Abstract
Acute ischemic stroke (AIS) is the leading cause of disability worldwide, and recanalization therapy is significant in the hyperacute phase of AIS. However, reperfusion injury and hemorrhagic transformation after recanalization predict poor prognosis of AIS. How to minimize reperfusion injury and hemorrhagic transformation, which greatly improves the prognosis of vascular recanalization, is becoming a hot topic in AIS research and an urgent problem to be solved. A wealth of neuroprotective drug studies is now available, while some of the neuroprotectants have met with failure in human studies. It is discussed in this review about the progress in neuroprotective therapy for AIS based on understanding the pathophysiologic mechanisms of reperfusion injury and hemorrhagic transformation, as well as challenges in exploring new neuroprotectants.
Keywords
Neuroprotective therapy, blood-brain barrier, acute ischemic strokeIntroduction
Acute ischemic stroke (AIS) is defined as the sudden loss of blood flow to an area of the brain with the resulting loss of neurological function due to the occlusion of cerebral vessels [1]. The pervasive influence of stroke is observed globally. According to The Global Burden of Diseases, Injuries, and Risk Factors Study in 2020, there were 11.71 million stroke cases worldwide, of which the incidence of ischemic stroke was 7.59 million [2]. Stroke is ranked as the second most prevalent factor contributing to disability, resulting in a loss of 143 million global disability-adjusted life-years (DALYs) in 2019, of which population attributable fraction (PAF) in AIS is 85.7% [3].
Endovascular recanalization remains the primary approach for AIS, encompassing balloon angioplasty, stent implantation, intraarterial thrombolysis and thrombectomy upto a 24-hour window for patients with disabling AIS. Thrombolysis patients were more likely to have a favorable outcome with modified Rankin Scale (mRS) score less than 3 or no or minimal disability at 3 months than placebo [4]. A meta-analysis of 8 randomized controlled trials showed that mechanical thrombectomy had a better outcome than thrombolysis at 90 days [5]. Nowadays, thrombolysis and/or mechanical thrombectomy are the first choice for AIS patients. Furthermore, a subset of clinical investigations and animal trials have demonstrated that protracted recanalization therapy (lasting over 24 h or even approaching a month) confers benefits to patients, and the relevant mechanisms need further exploration [6, 7].
Hemorrhagic transformation (HT) refers to hemorrhagic infarction subsequent to arterial thrombosis and embolism. Autopsy studies have reported an HT rate of 18–42% in AIS [8] and ischemia/reperfusion (I/R) injury caused by thrombolysis and mechanical thrombectomy leads to a further increase in the incidence of HT [9]. To enhance the efficacy of vascular recanalization, it is imperative to investigate neuroprotective interventions aimed at mitigating HT. This article comprehensively reviews the underlying mechanisms, current challenges, existing therapies, and potential therapeutic targets for neuroprotective treatment for AIS.
Pathophysiologic mechanism of HT in AIS: disruption of blood-brain barrier and neurovascular unit
HT in AIS mainly arises from disruption of the blood-brain barrier (BBB) and neurovascular unit (NVU) in AIS [8].
BBB, composed of brain endothelial cells, astrocytes, pericytes, neurons, supporting structures (microglia, oligodendrocytes), and extracellular matrix (ECM), acts as a selective barrier regulating the exchange of substances between the bloodstream and brain parenchyma while preventing the entry of harmful substances [10]. NVU encompasses the BBB along with neurons and the surrounding ECM [11], the definition of which was proposed by the Stroke Progress Review Group meeting of the National Institute of Neurological Disorders and Stroke in 2001, emphasizing the relationship between the brain and vessels [12]. During AIS, an ischemic cascade occurs, involving 1) deprivation of oxygen and glucose leading to the release of glutamate, which triggers excitotoxic signaling pathways within neurons; 2) the release of proinflammatory cytokines exacerbating neuronal injury; 3) the migration of leukocytes to the ischemic area promoting to further release of inflammatory cytokines (Figure 1) [13]. These can lead to damage to the BBB. The inflammatory factors generated in this process will further lead to the destruction of NVU. Therefore, glutamate and inflammatory factor storm at the infarct site can be targets of neuroprotective therapy. Furthermore, matrix metalloproteinases (MMPs) are enzymes that degrade protein molecules in ECM and crucial molecules in the pathophysiologic process of disruption of BBB. MMP-2, MMP-3, and MMP-9 [8, 14] have been confirmed to be involved in the destruction of BBB and NVU. The involvement of MMPs in the mechanism of hemorrhagic retransformation makes them a promising therapeutic target.
