnostic tests they should use to diagnose, confirm and determine the etiology of SFN. The strengths and limitations of each potential biomarker will be discussed. Clearer diagnostic criteria, guidelines, and work-up for SFN are required for clinicians to better identify the disease in patients presenting with non-specific symptoms.
Keywords
Small fiber neuropathy, biomarkers, antibodies, skin biopsy, intra-epidermal nerve fiber densityIntroduction
Peripheral nerve fibers can be categorized according to their size and related functions. While large nerve fibers comprise myelinated Aα, and Aα/β fibers that control motor and sensory functions, the small nerve fibers comprise the unmyelinated C fibers and the thinly-myelinated Aδ fibers that control temperature perception, pain, and autonomic functions. In patients with small fiber neuropathy (SFN), both the Aδ and C fibers are damaged. Due to the diverse functions of small fibers, the symptoms of SFN are varied, with the most common clinical presentation including neuropathic pain (NeP), burning in the extremities, and autonomic dysfunction [1]. Increasingly more is being discovered on the mechanism of NeP in SFN. Transient receptor potential vanilloid subtype 1 (TRPV1) was first described to be involved in painful neuropathies in 2006 [2] and was later localized to the keratinocytes in patients with SFN [3]. Neurotoxins depleting TRPV1 induces the upregulation of multiple biochemical compounds and receptors, thus leading to a complicated cascade of cellular signaling changes [4].
Small fibre neuropathy (SFN) is a relatively common, but largely understudied neurological syndrome which has affected the lives of many globally. Although the estimated incidence of SFN is about 52.95 per 100,000 population [5], this is likely a gross underestimation due to diagnostic challenges. Studies even propose that SFN underlies 40% of fibromyalgia syndrome [6, 7] and hence, the actual number of sufferers could be close to 100 million worldwide [8].
While autoimmune diseases, diabetes, amyloid, sarcoidosis, toxic substances, and vitamin B12 (vit B12) deficiency are common secondary causes of SFN, no cause is found in around 50% of the cases [9, 10]. iSFN treatment often targets symptomatic relief using non-opioid and opioid analgesics, antidepressants, and antiepileptic medications [11]. Not only do these methods fail to address the underlying causes of iSFN, long-term use may also create drug dependence, specific drug-dependent adverse effects, and opioid abuse. Thus, the identification of biomarkers is recommended to screen for occult causes of initially iSFN (iiSFN) cases in patients without known risks. Identifying underlying causes in iiSFN patients can allow early treatment, thus greatly improving patients’ quality of life, particularly in young patients. As peripheral axons grow throughout life, early intervention in SFN patients may spur axonal regeneration which can improve or even cure patients’ symptoms [12]. A study of patients with mixed polyneuropathies obtained evidence showing that screening led to potentially disease-modifying management changes in 25% of them [12]. Hence, screening in patients with iiSFN for its most common occult causes is recommended [10, 13].
The prevalence and the debilitating yet non-specific effects of SFN underline the need for biomarker identification to stratify patients and develop cause-centered therapies. According to the Biomarkers, EndpointS and other Tools (BEST) glossary, a biomarker is a defining characteristic that is measured as an indicator of normal biological processes, pathogenic processes, or responses to an exposure or intervention, including therapeutic interventions. Biomarkers can be further divided into their functions: susceptibility/risk, diagnostic, monitoring, prognostic, predictive, pharmacodynamic/response, and safety [14]. In this way, SFN biomarkers can identify the patient population more accurately and may be used as diagnostic tests to tailor treatment plans according to different causes of SFN.
However, the “objectivity” of SFN biomarkers is affected by the local context and age of patients. Due to the complex nature of the disease, the frequencies of different causes of SFN may vary depending on geographical location, ethnicity, genetics, and environment. A study conducted on 84 patients in the Czech Republic showed diabetes mellitus (DM) as the most frequent and powerful risk factor for SFN in that region (odds ratio = 3.6, P = 0.009) [15]. In contrast, a study from the United States found that the most common etiologies of SFN were idiopathic (73%), presumed hereditary (18%), and DM (10%) [16]. The largest cohort study to date of 921 patients with pure SFN was conducted in the Netherlands, and it found that associated conditions in order of prevalence were autoimmune diseases, sodium channel (SCN) gene mutations, DM, glucose intolerance, and vit B12 deficiencies [10]. In addition, SFN commonly affects otherwise healthy children and young adults, in whom its association is often linked to inflammation or dysimmunity [17]. Hence, this review highlights the characteristics of various biomarkers and their functions to aid clinicians in discerning the types they should employ to diagnose and confirm SFN accordingly.
SFN is a disabling condition that significantly decreases a patient’s quality of life both physically and psychologically [11]. Due to the heterogeneity of the disease, diagnosis, search for etiology, treatment choices and prognostication are challenging. Therefore, biomarkers would significantly aid in these management aspects and the treatment of the root causes of SFN.
A literature review was performed with the search terms “small fiber neuropathy” and “biomarkers” or “diagnosis” or “diagnostic tools” or “diagnostic tests”, limited to human studies on PubMed/MEDLINE, Web of Science, and Embase from 2010 to the present. This year was chosen as the cutoff as guidelines from the European Federation of Neurological Societies/Peripheral Nerve Society were published on the use of skin biopsy and normative values of intra-epidermal nerve fiber density (IENFD) for bright field microscopy in the diagnosis of SFN [18]. This is to ensure stringent criteria of biopsy-proven SFN were used. The aim was to comprehensively summarize the important biomarkers to guide clinicians in the management of SFN in patients from various age groups. Ultimately, we hope that the information in this review may help reduce time, patient distress, and costs during the screening process and determine causal treatments of SFN to improve patients’ quality of life.
