Abstract
Early in the course of infection, human immunodeficiency virus (HIV) is able to enter the central nervous system where it stablishes a permanent reservoir. Current antiretroviral therapies do not efficiently cross the blood-brain barrier and therefore do not reach the HIV located in the central nervous system. Consequently, HIV infection can often be associated with neurocognitive impairment and HIV-associated dementia. The purpose of this review is to brief the reader into the world of neurological complications arising from HIV infection. Mechanisms by which HIV directly or indirectly impairs the central nervous system are discussed, as well as other factors influencing or contributing to the impairment, and the animal models currently used to perform research on the topic.
Keywords
HIV, neurological complications, HIV-associated neurocognitive disorders, dementia, inflammationIntroduction
Human immunodeficiency virus (HIV) is able to enter the central nervous system (CNS) early in the course of the infection where it stablishes a reservoir. In fact, the brain is seen as both, a reservoir and a hideaway for HIV [1, 2]. HIV infection can be efficiently suppressed with antiretroviral therapy (ART) but the existence of long-term reservoirs allows the viral load to rebound quickly after ART interruption. Therefore, HIV infection is currently chronic and incurable, requiring constant therapy to maintain clinical stability and low viral loads. Unfortunately, current antiretroviral therapies do not efficiently cross the blood-brain barrier (BBB) and therefore do not optimally reach the HIV found in the CNS [3–5]. Consequently, HIV infection can often be associated with neurological damage, including neurocognitive impairment and HIV-associated dementia (HAD) [6, 7]. Typical symptoms include loss of concentration, attention, and memory, irritability, depression, and slowed movements [8]. The severity of the symptoms can range from asymptomatic to HAD in the most severe cases.
Recent studies indicate that CNS impairment is at least partially induced by subtle damage in synapsis and dendrites, thus mediating disruption of neuronal networks in the brain that are involved in learning, memory and executive functions [8]. Because HIV replication in the CNS occurs principally in microglia (the resident macrophage-type cells), is thought that both, direct effects of viral proteins, and indirect effects mediated by microglia activation and secretion of neurotoxic products can contribute to neuronal damage [9, 10]. However, the causes for such neurological dysfunctions are diverse and will be discussed in this review. Overall, HIV infection can cause disorders of the nervous system in at least three ways: 1) directly or indirectly by the HIV itself; 2) by complications related to the toxicity of the antiretroviral drugs used to control the viral loads; and 3) by opportunistic infections or certain cancers associated with HIV infection but not caused directly by the HIV virus.
HIV infection
HIV is a lentivirus that infects the very cells of the immune system in charge of fighting off incoming pathogens. There are two types of HIV: HIV-1 and HIV-2. Both originated by zoonotic transmission from non-human primates [11]. HIV-1 is a pandemic virus: approximately 36 million people are currently living with this virus around the world. Conversely, HIV-2 is predominantly present in West Africa. While HIV was discovered forty years ago, and despite strenuous efforts from the scientific community, a successful vaccine for this disease is yet to be found [12]. With almost a million deaths per year, the disease has become a global public health priority.
At the molecular level, the infection process is mediated by the HIV envelope spike, which attaches to cell-surface CD4 and then to a cellular co-receptor, such as chemokine receptors CXCR4 or CCR5. HIV infects predominantly CD4 helper T-cells but it can also infect macrophages, monocytes and dendritic cells. HIV infection is characterized by a progressive decline in number and function of CD4 T-cells, leaving the host vulnerable to opportunistic infections. If the HIV infection goes untreated, most individuals eventually develop acquired immunodeficiency syndrome (AIDS) and die in an 8–10 years’ time-period. While both HIV-1 and HIV-2 can cause AIDS, HIV-1 mediates a faster decline of CD4 T-cells and onset of the disease [13]. Fortunately, due to the advent of ART, which is able to reduce the viral load to undetectable levels, AIDS-associated morbidity and mortality have significantly dropped. However, HIV is able to seed reservoirs early in infection, allowing the viral load to rebound after treatment interruption [14–16]. Thus, HIV infection is nowadays a chronic disease with the need for constant therapy to maintain clinical stability and low viral loads.