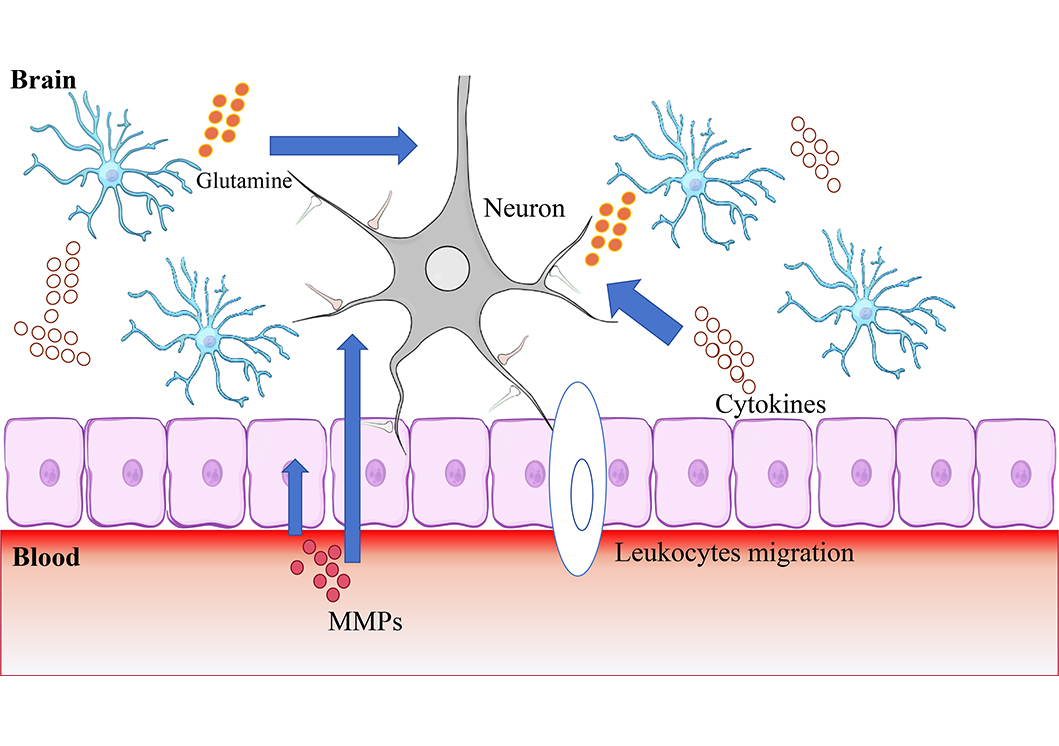
This figure shows important mechanisms of HT: 1) Deprivation of oxygen and glucose leading to the release of glutamate, which triggers excitotoxic signaling pathways within neurons; 2) the release of proinflammatory cytokines exacerbating neuronal injury; 3) the migration of leukocytes to the ischemic area promoting to further release of inflammatory cytokines. This suggests that glutamate, MMPs, oxidative stress, and inflammatory storm are the targets of neuroprotective therapies
Note. Neuron schematic reprinted from scidraw.io (https://doi.org/10.5281/zenodo.3925929). CC BY. Epithelial layer schematic reprinted from scidraw.io (https://doi.org/10.5281/zenodo.3926185) by Chilton J. CC BY. Astrocyte schematic reprinted from scidraw.io (https://doi.org/10.5281/zenodo.3926048) by Chilton J. CC BY.