Clinical assessment
Clinical presentation
Clinical history and presentation are the first steps in diagnosing SFN, both secondary and idiopathic, and center on sensory and autonomic complaints. As large nerve fibers transmit motor functions and sensory functions of light touch, proprioception, and vibration, large fiber pathology is less likely if power and tendon stretch reflexes are preserved. Additionally, a nerve conduction test is required for exclusion [11].
Due to its heterogeneous nature, SFN causes a multitude of symptoms which makes the disease and its subtypes difficult to diagnose. Typical symptoms of SFN include pain triggered by increasing temperatures [19], intolerance to bedsheets and socks accompanied by alteration of thermal sensation [20], cramps [20], restless legs syndrome [19], and pruritus [21, 22].
Small fiber pathology occurs in conditions such as motor neuron disease [23] and Parkinson’s disease [24], but these patients do not usually present with the characteristic clinical features or neuropathic distribution required for a diagnosis of SFN. In particular, SFN typically presents in 2 main clinical patterns: length-dependent SFN (LD-SFN) or non-length-dependent SFN (NLD-SFN). LD-SFN starts in the distal extremities of the legs and hands symmetrically, before ascending upwards; NLD-SFN symptoms are asymmetric, patchy, or involve the face and trunk prior to the involvement of the extremities. The proportion of NLD-SFN to LD-SFN has been approximated at 1:4 [22] to 1:3 [25], but the exact proportion is unknown, as patients with NLD-SFN tend to be under-recognized and misdiagnosed as having a psychogenic disorder [25]. In particular, NLD-SFN is more common in women, presents at a younger age, and has a greater association with immune-mediated conditions than LD-SFN [25].
Diagnostic criteria for more accurate diagnosis of diabetic SFN were decided by panel experts at the joint meeting of the 19th Annual Diabetic Neuropathy Study Group of the European Association for the Study of Diabetes (NEURODIAB) and the 8th International Symposium on Diabetic Neuropathy (Toronto, Ontario, Canada, October 2009). Graded diagnostic criteria for use in all forms of SFN were proposed, including (1) possible SFN, the presence of length-dependent symptoms, and/or clinical signs of small fiber damage; (2) probable SFN, the presence of length-dependent symptoms, clinical signs of small fiber damage, and normal sural nerve conduction study; and (3) definite SFN, the presence of length-dependent symptoms, clinical signs of small fiber damage, normal sural nerve conduction study, and low IENFD at the ankle and/or abnormal quantitative sensory testing (QST) thermal thresholds in the foot [26]. A diagnostic algorithm for patients with suspected SFN can be followed Figure 1, based on the common clinical features Table 1 to identify SFN and the blood tests and investigations Table 2 according to clinical suspicion that clinicians may refer to for greater ease in confirming the etiology of SFN.
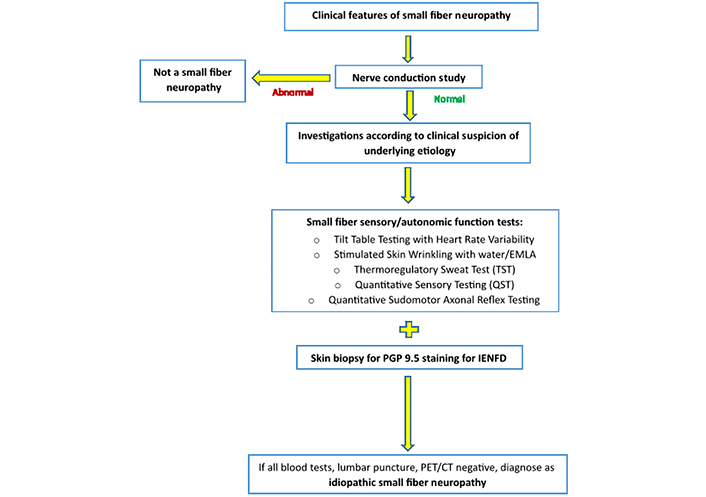
Algorithm of diagnostic work-up for patients with suspected SFN. EMLA: eutectic mixture of local anesthetics; PET: positron emission tomography; CT: computerized tomography
Clinical features of SFN in the presence of normal muscle strength, proprioception and tendon reflexes
Sensory | Autonomic |
---|---|
Positive sensory symptoms: tingling, burning, prickling, shooting pain, or aching Worse at night, interfering with sleep Allodynia Hyperesthesia Cramps Numbness, tightness Sensation of coldness Impaired thermal and pain sensitivity | Increased/decreased sweating Facial flushing Skin discolouration Dry eyes and mouth Orthostatic hypotension Urinary symptoms of hesitancy, incomplete emptying Enteric symptoms of constipation, diarrhea, abdominal distension, food intolerance Miosis and tonic pupils |
Blood tests according to clinical suspicion of underlying SFN etiologies
Etiologies associated with SFN | Diseases | Blood tests and other investigations |
---|---|---|
Metabolic | DM, IFG, IGT, hypertriglyceridemia, hypothyroidism | HbA1C, FG, OGTT, TFT |
Nutritional deficiencies | - | vit B1, vit B12 |
Infectious | HIV, HCV, HBV, Lyme disease | CRP, HIV, Borrelia burgdorferi, HBV, HCV, lumbar puncture |
Autoimmune | Vasculitis, SLE, Sjögren’s syndrome (SS), rheumatoid arthritis, connective tissue disease, celiac disease, sarcoidosis | ESR, ANA, anti-ENA, SLE panel, ANCA, anti-transglutaminase antibody, RF, serum ACE, CXR, lumbar puncture |
Paraneoplastic | Small cell lung cancer, solid tumors, lymphoma, myeloma, monoclonal gammopathies | Tumor markers, LDH, myeloma screen, SPE, UPE, anti-Hu and anti-CV2/CRMP-5 antibodies, lumbar puncture, whole body PET/CT |