Crossing barriers
HIV is able to enter the CNS early in the course of infection, where it establishes a permanent reservoir. The CNS, which comprises brain, cerebellum and spinal cord, performs a plethora of key functions such as receiving and processing sensorial information, controlling voluntary and involuntary body movement, reflexes, and regulating homeostasis and body temperature. It is also where complex functions such as thinking, memory and learning reside. Due to its paramount importance, the CNS is protected and isolated from the rest of the body by the BBB. It has what is called immunoprivileged status [17, 18]. The BBB is formed by brain endothelial cells sealed with tight junctions and is highly selective in restricting the transport of both, cells and molecules, from the blood into the CNS [19]. The CNS is surrounded by a colorless body fluid, different from blood: the cerebrospinal fluid (CSF). The tightly regulated protection imposed by the BBB is aimed at preventing pathogens from reaching the CNS and to help maintaining a constant and optimal local environment [18].
Both HIV-1 and HIV-2 are able to efficiently cross the BBB early in infection [20, 21]. HIV can enter the CNS using a “Trojan horse” strategy: it infects monocytes or macrophages in the periphery, some of which will eventually cross the BBB, before the progeny viruses are released (see Figure 1). Moreover, free viruses can also transit across BBB through endothelial cells [22]. Once in the CNS, HIV is able to infect several cell types expressing CD4 on their cell-surface, including macrophages, microglia (also of macrophage lineage) and astrocytes [23, 24]. In fact, HIV isolates obtained from the CNS are mostly CCR5-using strains (i.e. with macrophage tropism) rather than CXCR4-using strains (i.e. with T-cell tropism) [9]. There has been some debate on whether neurons themselves can be infected with HIV [25]. While it has been reported [26, 27], neuronal infection seems largely non-productive, i.e. the virus is able to enter the cells but unable to produce a viral progeny [28].
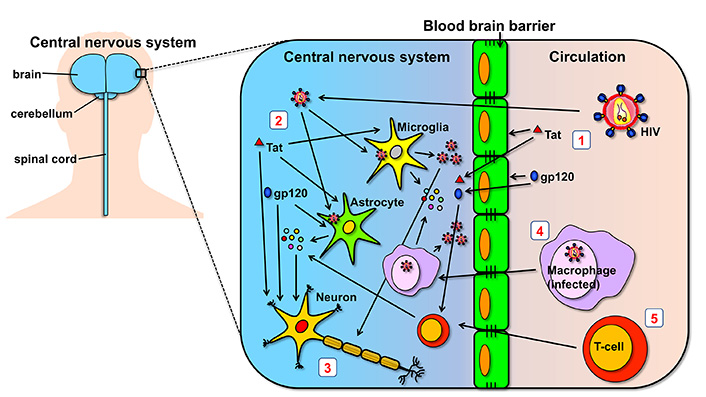
Summary of mechanisms of neuropathogenesis in the CNS. (1) HIV and HIV proteins glycoprotein 120 (gp120) and trans-activator of transcription (Tat) found in circulation are able to cross the BBB and reach the CNS. Importantly, they can cause direct damage to the cells forming the BBB; (2) once in the CNS, HIV is able to infect and replicate in microglia and astrocytes, cells which may also be affected by the direct toxic effects of Tat and gp120; (3) HIV virions and the two viral proteins, gp120 and Tat, can generate neuronal damage directly by acting on neurons, and indirectly by the cytokines that are released by bystander cells; (4) HIV can also cross the BBB by travelling inside macrophages which were infected in the periphery, using what is called the “Trojan horse” strategy; (5) similar to macrophages, T-cells can also infiltrate the CNS and have a role in neuroinflammatory processes. Although not indicated in the figure, HIV can also use T-cells as Trojan horses
Current antiretroviral therapies are extremely efficient at decreasing peripheral viral loads to undetectable levels. However, these drugs do not efficiently cross the BBB and therefore do not optimally reach the HIV found in the CNS [3–5]. Consequently, HIV infection can often be associated with neurological damage, including neurocognitive impairment and HAD [6, 7].
Neurological complications associated with HIV infection
People living with HIV can frequently suffer from HIV-associated neurocognitive disorders (HAND). These disorders include three categories: asymptomatic neurocognitive impairment (ANI), mild neurocognitive disorder (MND), and HAD [29–31]. ANI, the mildest form of HAND is defined by substandard performance (1 standard deviation below the mean) in at least two of the so-called “cognitive areas”, which include fine movement, fluency, executive functions and memory, when individuals are tested for such neurocognitive abilities. By definition, no impairment with daily functioning should be observed in the individual. While often these mild impairments, despite being detected by the aforementioned tests, can pass unnoticed by the affected individual, ANI is associated with an increased risk for symptomatic decline [29]. Therefore, early detection is important for proper management. The second form of HAND, MND, involves substandard performance (1 standard deviation below the mean) in at least two cognitive areas, similar to ANI, but with the addition of, at least mild, interference with daily functioning. Lastly, HAD is the most severe form of HAND. HAD is characterized by a worse performance in at least two of the aforementioned cognitive areas (2 standard deviations below the mean) and below-average performance in neuropsychological tests. Marked impairment in daily activities should be found too [29–31]. Besides these primary HAND, secondary cognitive limitations may also ensue, due to other factors.