Pathophysiologic mechanism of I/R injuries after recanalization therapy
Vascular recanalization therapy can destroy BBB and NVU due to the pathophysiologic process of I/R injury, leading to an increased incidence of HT. It needs to be acknowledged that even in the presence of I/R injury, patients may benefit more from recanalization than from cerebral infarction [4]. However, it remains crucial to safeguard brain cells against I/R injury. I/R injury is associated with mitochondrial dysfunction, calcium overload, excitotoxicity, oxidative stress, programmed cell death, and neuroinflammation [15]. Those pathophysiologic mechanisms are the current focus of neuroprotective treatment.
Mitochondrial dysfunction is one of the most important hallmarks in the pathophysiologic process of ischemic stroke [16]. Triggered by mitochondrial oxidative stress, Ca2+ overload, dysregulation of iron homeostasis, mitochondrial DNA defects, and disruption of mitochondrial quality control (MQC), programmed cell death subsequently ensues encompassing apoptosis, pyroptosis, necroptosis, and ferroptosis [17]. MQC is a critical process to maintain mitochondrial health and function. A key aspect of MQC is mitophagy mediated by mitochondria to remove inactivated or damaged mitochondria [18]. While it scavenges damaged mitochondria, effectively enhancing mitophagy can timely eliminate damaged mitochondria thereby preventing programmed cell death and reducing I/R damage [19].
Neuroinflammation represents an autonomous pathophysiologic process that detrimentally affects the integrity of both BBB and NVU. The cardinal feature of neuroinflammation is release of cytokines and chemokines by activated microglia, endothelial cells, and astrocytes, which inflict damage upon the BBB and augment its permeability. That process will allow monocytes and macrophages to migrate across the endothelium [20]. As a result, targeted therapeutic interventions aimed at mitigating microglia activation, macrophage infiltration, inflammatory mediators, and cytokines production are promising for developing neuroprotective agents.
The assessment of microcirculation dysfunction after recanalization warrants attention. Animal experiments have suggested that I/R injury may lead to pericyte impairment, consequently impeding microcirculatory restoration and even resulting in the absence of reflow phenomenon [21, 22]. Therefore, targeting the restoration of pericyte function and promoting microvascular patency are promising for future neuroprotective strategies [23].
Obstacles to investigating neuroprotective therapy
Although preclinical research on neuroprotective therapies is abundant, it remains challenging to translate these findings into clinical applications. In the Stroke Treatment Academic Industry Roundtable (STAIR) conference, the failure of clinical translation of neuroprotective agents has been attributed to the following reasons.
Firstly, the research of neuroprotective treatment needs to change from neuron protection to brain protection. Different cells in the NVU respond differently to different stimuli, while researchers may assume that all components of the NVU respond similarly when applying brain protective agents. This may partly explain the failure of clinical applications of neuroprotective therapies [24]. Therefore, during the STAIR X meeting, it was proposed to adopt the concept of brain protection instead of solely focusing on neuroprotection. In the STAIR XI meeting this point was made more explicit and recommended to be taken into account in the design of preclinical studies [25, 26].
Secondly, neuroprotective treatment should choose a multi-target and multi-pathway treatment and involve all cells of NVU including neurons involved in the ischemic cascade [24].
Additionally, new human-matched animal models are needed for preclinical studies. Most existing animal models, particularly rodents, differ from humans in terms of age, thrombosis mode, vascular anatomy, living environment, etc. [27]. Therefore, it is crucial to modify animal models and utilize those that closely mimic the internal environment of humans. Under the existing circumstances, researchers have proposed several strategies. In the beginning, researchers tried to use large mammals instead of the mouse model due to their resemblance to humans. However, practical considerations and ethical issues significantly hinder their use in experimental studies [28]. In a preclinical investigation of the neuroprotective agent NXY-059, middle cerebral artery (MCA) embolization was implemented to instigate stroke in monkeys, yielding encouraging outcomes [29]. In large-scale clinical trials of NXY-059, this neuroprotective agent demonstrated no notable efficacy in humans [30]. Thus, it becomes evident that even primates, beings closely similar to humans, do not entirely replicate the human brain environment. This may be due to the differences in anatomical structures and the incongruence between surgery-induced stroke and common stroke etiologies. Furthermore, the procedure involved invasive measures that caused pain and stress to the animal [28]. Currently, an increasing number of animal models pertinent to AIS are being investigated. Wang et al. [31] successfully established three-dimensional cerebral organoids from human pluripotent stem cells, which demonstrated similar effects as traditional models. They evaluated this new model using recognized neuroprotective drugs such as butylphthalide and edaravone. However, the absence of NVU, BBB, and immune cells in cerebral organoids poses a significant hindrance to the application of this model. Consequently, it is imperative to explore novel human models for AIS.