Neurotoxins | Metronidazole, alcohol, chemotherapeutic agents, solvents | Urine + blood toxicology, EtOH level, GGT, MCV, screen and review drug history |
Hereditary | Fabry disease, HSAN, hATTR, Nav 1.7, Nav 1.8 and Nav 1.9 sodium channelopathies | Leukocyte alpha-galactosidase A and globotriaosylceramide (Gb3 or GL-3) levels, renal panel, urine protein, genetic testing for TTR, HSAN, SCN9A, SCN10A, SCN11A, skin/nerve/other peripheral fat pad biopsy for congo red staining |
IFG: impaired fasting glucose; IGT: impaired glucose tolerance; HbA1C: hemoglobin A1C; FG: fasting glucose; OGTT: oral glucose tolerance test; TFT: thyroid function tests; EtOH: ethanol; GGT: gamma-glutamyl transpeptidase; MCV: mean cell volume; CRP: C-reactive protein; hATTR: hereditary transthyretin amyloidosis; HIV: human immunodeficiency virus; HBV: hepatitis B virus; HCV: hepatitis C virus; SLE: systemic lupus erythematosus; ESR: erythrocyte sedimentation rate; ANA: anti-nuclear antibody; anti-ENA: ant-extractable nuclear antigens; ANCA: anti-neutrophil cytoplasmic antibody; RF: rheumatoid factor; ACE: angiotensin converting enzyme; CXR: chest X-ray; LDH: lactate dehydrogenase; SPE: serum protein electrophoresis; TTR: transthyretin, UPE: urine protein electrophoresis; HSAN: hereditary sensory autonomic neuropathy; Nav 1.7: SCN subunit 1.7; CV2/CRMP-5: CV2/collapsing response mediator protein 5; Gb3 or GL-3: globotriaosylceramide; -: information not available
In patients with LD-SFN, the most common presentation is burning pain in the feet. This NeP in the feet is a manifestation of the spontaneous activity of damaged sensory C-fibers that transmit unprovoked pain signals centrally while releasing vasoactive substance P and calcitonin gene-related peptide distally to cause neurogenic inflammation [27]. However, the condition can also be pain-free, with absent or reduced pain and temperature sensation [28]. Clinically, patients with LD-SFN may present with additional symptoms including chronic fatigue and reduced exertional tolerance, which are caused by neuropathic micro-vasculopathy, as skeletal muscle shunts arterial blood directly into venules, bypassing capillaries, depriving exercising myocytes and triggering local hypoxemia [29, 30]. In addition, impaired venous contractility in the legs reduces cardiac return and thus cardiac output, which worsens perfusion throughout the body. Among 229 patients with chronic fatigue attributed to preload failure, 31% had skin biopsies consistent with SFN [31]. This pathophysiologic profile, which records low perivascular unmyelinated axon-Schwann (UAS) ratios, reportedly identifies SFN with 90% sensitivity and 91% specificity [30]. Furthermore, gastrointestinal symptoms such as postprandial nausea, vomiting, and lower dysmotility symptoms may be due to neuropathic dysregulation of enteric vasculature (gastrointestinal angina), along with enteric small fiber loss [32]. It is possible that neuropathic dysregulation also contributes to brain symptoms of SFN, such as chronic daily headache and cognitive dysfunction [33–35]. Future studies are needed to confirm the intersystem of SFN symptoms.
LD-SFN symptoms may be present in patients with iSFN [36] as well as those with impaired glucose intolerance or diabetes, which are common secondary causes of SFN [37]. However, symptoms remain limited to the feet in most patients with pure SFN [19]. Although neuropathic symptoms typically occur late in patients with type 2 DM, SFN may also occur early and predate large fiber neuropathy [38]. This observation supports the hypothesis that pure SFN precedes large fiber dysfunction in diabetic neuropathy and further develops into mixed neuropathies involving both small and large fibers.
Conversely, 25% of SFN patients do not present with classical symmetric stocking-and-glove presentations [22] and instead, with patchy or proximal distributions of NeP. As opposed to axonopathy in LD-SFN, NLD-SFN is caused by selective ganglionitis or neuronitis involving dorsal root ganglion cells that aid the function of small nerve fibers, with metabolic or immune processes detected in half of these patients [39]. Cerebrospinal fluid can reveal inflammatory markers and mononuclear infiltrates, and nodules of Nageotte are pathological hallmarks of cell-body degeneration [40]. As large fiber cell bodies are often also attacked, patients can experience ataxia or reduced proprioception, hyporeflexia and abnormal nerve conduction study results, or somato-sensory-evoked potentials. Since nerve conduction studies may be unrevealing early in the disease course, magnetic resonance neurography (MRN) may be useful in showing the appearance of dorsal column lesions on spinal cord MRI to help diagnose SFN early [41], which may reflect neuronal death and Wallerian degeneration from the loss of cell bodies within the dorsal root ganglion [42].