Other common HAND include: a) peripheral neuropathy (HIV-related damage to peripheral neurons) [32]; b) vacuolar myelopathy, which is a disease of the spinal cord characterized by removal of the protective myelin sheath from nerve cells of the spinal cord, thus forming small holes or vacuoles. Symptoms include weak and stiff legs and shakiness when walking [33]. And lastly, c) immune reconstitution inflammatory syndrome (IRIS), which starts as an aberrant reconstituted immunity during ART and results in acute inflammatory responses in the host. Individuals with IRIS have a transient improvement of their HIV infection after starting ART, but then develop complications related to exacerbated inflammation [34]. IRIS can occur in two forms: “unmasking” IRIS, which refers to an exaggerated immune response developed towards a previously undiagnosed infection; and “paradoxical” IRIS, referring to the worsening of a previously recognized and treated infection. Importantly, IRIS can present in the CNS [35]. CNS-IRIS develops in a significant percentage (9–47%) of people living with HIV who have an opportunistic infection in the CNS and start ART. Opportunistic infections in the CNS, such as toxoplasmosis, cryptococcal meningitis, and tuberculous meningitis, are not rare in untreated people living with HIV [36, 37]. Depending on the causative pathogen, the mortality rate of CNS-IRIS can vary from 13% to up to 75% [38].
The CNS is arguably one of the main targets of HIV-1 infection, but viral replication in this compartment is typically limited by the availability of target cells [20]. It is thought that the CNS is usually affected during the HIV primary infection, and that injury is innocuous and largely asymptomatic for the first few years. However, it can lead later on to HAND, stage at which many opportunistic infections can also affect the CNS [39].
Viral proteins as neurotoxins
The HIV virus encodes a number of proteins in its genome, some of which are known to cause damage to neural cells, including both neurons and neuroglia. The most well-known proteins to induce such problems are gp120 and Tat. Gp120 is part of the envelope or spike that the virus uses to enter the cells. Gp120 is often shed from infected cells or virions into circulation. Conversely, Tat is a small multifunctional protein involved in the control of the transcription of the HIV genome [40]. Despite having intracellular functions, Tat can be secreted [41] and has the intriguing property of entering bystander cells via a cell-penetrating peptide sequence [42]. Through this process, Tat can contribute to immune activation and cell death of bystander cells [43]. Importantly, both proteins have been found in CSF from HIV infected people, even when those individuals were under ART [44]. Accordingly, the effects of these proteins are not only seen in the periphery but also locally in the CNS (see Figure 1).
Gp120 has been identified as a potent neurotoxin promoting neurodegeneration via indirect and direct mechanisms involving interactions with CCR5 and CXCR4 [45, 46]. Besides being found in immune cells, these chemokine receptors are also generally expressed in neurons and some other cell types in the CNS [45, 47]. Paradoxically, evidence for a CCR5-mediated neuroprotective pathway has also been demonstrated through heterologous desensitization of CXCR4 [48]. While mostly studied during HIV infection, CCR5 has also been linked to Alzheimer’s disease [49], and it can influence neuron survival [47]. CCR5 has also been recognized as a therapeutic target for recovery after stroke and traumatic brain injury [50].
Gp120 has a role in disrupting costimulatory signals in T-cells, more specifically the adenosine deaminase (ADA)-CD26 axis [51]. ADA is an enzyme that can be found inside the cells, where it catabolizes adenosine; but also outside the cells, where it exerts both enzymatic and extra-enzymatic functions [52]. The ADA-CD26 interaction occurs on the cell surface and provides costimulatory signals during T-cell activation [53]. These signals are involved in T-cell differentiation, and the acquisition of immunological memory [53–56]. The gp120-mediated impairment of costimulation [51], and cytokine production [51, 54] may have significant relevance during HIV infection and AIDS pathogenesis [52, 57]. Because T-cells can infiltrate the CNS [58], it is reasonable to think that the HIV gp120 found in the CNS may disrupt the ADA-mediated costimulatory signals on those T-cells too. Emerging studies have shown a fundamental role for infiltrating T-cells in neuroinflammatory processes, as a key mechanistic piece leading to subsequent neurodegeneration in neurodegenerative disorders such as Parkinson’s disease, Alzheimer’s disease, amyotrophic lateral sclerosis and multiple sclerosis [59–62]. Because of the exacerbated inflammation associated with HIV infection, infected individuals may be more prone to develop such neurodegenerative diseases [63, 64].