Promising neuroprotectants and neuroprotective therapy in AIS
3-N-Butylphthalide
In 2002, the China Food and Drug Administration granted approval for the use of 3-N-Butylphthalide (NBP) in treating ischemic stroke, which consists of optical isomers l-NBP, dl-NBP, and d-NBP, which is isolated from seeds of Apium graveolens (Chinese celery). The effectiveness of butylphthalide has been validated through various clinical trials and animal experiments in alleviating neuroinflammation, preserving mitochondrial function, mitigating oxidative stress, and ameliorating BBB impairment [32].
Yan et al. [33] found NBP significantly reduced cerebral infarction size, cell apoptosis, BBB damage, and brain edema in mice as a neuroprotective role with I/R injury of MCA occlusion (MCAO) preconditioned. Many researchers have investigated the pathways involved in the neuroprotective effects of NBP. Huang et al. [34] suggested that early application of butylphthalide alleviated cerebral I/R injury by inhibiting mitochondrial high-temperature requirement A2 (HtrA2/Omi)-mediated apoptosis in MCAO rats. Zhan et al. [35] proved that NBP exerted a neuroprotective effect on MCAO mice by up-regulating miR-21 level to inhibit neuronal apoptosis and reactive oxygen species (ROS) production as well as improve cell proliferation activity, which may be associated with the inhibition of Toll-like receptor 4/nuclear factor-kappa B (TLR4/NF-κB) pathway. NBP also plays a neuroprotective role in clinical studies, improving functional outcomes in patients with AIS. Wang et al. [36] conducted a randomized controlled clinical trial among patients with AIS receiving intravenous thrombolysis or endovascular therapy. In that study, NBP was associated with a higher percentage of patients with favorable functional outcomes at 90 days compared with placebo. In summary, the neuroprotective effect of NBP on AIS has been extensively confirmed in both animal and human, making it widely used in clinical practice.
Edaravone dexborneol
Edaravone, a low molecular-weighted free radical scavenger, can readily traverse the BBB and effectively reduce the production of oxygen free radicals while modulating additional deleterious cascades, such as inhibition of lipid oxidation, inhibition of endothelial nitric oxide synthase, and activation of MMP-9 [37, 38]. The neuroprotective effects of edaravone are shown in the meta-analysis of randomized controlled trials and prospective cohorts on edaravone [39]. Nowadays, an edaravone-containing compound drug, edaravone dexborneol, has gained widespread utility in animal and clinical studies. Wu et al. [40] first combined borneol, an anti-inflammatory drug, with edaravone in a rat model of transient cerebral ischemia-reperfusion. They discovered that this combination exhibited a treatment time window of 6 h and significantly improved the damage in a permanent cerebral ischemia-reperfusion model. In terms of the study of molecular pathways, Hu et al. [41] showed that edaravone dexborneol effectively suppressed nucleotide-binding oligomerization domain-like receptor pyrin domain-containing protein 3 (NLRP3) inflammation-induced activation of microglial pyroptosis in experimental ischemic stroke through NF-κB/NLRP3/gasdermin D (GSDMD) signaling pathway using transient MCAO (tMCAO) mouse model in vivo and oxygen-glucose deprivation (OGD) BV2 cell model in vitro. A multi-center, randomized, double-blind, controlled phase III trial, Thrombus Aspiration in ST-Elevation Myocardial Infarction in Scandinavia (TASTE) trial [42], enrolled 1,165 AIS patients who were randomly assigned to receive either edaravone dexborneol or edaravone. The proportion of patients with good functional outcomes at 90 days was significantly higher in the edaravone dexborneol group than in the edaravone group [mRS score ≤ 1, 67.18% vs. 58.97%; odds ratio (OR): 1.42; 95% confidence interval (CI): 1.12 to 1.81; P = 0.004]. Therefore, the neuroprotective effect of edaravone dexborneol is more worthy of promotion than edaravone in clinical trials. TASTE-III, a randomized, controlled trial has been completed evaluating the efficacy and safety of edaravone dexborneol in patients with AIS undergoing reperfusion therapy, and the results are pending. In addition to edaravone dexborneol, oral administration of edaravone [43] and edaravone ionic liquid [44] have also been proven to have good anti-oxidative free radicals and reduce I/R injury in animal experiments. In conclusion, edaravone dexborneol is a neuroprotective agent with relatively sufficient clinical research data.