Possible causes of chronic NLD-SFN symptoms encompass SS and proximal diabetic radiculopathy [43, 44]. On one hand, restricted ganglionitis can present without serologic markers, including in 60% of patients with SS-associated SFN [17]. Suspected cases can be confirmed with ophthalmologic evaluation and lip biopsy of salivary glands. On the other hand, paraneoplastic painful ganglionitis is most often associated with anti-Hu amphiphysin and anti-CV2 autoantibodies, for which the lung is the most common site of a malignant condition, followed by hematological and gastrointestinal tumors [45]. As there is a narrow therapeutic window to intervene in NLD-SFN before there are irreversible deficits, NLD-SFN requires prompt recognition for early disease-targeted intervention [46].
Questionnaires and scales
In addition to the clinical presentation to identify SFN and its possible causes, the use of validated questionnaires and scales is recommended to determine the severity and intensity of symptoms for diagnosis and evaluation of treatment response [9]. There are many questionnaires that individually assess for NeP and dysautonomia. The Leeds Assessment of Neuropathic Symptoms and Signs, the Neuropathic Pain Questionnaire the Douleur Neuropathique en 4 Questions (DN4), PainDETECT, and Identification Pain (ID-P), based on verbal pain descriptors have been validated as screening questionnaires for NeP [47], while the Scales for Outcomes in Parkinson’s Disease Autonomic Questionnaire (SCOPA-AUT) [48], the Survey of Autonomic Symptoms (SAS) [49], and the composite autonomic symptoms scale (COMPASS) [50] have been validated to assess autonomic dysfunction in various medical conditions. For this review, we will focus on the SFN-specific and SFN-validated questionnaires: SFN Symptoms Inventory Questionnaire (SFN-SIQ), SFN-Specific Rasch-built Overall Disability Scale (SFN-RODS), SFN Screening List (SFN-SL), and COMPASS-31 [51].
The SFN-SIQ determines the severity of SFN symptoms. It consists of 13 questions to assess changes in various aspects of sensory or autonomic dysfunction: palpitations, flushes, constipation or diarrhea, urination problems (incontinence or hesitancy), changes in sweating pattern, restless legs, orthostatic dizziness, dry eyes or mouth, oversensitivity and sheet intolerance of the legs via a 4-point Likert scale [52]. The SFN-SIQ is the only validated SFN-specific tool to assess symptoms and has been shown to have a sensitivity of 86% and specificity of 70% in the diagnosis of pure SFN compared to IENFD as the diagnostic standard [53].
Adapted from SFN-SIQ, the SFN-RODS is a 32-item questionnaire that uses an activity/participation scale at a disease-specific interval level to assess the disability in SFN patients. Specific to SFN symptoms, interval measures at the impairment level address symptoms, and daily and social restrictions at the activity/participation level [52].
In patients with sarcoidosis, the SFN-SL is often used to detect SFN. Consisting of two parts of 8–13 questions, SFN-SL assesses the incidence of symptoms and their respective intensities based on a rating from 0 point to 4 points. A moderate probability for SFN is indicated by a total score exceeding 22 points whereas a very high probability would have a score exceeding 48 points. The main limitation of the SFN-SL questionnaire is that it was developed to diagnose SFN due to sarcoidosis, and a large proportion of scores were in the intermediate range [54, 55].
Amongst standard neuropathy scales, the Utah Early Neuropathy Scale (UENS) is the most specific to SFN diagnosis. The UENS is the only scale that focuses on the severity and spatial distribution of pin (sharp) sensation loss in the foot and leg, with less emphasis on motor weakness [56]. The UENS was designed specifically to detect early SFN in diabetic or pre-diabetic patients. It has a high sensitivity of 92% without sacrificing specificity and an inter-rater reliability of 94% [36, 56].
The COMPASS-31 was developed from the autonomic symptom profile questionnaire that encompasses 31 questions measuring autonomic dysfunction alone—cardiovagal, adrenergic and sudomotor functions. COMPASS-31 scores were found to be different between subjects with and without SFN, with fair diagnostic accuracy. The optimal cutoff derived from the receiver operating characteristic (ROC) curve is 30, yielding a sensitivity of 0.741 and a specificity of 0.730 [51].
Functional biomarkers
While SFN is an obviously recognizable diagnosis primarily diagnosed by history and clinical examination in patients with known risk factors such as diabetes, objective test confirmation is recommended for patients without known risks. The non-specific symptoms of SFN further emphasize the need for selecting biomarkers to exclude the occurrence of other neuropathies. Thus, diagnostic confirmation may be provided by functional neurophysiological tests and histological skin biopsies. However, it is worth noting that although these tests may help in diagnosing neuropathy, they may have limited value in assessing NeP in SFN [57, 58].
One functional neurophysiological test for the cardiovascular adrenergic function is the Valsalva maneuver (VM) test. The VM test evaluates baroreceptor sensitivity, as baroreceptors play a pivotal role in blood pressure regulation [56] and blood pressure recovery time (BPRT) is an often-used index of adrenergic baroreflex function. The patient with suspected SFN is rested and recumbent and asked to maintain a column of mercury at 40 mmHg by forcefully breathing out of their mouth with a tightly closed nose and straining for 15 s. Repeated tests are conducted with the patient tilted at a 20°–40° angle until a significant reduction in blood pressure is recorded [59]. In addition, the tilt table test measures changes in blood volume due to gravity redistribution in the transition to an upright angle between 60° and 80°. When standing from a supine position, excessive pooling of blood in the lower limbs is similarly prevented by baroreflex that is controlled by adrenergic outflow [60]. Positive tilt table test results may be present in patients with SFN due to abnormal changes in blood pressure, heart rhythm, or heart rate.