Tat protein is known to contribute to neuropathogenesis in several ways, including its ability to be taken up by uninfected bystander CNS cells, and to activate inflammatory host genes causing synaptic injury [42]. Tat can also modulate proliferation and differentiation of human neural precursor cells [65] and impair neurogenesis [66].
Substance abuse seems to synergistically enhance the neurological damage mediated by some HIV proteins. For instance, methamphetamine and Tat protein cooperatively induce oxidative stress and BBB damage [67]. The presence of Tat in the CSF has been associated with previous abuse of psychostimulants, such as cocaine or amphetamines, and poorer performance in information processing and psychomotor speed [44]. Interestingly, it has been proposed that Tat in the CNS can mediate potentiation of the reward mechanisms associated with cocaine, opioid and alcohol use [68] and therefore potentiate the use of such substances. Glial CCR5 has also a critical role in directing neuropathological interactions of HIV-1 Tat and opiates [69].
In terms of treatment and management of HIV-related neurological complications, ART has been shown to improve cognitive function. In fact, increases in CD4 counts and decreases in viral loads correlate with cognitive improvement [70, 71]. However, despite management with ART, HIV-1 induced dysfunction of the CNS continues to persist. Important factors to consider include the different penetrability of the individual ART drugs in the CNS and their potential toxicity [72].
ART-associated toxicity or virus-mediated damage?
AIDS-associated morbidity and mortality have dropped considerably since the advent of ART in the mid 1990’s. However, long-term use of antiretroviral therapy is not free of long-term complications. Some of the problems appear to relate to long-term side effects associated with the HIV medications themselves. These include lipodystrophy, insulin resistance, decrease in bone density, and lipid abnormalities [73]. Others, specifically chronic immune activation, inflammation, and hypercoagulation, seem to relate to the virus itself [74]. While a number of causes have been proposed, microbial translocation in gut is surely a critical factor [74–76]. It is also possible that some low-level replication of HIV in the presence of antiviral drugs could contribute to the chronic immune activation. It also seems probable that damage to the gut mucosa during the first weeks of HIV infection never gets adequately repaired, being an important factor in the chronic activation of the immune system in the face of effective therapy.
Chronic inflammation, and immune activation are key contributors to the CNS impairment. While ART can partially reverse the severity of the HIV-induced dysfunction in the CNS, the injure generally continues to persist. The problem likely relays on the bioavailability of the ART drugs in the brain, since they do not efficiently cross the BBB, and concentrations in the CSF are not an accurate measure of concentrations in the brain [77]. In an attempt to guide physicians, CNS penetration scores have been calculated for current ART drugs. While better scores have been correlated with more efficient suppression of CSF viral loads, and even some studies found improvement of HAND with that treatment, others could not find improvement in neurocognitive performance [77]. Differences may relate to the aforementioned disparities between drug concentration in CSF versus brain, since drug concentrations can be measured in the CSF but not in the brain, without risky invasive procedures. In some of these studies, enhanced CNS penetration was actually detrimental, highlighting that long-term use of ART can also result in toxicity to the CNS [77], which can exacerbate the damage from the virus itself.
All in all, while ART definitely helps in improving HAND and other neurological complications, long-term treatment can incur in toxicity to the CNS. Therefore, the selection of the ART combination must be done carefully. Novel drugs and therapeutic approaches are needed to improve the availability of ART drugs in the brain while reducing their toxicity [39, 72, 77].
Co-infections, cancer and external factors affecting the CNS
People living with HIV are often more susceptible to other infections and certain types of cancer [78, 79]. Despite overall risks have significantly decreased during the ART era, common HIV co-infections include tuberculosis and hepatitis B and C. The types of cancer with higher incidence in the HIV positive population include Kaposi’s sarcoma, Hodgkin’s lymphoma, non-Hodgkin lymphoma, cervical cancer, anal cancer, liver cancer and lung cancer [79, 80]. In terms of neurological impairment, hepatitis C virus (HCV) co-infection has been often associated with a greater risk for neurocognitive disorders in HIV infected individuals [81, 82]. This may be related to enhanced HCV-related immune activation, both systemic and in the CNS. In fact, chronic HCV infection has been associated with extrahepatic neurological conditions, even in the absence of HIV infection. Those affections include cerebrovascular events, encephalopathy, myelitis, cognitive impairment, and motor and sensory peripheral neuropathy (reviewed in [83] and [84]).