Agents associated with glucose metabolism—glucagon-like peptide-1 and insulin-like growth factor-1
Glucagon-like peptide-1 (GLP-1) is a multifunctional polypeptide that activates GLP-1 receptor (GLP-1R), playing a crucial role in neuroprotection [45]. Numerous animal studies have confirmed the significance of GLP-1/GLP-1R as key molecules for neuroprotection, particularly beneficial for AIS patients with diabetes. The neuroprotective effects of GLP-1/GLP-1R primarily involve reducing neuroinflammation, and MMP-9 to reduce BBB damage, oxidative stress, cell excitability, cell apoptosis, and pyroptosis [46–48].
Insulin-like growth factor-1 (IGF-1) induces various neuroprotective activities through the activation of phosphoinositide 3-kinases/protein kinase B/mammalian target of rapamycin (PI3K/Akt/mTOR) and mitogen-activated protein kinase (MAPK) pathways [49, 50]. MicroRNA (miRNA) analysis conducted by Bake et al. [51] revealed that IGF-1 significantly downregulated the PI3K/Akt signaling pathway, cell adhesion/endothelial stromal cell receptor pathway, T and B cell signaling pathway, and cytokines storm and inflammatory factors. This implies that IGF-1 exerts neuroprotective effects mainly by protecting the BBB and inhibiting neuroinflammation. The above are neuroprotective drugs related to glucose metabolism.
The two categories of medications are extensively utilized in clinical practice, particularly for individuals diagnosed with diabetes. Although no randomized clinical controlled trials investigating the aforementioned drugs’ neuroprotective effects have been officially published yet, we anticipate their promising prospects for AIS patients in future research.
Traditional Chinese medicine
Traditional Chinese medicine (TCM), derived from natural extracts and analogs of herbs, is now widely employed in animal studies, clinical research, and clinical practice of AIS due to its multi-target, multi-pathway, and wide range of effects containing anti-oxidative, anti-apoptotic, anti-neuroinflammatory, and neuromodulatory effects [10, 52]. A review conducted in 2022 on the neuroprotective effects of TCM [53] classified the natural compounds of TCM into glycosides (astragaloside IV, gastrodin, ginsenoside Rg1, and salidroside), flavonoids (baicalin, icariin, puerarin, and breviscapine), phenols (resveratrol, curcumin, and salvianolic acid B), and terpenes (ginkgolide B and catalpol). The targets of TCM are numerous and not fully elucidated. In preclinical studies, a natural compound can exert its neuroprotective effects through different mechanisms. For instance, astragaloside IV, a natural compound extracted from Astragalus, showed neuroprotective effects through calcium-sensing-receptor-mediated apoptosis. Apart from these natural compounds, we can also see the neuroprotective effects of many TCM prescriptions, such as Tongxinluo [54], XingNaoJing [55], Gualou Guizhi decoction [56], Tao Hong Si Wu decoction [57], etc. in those preclinical studies. In clinical trials, Zhang et al. [58] conducted a randomized clinical trial to validate the efficacy and safety of ginkgo diterpene lactone. In conclusion, TCM still has great therapeutic potential. Further exploration regarding their molecular mechanisms is challenging.