Amongst tests for the sudomotor function is the stimulated skin-wrinkling (SSW) test, which is a non-invasive indicator of limb sympathetic function [61]. SSW involves immersing the patient’s hands either in water or a EMLA, such as EMLA. The degree of wrinkling depends on vasoconstriction controlled by sympathetic nerve fibers in the glabrous hand and, to a lesser extent, foot skin [62]. EMLA-stimulated wrinkling showed 81.6% sensitivity and 74.7% specificity, whereas water wrinkling showed 71.4% sensitivity and 73% specificity in detecting abnormal IENFD in iSFN patients [63]. Studies have shown that compared to water wrinkling, EMLA produces a more linear response that persists for a longer time to allow for grading [62, 63]. As SSW is simple, cost-effective, and does not require specialized equipment, it is a practical clinical bedside test that has been implemented for routine use [64].
Other tests include quantitative sudomotor axon reflex testing (QSART) which measures the autonomic nerve fibers that control sweating to provide a quantitative assessment of postganglionic sudomotor function [65]. In the diagnosis of SFN, QSART has a sensitivity of 80% [66]. However, the test-retest reliability of QSART can be moderate with a large standard error of measurement [67], suggesting that the test is not ideally suited for longitudinal and interventional studies. Furthermore, QSART norms are only established for individuals who are 10 years or older [68] which excludes many suspected pediatric SFN patients. Hence, QSART should not be the only investigation modality used to confirm SFN.
In addition, QST assesses the psychophysical thresholds for cold and warm sensations, reflecting the function of the A–δ fibers. It is noninvasive, easily performed, and provides results that can be analyzed immediately to detect small fiber dysfunction [69]. A study found that QST is significantly more reproducible than QSART [70], so the two tests are often used in conjunction to improve the accuracy of results. Nonetheless, although QST has reasonable reproducibility, it has high inter-operator, inter-patient, and inter-test variability [71]. QST cannot differentiate between central and peripheral causes of temperature perception which means that any miscommunication between the examiner and patient would affect the results [72]. To improve the administration of QST in clinical practice and research, the Neuropathic Pain Special Interest Group of the International Association for the Study of Pain (NeuPSIG) recommended using predefined standardized stimuli and instructions, validated algorithms of testing, and established reference values corrected for the anatomical site, age, and gender [73]. When appropriate standards are applied, QST can provide valuable information about the functional status of the somatosensory system. Due to the disadvantages of QSART and QST, they are not recommended as stand-alone diagnostic confirmatory tests [74]. As such, the sensitivity of SFN diagnosis can be improved by combining the complementary assessments of somatic (QST) and peripheral autonomic small fiber function (QSART) [65]. The various autonomic and small fiber function tests that are clinically available for diagnostic confirmation of SFN following clinical examination are shown in Table 3.
Clinically available autonomic and small fiber function tests
Clinically available autonomic and small fiber function tests | What they measure | Modalities | Advantages | Disadvantages | Sensitivity/specificity | Reference |
---|---|---|---|---|---|---|
VM | Assesses the reduction in cardiac preload | Cardiovascular adrenergic | Provides quick results for analysis | Patients may experience increase in blood pressure | 80% Sensitivity, 80% specificity | [56, 59, 67] |
Tilt table test | Changes in blood volume due to gravity redistribution in the transition to an upright posture | Cardiovascular adrenergic | Provides quick results for analysis | Large range of long-term reproducibility (50–85%) | The sensitivity and specificity are difficult to establish | [56, 111] |
SSW test | Degree of wrinkling, which reflects vasoconstriction controlled by sympathetic nerve fibers in the glabrous hand | Sudomotor |
| Limited to the hands, because wrinkling in the feet is unreliable |
| [60–63] |
QSART | Quantitative assessment of postganglionic sudomotor function controlled by autonomic nerve fibers that cause sweating | Sudomotor | Able to assess the distribution of sudomotor impairment |
| Sensitivity of 80% | [16, 64–66] |
QST | Psychophysical thresholds for cold and warm sensations | Thermal sensation |
|
| - | [69, 70, 72, 73] |
Thermoregulatory sweat test (TST) | Percentage of anterior body surface anhidrosis | Sudomotor |
| Patients experience thermal discomfort of the heat stimulus which makes them reluctant to undergo repeat testing | 80% Sensitivity | [56] |
Sympathetic skin response (SSR) | Momentary changes in electrical potentials associated with induced sweating in palms and soles | Sudomotor | Simple to implement |
| 87.5% Sensitivity88.2% Specificity | [56] |
-: information not available
There are other measures to assess sweat glands that are not validated for routine clinical use as of yet, such as the dynamic sweat test that assesses sweat gland density, distribution, and stimulated sweat production [75, 76]. To improve neurophysiological assessment by overcoming the inaccessibility of small nerve fibers with conventional nerve conduction tests, nociceptive laser-evoked potentials (LEPs) or contact heat-evoked potentials (CHEPs) can be recorded [77]. A recent study of Chinese adults found that CHEPs had a high reproducibility in diagnosing SFN [77]. Amplitudes of Aδ evoked potentials and leg skin flare responses from CHEPs were reduced in patients with sensory neuropathy compared to healthy controls (HCs) [78], and diagnostic accuracy was 74% compared to IENFD [79]. LEPs strongly associated with pinprick sensory disturbances at a sensitivity of 78% and specificity of 81% for the diagnosis of diabetic SFN [80].