Other viruses that can negatively affect cognitive functions in HIV-infected individuals are Epstein-Barr virus (EBV) and Cytomegalovirus (CMV), due to increased inflammation [85]. EBV DNA can be detected in the CSF of many viremic individuals and some aviremic ones (on ART), and is associated with increased levels of neuronal damage and inflammation [86]. Higher levels of CMV and EBV DNA also correlated with multiple inflammatory markers and lower CD4/CD8 ratios [85] (the CD4/CD8 ratios are the number of CD4 T-cells per milliliter of blood divided by that of CD8 T-cells, and is usually between 1 and 4).
Neurosyphilis is caused by the bacterium Treponema pallidum when it reaches the CNS. Neurosyphilis is a complication of syphilis, a resurgent sexually transmitted disease. Progression to neurosyphilis is more common in individuals living with HIV [87, 88] and this may exacerbate ongoing neurological problems due to the HIV infection.
Important external factors that can exacerbate the damage to the CNS during HIV infection include the use of drugs and/or alcohol. Rates of substance use, abuse and dependence may often be higher among people living with HIV than comparable HIV negative populations [89]. A substantial minority of people living with HIV has comorbid substance use disorders which correlates with higher viral loads and less adherence to ART [90]. Overall, HIV infected individuals suffering from drug and/or alcohol abuse are at a greater risk of suffering neurocognitive disorders.
Depression and other mental health issues may also be exacerbated due to HIV infection and can contribute to poorer clinical outcomes.
Aging in people living with HIV
At the start of the HIV epidemic, newly diagnosed people could only expect to live a few years after their diagnosis. Therefore, issues related to aging were not a major focus at that time. Thanks to the development of successful ART able to control viral loads, people with HIV have now extended life expectancy, in some cases almost matching that of HIV negative individuals [91]. Therefore, the HIV population is an ever-aging population. For instance, according to the United States Centers for Disease Control and Prevention, of the estimated 1.2 million people living with HIV in 2018 in the U.S., about 51% were aged 50 and older. In addition, people aged 50 and older accounted for 17% of the new HIV diagnoses in the country that year. People living with HIV aged 50 and older are living longer, healthier lives because of effective ART. Nevertheless, the chronic inflammation observed during HIV infection, even in successfully treated individuals, appears to increase the risk for age-associated diseases. Furthermore, all effectively treated HIV-infected adults are increasingly manifesting signs of what is called “accelerated aging”, which includes neurocognitive disorders (HAND), cardiovascular disease, osteoporosis, and kidney disease. Older adults are often at greater risk of HIV-related neurocognitive dysfunction [92] and because the population living with HIV is aging, HAND and other HIV-related complications are already a growing problem [93].
Animal models of HIV infection in the CNS
The study of the neurological complications related to HIV infection has benefited from both in vitro models (reviewed by Faia et al. [94]) and in vivo models of HIV infection (reviewed by Mallard et al. [95]). Animal models have traditionally been very useful and complementary to research performed in human subjects across biomedical disciplines. Animal models allow for more controlled conditions in a laboratory setting, the use of more invasive techniques, extensive sampling, and the study of approaches otherwise not feasible or ethical to be performed in humans. Because CNS tissues are only available post-mortem, it is difficult to study HIV pathogenesis in living patients; therefore, animal models for the study of HIV pathogenesis in the brain have special relevance in this regard. Commonly used animal models to study effect of HIV in the CNS include rodents (mice and rats), and non-human primates. Because rodents are not naturally permissive to HIV infection, they have been generally used to test the neurotoxic effects of individual proteins from the virus. Remarkably, a transgenic rat model was developed, called HIV-1Tg rat, in which the rat brain contained seven of the nine genes encoded by the HIV genome, resulting in a non-infectious, long-term model of HIV protein exposure in the brain [96–99]. A mouse model was also developed in which HIV-1 infection can occur by viral re-targeting: the coding region of gp120 in HIV-1 was replaced with that of gp80 from ecotropic murine leukemia virus, a retrovirus that infects only rodents. The resulting virus is called EcoHIV. EcoHIV infection of mice seems able to establish latent viral reservoirs in T cells and active viral reservoirs in macrophages which are sufficient for induction of neurocognitive impairment [100]. The EcoHIV virus model allows to study systemic infection and neuroinvasiveness in the mouse model [101, 102]. Such experiments have recently revealed a novel nonneurotoxic viral function in hippocampal synaptodendritic injury and memory impairment [102].