Nerinetide
Nerinetide (NA-1) is a polypeptide that interferes with postsynaptic density protein 95 (PSD-95) and reduces the interaction between PSD-95 and neuronal nitric oxide synthase, thereby reducing nitric oxide radical production and its mediated cell death [59]. In the preclinical model of ischemic stroke, it has shown promise in reducing infarct size during cerebral ischemia-reperfusion and improving functional prognosis [60]. However, in a large randomized controlled trial (ESCAPE-NA1) [61] published in 2020, the rate of favorable functional outcome at 90 days in patients undergoing endovascular thrombectomy was not significantly different with NA-1 compared with the control group, which represented a failure of clinical translation. In the case of utilizing experimental models similar to humans in preclinical research, the failure of clinical translation not only indicates that we need to find a preclinical model that is more suitable for humans but also motivates us to look for multi-target and multi-pathway neuroprotective drugs. Previous observational studies have demonstrated a positive association between NA-1 and favorable patient outcomes in those not receiving recombinant tissue-type plasminogen activator (rt-PA) [61], thus highlighting the need for further clinical investigations on NA-1.
Potential therapeutic agents targeting NVU
As mentioned above, MMPs are a class of molecules associated with BBB damage. Consequently, anti-MMP molecules have emerged as an important therapeutic strategy for combating hemorrhagic retransformation in AIS. Ji et al. [62] explored the therapeutic potential of an immunoglobulin G (IgG) monoclonal antibody with neutralizing specificity for MMP-9, which significantly attenuated BBB disruption in the stroke model.
Osteopontin is another molecule that has been identified in NVU studies. Spitzer et al. [63] established an NVU transcript database and found that osteopontin was highly upregulated in all NVU cell types at 24 h after ischemic stroke. Targeted therapy with subcutaneous injection of anti-osteopontin antibody could neutralize the expression of osteopontin in NVU cells after ischemic stroke in mice, thereby ensuring the integrity of the NVU and reducing the risk of hemorrhagic retransformation. The aforementioned two agents are currently undergoing preclinical investigations exclusively, with a specific focus on the NVU, exhibiting substantial therapeutic potential as neuroprotective agents.
Ferrostatin-1
Ferroptosis has recently been increasingly recognized as an important mechanism of pathological cell death during stroke. Ferrostatin-1 [64] and its analog, Srs11-92 [65], can improve oxidative stress and neuroinflammation following cerebral I/R injury, potentially serving as novel targets for neuroprotection.
Sevoflurane
Sevoflurane, one of the most commonly used anesthetics, exerts a protective effect on cerebral I/R injury [66]. Currently, the research on sevoflurane is mainly reflected in the control of neuroinflammation and the inhibition of apoptosis. Yang et al. [67] demonstrated that sevoflurane can reduce neuronal apoptosis by mediating the E2F transcription factor 1/enhancer of zeste homolog 2/tissue inhibitor of metalloproteinases 2 (E2F1/EZH2/TIMP2) regulation axis, thereby protecting rats from brain I/R injury. Lyu et al. [66] found that sevoflurane could inhibit ferroptosis of cells through the specificity protein 1/acyl-CoA synthetase long chain family member 4 (SP1/ACSL4)-pathway.
Clomethiazole
Clomethiazole, derived from thiazole moiety of thiamine, exerts its neuroprotective effects by activating gamma-aminobutyric acid (GABA) receptors [68]. In the clomethiazole acute stroke study (CLASS), a randomized, controlled trial of clomethiazole in 1,360 acute stroke patients, its efficacy was not significantly different from that of the placebo group [69]. Nevertheless, in the subgroup analysis of large stroke, a treatment effect was observed with clomethiazole, as reflected in an increase of Barthel index in proportion to 40.8%, with a relative benefit of 37% compared with placebo [70]. And it shows good safety in clinical application [71].