Morphological biomarkers
Amongst multiple diagnostic tests available, skin biopsy has the highest accuracy to date in the diagnostic confirmation of SFN, in particular, a skin biopsy showing decreased IENFD [11]. In the past, objective confirmation of SFN required a surgical biopsy of a sensory nerve which is invasive, expensive, and hence only rarely performed [12]. Today, a skin biopsy is valued because it is minimally invasive and specifically assesses small fibers, unlike standard nerve conduction studies and electromyographic tests which are typically used to diagnose large fiber neuropathy [17]. Despite its advantages, skin biopsies are mainly limited by test insensitivity due to poor non-representative or published norms, especially in pediatric and female patients, sampling error, and symptoms typically appearing before axons degenerate [17].
Overall, skin biopsies have a sensitivity of 81–88% in diagnosing SFN [18]. Skin biopsy samples are first stained for protein growth product 9.5 (PGP 9.5, a pan-axonal antigen) which reveals small fiber axons with light or fluorescence microscopy [18]. Thereafter, these samples are sent to laboratories where they are processed and measured for IENFD relative to biopsies from healthy, demographically matched volunteers [18, 81, 82]. According to the European Federation of Neurological Societies, IENFD from a distal leg skin biopsy is reliable and efficient to confirm SFN, and laboratories should provide their own normative values, stratified for age, gender, and intra- and inter-observer reliability [81]. SFN diagnosis is confirmed in patients with a measured epidermal nerve density below the fifth percentile of the anticipated normal distribution. If false negative results occur, second biopsies (e.g., from the thigh for non-length-dependent neuropathic conditions or the foot [18, 83–86]) may add sensitivity but cost as well. There are also insufficient norms to recommend routine use.
Several studies have further improved the accuracy of skin biopsies for SFN diagnosis. A worldwide normative data set for IENFD of the distal leg by bright-field microscopy and indirect immunofluorescence corrected for age and gender has been established [82, 86]. Compared to conventional bright-field microscopy, immunofluorescence visualizes twice as many fibers and requires its own set of norms [81, 86]. Ongoing research into other biopsy sites and measures includes the density of sweat glands or arrector pili innervation, but more validation is needed before they can be implemented clinically [17].
In vivo corneal confocal microscopy visualizes the exclusively C-fiber innervation of the cornea is non-invasive and is effective for longitudinal tracking, but it is not yet a routine method [87]. More recently, SFN was identified using peripheral nerve ultrasound which shows an enlarged cross-sectional area of the sural nerve, although more research is needed before it can be implemented as a diagnostic method [88, 89].
Molecular biomarkers
If clinical examination, functional neurophysiological tests, and skin biopsies confirm SFN without a known secondary cause, these patients are labeled as “initially idiopathic” (iiSFN) and the next step would be to screen for occult causes [12]. Firstly, iiSFN patients are screened using medical history and prior test results. Secondly, hematological tests, lumbar punctures, or imaging are performed, identifying potentially treatable causes in 30% to 50% of iiSFN patients [10, 90, 91]. Thirdly, genetic sequencing is recommended for patients with a high probability of genetic causes. Therefore, the diagnostic performance and cost-effectiveness of specific tests must be defined for clinicians to decide on how to screen iiSFN patients for causes in an accurate yet optimized way.
Blood tests
Blood tests are an effective method to identify occult causes of iiSFN. Sensory and autonomic-predominant polyneuropathies are associated with abnormal blood test results (ABTRs) for diabetes, alcohol-related liver dysfunction, heavy-metal toxicity, deficiencies of vit B12 (cobalamin) and B1 (thiamine), hypothyroidism and hyperthyroidism, paraproteinemia, sarcoidosis, and systemic autoimmune disorders including SS, SLE, and celiac disease. Infectious causes include HIV, hepatitis B and C, leprosy, and Lyme disease [12]. However, not all of these above-mentioned require testing, rather, the choice of blood tests should be determined according to the clinical context and patient demographics (Table 2).
A large cohort of 921 pure SFN patients showed that additional underlying conditions were found in 26.7% of patients with known possible underlying conditions [10]. It was recommended that patients with pure SFN, should at least be screened for autoimmune diseases, SCN gene mutations, DM, glucose intolerance, and vit B12 deficiency, even when they already have a potential underlying condition at referral [10].
Individual medical conditions tested might be specifically associated with iiSFN and their related blood tests could determine the cause of iiSFN. A 2016 SFN study evaluated the 21 widely-available, recommended blood tests to determine the prevalence of each ABTR in the iiSFN cohort compared to population averages. High ESR and ANA (≥ 1:160 titer) were each presents in 28% of subjects. High ESR, high ANA, low complement component 3 (C3), Sjögrens and celiac autoantibodies were also present with 3 times the greater prevalence in iiSFPN than in the total population. Overall, these ABTR results suggest the possibility of a specific association between iiSFPN and dysimmunity.
Promising results on plasma neurofilament light chain (Nfl) as a biomarker for large fiber neuropathy may potentially be used for patients with SFN. Nfl is a cytoskeletal protein specific for neurons, but the exact mechanism by which they are released is not completely understood [92]. Nfl levels are higher in patients with Charcot-Marie-Tooth disease [93], neuropathy due to amyloidosis [94], chemotherapy-induced peripheral neuropathy [95], chronic inflammatory demyelinating polyneuropathy [96], and prediabetes [97]. Higher levels were found to correlate with disease severity [93, 94]. Its role in patients with SFN needs to be determined.
Genetic tests
Secondary genetic sequencing should be considered for adult patients with iSFN after unyielding primary tests, and all pediatric cases, especially those with a family history. The types of genetic tests used can be selected based on a patient’s clinical presentation. Multisystemic diseases leading to SFN such as amyloidosis and Fabry disease will present with other organ dysfunction and involvement. On the contrary, sodium channelopathies, acute sensory neuritis, small fiber sensory Guillain-Barré syndrome (GBS), and HSAN present with isolated SFN symptoms [11]. As more genes are implicated, costs drop and more precision treatments become available, there is much promise in the development of genetic sequencing, and may potentially allow the creation of polygenic risk scores for SFN [17].