Another remarkable rodent model includes humanized mice, which are generated by implanting human tissues and/or injecting human cells into various strains of immunodeficient mice (reviewed in [103]). Several types have been developed: the c-HIVE model in which HIV-infected myeloid cells are injected directly into the brains of severe combined immunodeficient (SCID) mice, to see if they contribute or not to HIV-associated encephalitis (HIVE); the PBMC-transplanted mice, in which human peripheral blood mononuclear cells (PBMCs) are inoculated; the hematopoietic stem cells-transplanted mice; and the “myeloid only mouse model”, to cite a few (see [103] for further details).
While murine animal models have certain limitations, such as divergent receptor sequence with humans, or differences in regional expression of cellular receptors [99], most of them have relatively low cost and allow for large sample size.
The most physiologically relevant model for HIV and HIV-related neurological complications is the non-human primate model. Genetically speaking, monkeys are more closely related to humans than rats or mice. Importantly, certain simian immunodeficiency viruses (SIVs) can induce AIDS in infected monkeys in a process that recapitulates the infection course seen in humans with HIV [104]. Several non-human primate models of neuroAIDS exist (reviewed in [105]). Traditionally rhesus macaques or pigtail macaques have been mostly used, together with different SIV clones or swarms able to induce AIDS, which had macrophage tropism, and induced SIV encephalitis [105]. Interestingly, some SIVs can induce rapid AIDS with progression to AIDS within 6 months after infection, like SIVmac17E-Fr [106]. While for others it may take longer (like for SIVmac239, SIVmac316 or SIVmac251) [95, 105, 107] and therefore better reflect the tempo of neuroAIDS progression in humans. Another molecular clone, namely SIVsm804E, is able to target the CNS and efficiently induce neuroAIDS in rhesus macaques [108]. A variant originated from that clone, SIVsm804E-CL757, causes a more delayed progression to AIDS, inducing encephalitis in a high proportion of inoculated animals, with high CSF viral loads, and multinucleated giant cells (microglial and/or macrophage cells that fuse with each other) in the CNS [108, 109].
Animal models have proven especially useful for research on substance abuse and HIV comorbidity [97, 110–114]. For instance, animal experiments have shown evidence that the presence of HIV-1 viral proteins in the CNS increases the sensitivity and susceptibility of HIV-positive individuals to substance abuse [97]. Continued research on the manifestation and mechanisms of neurological injury in HAND patients is needed. Animal models will be critical to help develop safe and effective therapies that reverse neuropathology and cognitive impairment [8].
Conclusion
People living with HIV can suffer serious neurological complications in at least three ways: 1) by mechanisms related to the HIV infection itself; 2) by the toxicity induced by the long-term use of ART used to control the HIV viral loads; and 3) by opportunistic infections and certain cancers associated with HIV infection but not caused directly by the HIV virus. Due to longer life expectancies in people living with HIV and an aging population, the severity of the symptoms and the number of people affected are expected to increase over time. While efforts continue to find a cure or vaccine for this disease, strategies for early detection, treatment, and mitigation of neurological complications of HIV infection are warranted.
Abbreviations
ADA: | adenosine deaminase |
AIDS: | acquired immunodeficiency syndrome |
ANI: | asymptomatic neurocognitive impairment |
ART: | antiretroviral therapy |
BBB: | blood-brain barrier |
CNS: | central nervous system |
CSF: | cerebrospinal fluid |
DNA: | deoxyribonucleic acid |
EBV: | Epstein-Barr virus |
gp120: | glycoprotein 120 |
HAD: | human immunodeficiency virus-associated dementia |
HAND: | human immunodeficiency virus-associated neurocognitive disorders |
HCV: | hepatitis C virus |
HIV: | human immunodeficiency virus |
IRIS: | immune reconstitution inflammatory syndrome |
SIV: | simian immunodeficiency virus |
Tat: | trans-activator of transcription |
Declarations
Author contributions
The author contributed solely to the work.
Conflicts of interest
The author declares no conflicts of interest.
Ethical approval
Not applicable.
Consent to participate
Not applicable.
Consent to publication
Not applicable.
Availability of data and materials
Not applicable.
Funding
Not applicable.
Copyright
© The Author(s) 2021.