Substance P
Substance P, a neuropeptide consisting of 11 amino acids, has been demonstrated to promote the peripheral mobilization of bone marrow mesenchymal stem cells (MSCs) and monocyte/macrophage polarization in various acute and chronic tissue injuries. This peptide has exhibited promising neuroprotective effects in animal studies. Using a rat model of AIS induced by tMCAO, our research team observed that rats treated with substance P exhibited a 3-fold reduction in mortality rates and a 40% decrease in cerebral infarct volumes compared to those administered saline. Furthermore, substance P augmented the expression of neuroprotective and anti-inflammatory genes in the ischemic brain, and induced neuronal survival in the brain penumbra. This led to the preservation of the BBB in ischemic brain tissue [72]. More clinical studies are needed to further explore the neuroprotective effect of substance P.
Stem cells
Stem cells possess the ability to regenerate into new cells and differentiate into different cells. In neuroprotective therapy, they not only have the potential to replace damaged cells and tissues but also create a supportive microenvironment for neurogenesis, release of neurotrophic factors, and restoration of mitochondrial function [73]. Recent studies have focused on different types of stem cells including MSCs, neural stem cells (NSCs), and hair follicle stem cells. Among these, MSCs have been extensively investigated in preclinical and clinical research due to their capacity to mitigate oxidative stress, produce cytokines and extracellular vesicles that inhibit proinflammatory cytokines and inflammatory cell activation, suppress pyroptosis, as well as reduce BBB permeability [74]. Ha et al. [75] verified that adult human NSCs can significantly reduce tissue and neural function loss, which is mediated through its paracrine neuroprotective and proangiogenic activities through the Janus kinase 2/signal transducer and activator of transcription 3 (JAK2/STAT3) signaling pathway. In a 2023 preclinical study, Tang et al. [76] verified for the first time that hepatocyte growth factor-modified hair follicle stem cells could alleviate I/R injury and promote neurological function recovery by inhibiting inflammatory response, protecting the BBB, and promoting angiogenesis. At present, the sample size of patients in the clinical research of stem cells is small, and the clinical evidence is insufficient. For MSCs, there is a randomized controlled trial including twenty patients presenting AIS, which suggested fewer neurological complications, including cerebral edema and hemorrhagic retransformation [77]. For NSCs, an open-label, single-center, dose-escalation study was conducted and eleven men with chronic ischemia stroke were included, in which NSCs were found to cause no adverse events and were associated with improvement in neurological function [78]. Moreover, further investigation is required regarding immune responses induced by stem cell transplantation as well as optimal delivery methods for effective application [73, 79, 80]. Nonetheless, stem cell therapy holds promise as a potential future direction for neuroprotective treatment in AIS.
miRNA
miRNA is a class of small non-coding RNA molecules, ranging in length from 19 to 24 nucleotides, which plays a key role in biological processes by regulating the expression of messenger RNA. Numerous studies have demonstrated alterations in miRNA expression following AIS, suggesting their potential involvement in the pathophysiologic mechanisms underlying this condition. Modulating miRNA regulation may offer protective effects on the BBB and mitigate I/R injury [81].
The present study, as depicted in Table 1, provides a comprehensive overview of miRNA investigations conducted within the past three years. Notably, all these studies are preclinical and involve experimental animal models, encompassing diverse pathways and targets. However, as their role in the psychopathology of AIS is uncertain, the development of neuroprotective agents against them is currently not a priority. Further exploration through both preclinical and clinical trials is needed to fully elucidate the potential of miRNA as a neuroprotective therapeutic strategy.