Upon sequencing of patients’ genes, these results are sent to laboratories for analysis. Among 1,519 patients referred to the National Institutes of Health Undiagnosed Diseases Network for advanced testing, nearly half were referred for neurological symptoms. Among all diagnostic tools, whole-exome sequencing and whole-genome sequencing were found to be the most successful, providing molecular diagnoses in 74% of these patients [98]. Thus, it was found that molecular biomarkers as diagnostic tools for SFN can curtail further testing, switch treatments from palliative to precision-targeted to improve efficacy and safety, sometimes reduce cost, and influence reproductive planning [99]. Although genetic testing results might be inconclusive, genetic causes of SFN cannot be entirely excluded because our understanding of genetic testing is still evolving. Currently, laboratories are limited in their selection of sequences by a lack of available medical information, resulting in varying depth and quality of their analyses. As new findings emerge, initially “unhelpful” sequencing results may come of use for periodic reanalysis in the future [17].
Autoimmune biomarkers
Antibodies have long been associated with large fiber neuropathies and play a role in classifying the disease phenotype [100]. Although the pathophysiological mechanisms of SFN remain largely unknown, there is limited preliminary evidence showing that patients with SFN respond to immunotherapies and this correlates with the presence of auto-antibodies in SFN patients [101]. As SFN is a diagnostically and therapeutically challenging disease, further understanding of its pathophysiology is required to confirm its association with antibodies and to determine targeted treatments for SFN patients [102]. SFN may involve multiple immunological processes. Despite these SFN-associated autoantibodies, further validation and testing are required to determine pathogenicity for most of them.
Anti-sulfatide [immunoglobulin M (IgM)] antibodies have long been implicated in the sensory component in small and mixed fiber neuropathies. They are associated with acute onset in all SFN patients tested [103]. Steroid therapy was administered in the form of oral prednisone therapy to patients with acute SFN and there was a significant improvement in their clinical symptoms 1–2 weeks later. Of the four patients, three remained free of symptoms, and one experienced a recurrence of neuropathy after prednisone therapy ceased. However, these studies involve retrospective data analysis of clinical tests, and the level of evidence is low and merely associative [104].
Another study consisting of 155 patients with biopsy-confirmed iSFN was blindly tested for serum anti-tri-sulfated heparin disaccharide (TS-HDS) (IgM) antibodies. Of all the SFN patients tested, 48% had serum antibodies, and 37% specifically with anti-TS-HDS (IgM) antibodies. Compared to controls, anti-TS-HDS (IgM) antibodies were more frequent in SFN patients (P = 0.0012). Ninety-two percent of patients with acute-onset SFN had anti-TS-HDS (IgM) antibodies, which were more common in females and associated with NLD-SFN pathology. Hence, the presence of auto-antibodies in otherwise iiSFN patients’ sera, suggests that they may be used as markers for certain SFN subsets [104, 105].
There is also a suspected association between fibroblast growth factor receptor 3 (FGFR3) antibodies in the diagnosis of SFN, but its specificity remains unclear. In one study, 15% of iiSFN patients had serum anti-FGFR3 (IgG) antibodies present and in another, 17% had elevated serum anti-FGFR3 (IgG) antibodies [104, 105]. Although both TS-HDS/FGFR3 antibodies are found to be present in many SFN patients, more studies are needed to validate the role of these antibodies in the pathogenesis and clinical utility of immune-mediated dysautonomia.
Other novel autoantibodies for NeP include anti-plexin D1 antibodies. Plexin D1-IgG was originally detected in patients with NeP with underlying neuroinflammatory diseases, including atopic myelitis, multiple sclerosis, and neuromyelitis optica spectrum disorders [106]. Compared to control subjects, plexin D1-IgG antibodies were more frequent in patients with NeP (10% vs. 0%; P < 0.05). Furthermore, the application of anti-plexin D1 antibody-positive patient sera to cultured dorsal root ganglion (DRG) neurons increased membrane permeability and caused cellular swelling. This observation explains why patients with NeP and positive anti-plexin D1 antibodies commonly developed burning pain and current perception threshold abnormalities for C-fibers. Another study found that 75% of patients with iSFN present with a chronic persistent prickling or burning pain in a length-dependent manner [107]. There was a significant positive correlation between antiplexin D1-IgG levels with disease duration. This suggests that either a longer time is needed for SFN to fully develop in plexin D1-IgG, or that plexin D1-IgG may emerge due to long-term small fiber neuron damage because patients with secondary SFN caused by DM also carried plexin D1-IgG [108]. Although anti-plexin D1 (IgG) antibodies were confirmed to be pathogenic in SFN via mouse models, this study did not histologically confirm definite SFN in the test subjects [108].
One study confirmed an association between antibodies against SCNs, anti-Nav (IgG) antibodies, and SFN in cell-based assays [109]. The patients assessed had an SFN variant of GBS and presented with acute onset burning sensations in a length-dependent distribution. These patients were treated with intravenous Ig and it was found that IgG antibodies in their acute phase sera bound strongly to murine small nerve fibers, but this binding ceased during the convalescent phase. Additionally, the study observed that serum IgG transfer to a murine nociceptive model caused transient alteration in thermal pain responses. Hence, this suggests that anti-Nav (IgG) antibodies may induce an acute transient immune response directed at small nerve fibers [109].
More novel autoantibodies were recently reported by one study, in which researchers used a novel antibody technology [101] to identify putative autoantibodies in SFN using sera from SFN patients and age, gender-matched HCs that were screened against > 1,600 immune-related antigens on the patented array [110]. The results from this study elucidated that novel autoantibodies interferon-induced GTP-binding protein Mx1 (MX1), drebrin-like protein (DBNL), and cytokeratin 8 (KRT8) are found in iSFN, while MX1 in particular may enable diagnostic subtyping of iSFN patients as it was found to be higher in iSFN compared to secondary SFN. Hence, this study adds strength to the concept that an immune-mediated process may be involved in a subset of SFN. It provides a stepping stone for more studies to determine the pathophysiology of SFN for clinical subtyping and the development of targeted treatments for iSFN patients, who are currently treated symptomatically rather than causally.
A summary of the various auto-antibodies relating to their clinical significance and the level of evidence establishing their association with SFN is shown in Table 4.
Autoantibodies associated with SFN
Autoantibody | Clinical significance | Level of evidence | References |
---|---|---|---|
Anti-sulfatide (IgM) |
| Low; retrospective data analysis | [102, 103] |
Anti-TS-HDS (IgM) |
| Low; retrospective data analysis | [104, 105] |
Anti-FGFR3 (IgG) |
| Low; retrospective data analysis | [104, 105] |
Anti-Plexin D1 (IgG) |
| High; animal studies confirming pathogenicity | [106, 108] |
Anti-Nav (IgG) |
| Low; cell-based assays, but pathogenicity not confirmed | [109] |
Anti-MX1 (IgG) |
| Low; no mechanistic studies | [101] |
LD: length dependent
Conclusions
The selection of appropriate biomarkers would improve patient stratification and management of SFN, whether secondary or idiopathic. As SFN patients often present with non-specific symptoms, clearer guidelines for SFN diagnosis would help clinicians to identify symptoms and implement tests that characterize SFN. However, awareness of the advantages and limitations of a given biomarker is important to minimize false positives and false negatives.
As outlined in this review, the diagnostic approach to SFN should be done in a stepwise manner, with consideration for each patient’s gender, age, ethnicity, and locality. Firstly, preliminary diagnosis can be made from clinical examination and history-taking, which usually identify patients with secondary causes of SFN such as DM. Secondly, diagnostic confirmation may be provided by functional neurophysiological and histological tests. Objective test confirmation is especially important for patients without known risk factors, because although they may present clinically with SFN symptoms, NeP may be due to other causes distinct from SFN. Thirdly, iiSFN patients without a known secondary cause are screened using blood tests and genetic sequencing to determine underlying etiology. Depending on the specificity of the patient’s symptoms and history, autoimmune tests can be administered for clinical subtyping if dysimmunity is suspected as an occult cause of iSFN.
By determining the etiology of iSFN, biomarkers can enable the development of better-targeted treatments that address specific causes of SFN. Significant breakthroughs have been made in developing and improving biomarkers of SFN, yet there is still much to uncover. We hope that this review encourages further studies to dispel the mystery surrounding SFN, and to enhance the diagnosis, treatment, and monitoring of this disease that has affected the lives of so many.
Abbreviations
ABTRs: | abnormal blood test results |
ANA: | anti-nuclear antibody |
CHEPs: | contact heat-evoked potentials |
COMPASS: | composite autonomic symptoms scale |
CT: | computerized tomography |
DM: | diabetes mellitus |
EMLA: | eutectic mixture of local anesthetics |
ESR: | erythrocyte sedimentation rate |
FGFR3: | fibroblast growth factor receptor 3 |
HIV: | human immunodeficiency virus |
HSAN: | hereditary sensory autonomic neuropathy |
IENFD: | intra-epidermal nerve fiber density |
IgM: | immunoglobulin M |
iiSFN: | initially idiopathic small fiber neuropathy |
iSFN: | idiopathic small fiber neuropathy |
LD-SFN: | length-dependent small fiber neuropathy |
MX1: | interferon-induced GTP-binding protein Mx1 |
Nav 1.7: | sodium channel subunit 1.7 |
NeP: | neuropathic pain |
Nfl: | neurofilament light chain |
NLD-SFN: | non-length-dependent small fiber neuropathy |
PET: | positron emission tomography |
QSART: | quantitative sudomotor axon reflex testing |
QST: | quantitative sensory testing |
SCN: | sodium channel |
SFN: | small fiber neuropathy |
SFN-SIQ: | small fiber neuropathy Symptoms Inventory Questionnaire |
SFN-SL: | small fiber neuropathy Screening List |
SLE: | systemic lupus erythematosus |
SS: | Sjögren’s syndrome |
SSW: | stimulated skin-wrinkling |
TS-HDS: | tri-sulfated heparin disaccharide |
UENS: | Utah Early Neuropathy Scale |
vit B12: | vitamin B12 |
VM: | Valsalva maneuver |
Declarations
Author contributions
ACYC, KO, and JV: Conceptualization. KO, ACYC, JV: Writing—original draft, Writing—review & editing. All authors contributed to manuscript revision, read and approved the submitted version.
Conflicts of interest
The authors declare that they have no conflicts of interest.
Ethical approval
Not applicable.
Consent to participate
Not applicable.
Consent to publication
Not applicable.
Availability of data and materials
Not applicable.
Funding
ACYC is supported by the Singapore Ministry of Health’s National Medical Research Council [MOH-000437-0] under its NMRC Research Training Fellowship. The funders had no role in study design, data collection and analysis, decision to publish, or preparation of the manuscript.
Copyright
© The Author(s) 2022.