Recent miRNA-related studies in AIS
Types | Year | Abstract | Reference |
---|---|---|---|
miR-20a-3p | 2022 | Identify the neuroprotective effect of miR-20a-3p based on the astrocytes | [82] |
miR-126a-5p | 2021 | miR-126a-5p is a novel potential target for ischemic stroke therapy due to its protection against cerebral I/R injury via directly targeting nicotinamide adenine dinucleotide phosphate oxidase 2 (NOX2) | [83] |
miR-190 | 2021 | miR-190 confers protection against brain I/R injury by modulating Rho/Rho-kinase signaling | [84] |
miR-30c | 2020 | miR-30c can improve the pathological damage of rats with I/R injury and plays a neuroprotective role both in vivo and in vitro by targeting SRY-box transcription factor 9 (SOX9) | [85] |
miR-22 | 2020 | miR-22 exerts its neuroprotective and angiogenic functions via the PI3K/Akt signaling pathway, at least partly, in rats under cerebral ischemia-reperfusion | [86] |
miR-1202 | 2020 | miR-1202 exerts a neuroprotective effect by negatively regulating its target protein Rab1a, which can inactivate TLR4/NF-κB-involved inflammatory signaling pathway in OGD/reoxygenation (R) induced human microglia (HM) cells | [87] |
miR-532-5p | 2020 | These findings reveal that miR-532-5p protects against ischemic stroke by inhibiting phosphatase and tensin homolog (PTEN) and activating the PI3K/Akt signaling pathway stroke | [88] |
miR-18b | 2020 | These data indicate that miR-18b protects against cerebral I/R injury by inhibiting annexin A3 (ANXA3) and activating PI3K/Akt pathway | [89] |
miR-219a-5p | 2020 | Upregulation of miR-219a-5p decreases cerebral I/R injury by targeting phosphodiesterase 4D (Pde4d) in vitro | [90] |
miR-193b-3p | 2020 | miR-193b-3p has a potentially neuroprotective effect on focal cerebral I/R injury by inhibiting 5-lipoxygenase (5-LOX) expression | [91] |
Physical therapy
In addition to neuroprotective drugs and molecules, many physical therapies have shown neuroprotective effects in AIS patients through preclinical and clinical studies.
Therapeutic hypothermia
Therapeutic hypothermia (TH) is one of them. Kim et al. [92] utilized a mouse model of stroke and reperfusion to propose that TH at temperatures of 33°C or 36°C exhibited neuroprotective properties by mitigating cell death and modulating microglial activation and polarization. Similarly, Hong et al. [93] conducted an open-label prospective study involving 75 patients with AIS in the anterior circulation who achieved successful recanalization. Patients who received a mild hypothermia regimen were compared with guideline-directed care without hypothermia. There was less cerebral edema and HT in the mild hypothermia group, thus suggesting a correlation between TH and clinical outcomes in AIS. This clinical study, although prospective, is not a randomized controlled trial. In conclusion, TH has been found to have neuroprotective effects in animal experiments and is also relevant to neuroprotection in clinical practice. However, there remains a dearth of reliable clinical trials, and the optimal approach for implementing systemic or local hypothermia remains uncertain [94].
Electroacupuncture
Electroacupuncture (Ea) is a kind of auxiliary treatment of TCM through electrical stimulation of acupoints. Many preclinical studies have indicated that Ea exhibits neuroprotective effects by anti-apoptosis and reduction of neuroinflammation, mediated by the p38 MAPK pathway, TLR4/NF-κB pathway, and PI3K/Akt pathway [95–99]. Currently, the majority of studies on Ea primarily consist of preclinical research, with a limited number of clinical studies. However, there remains a lack of high-quality clinical research to bolster its credibility.
Oxygen therapy
Oxygen therapy is also one of the important treatments for neuroprotection. Qi et al. [100] established a rat model of I/R injury and subsequently administered normobaric hyperoxia as a therapeutic intervention. The rat model treated with normobaric hyperbaric oxygen maintained the homeostasis of glutathione peroxidase 4 and nicotinamide adenine dinucleotide phosphate (NADPH) oxidase 4 and the level of connexin43 in astrocytes, thereby preserving the stability of the BBB and safeguarding mitochondrial function.
In addition, studies on the neuroprotective effects of neuromodulation therapies are also ongoing. Some preclinical studies have shown that near-infrared ultrasound [89], transcranial direct current stimulation [101, 102], and low intensity pulsed ultrasound [103] are related to reducing ischemia-reperfusion and hemorrhagic retransformation and have a certain protective effect on AIS mice.
Transient ischemic attacks and remote ischemic conditioning
Transient ischemic attack (TIA), a transient impairment of brain or retinal nerve function resulting from localized ischemia, manifests as corresponding symptoms and signs at the site of ischemia. Some animal and clinical investigations imply that individuals with a history of TIA tend to exhibit superior functional outcomes post-stroke in comparison to those without any prior history of TIA. Tsai et al. [104] successfully developed a TIA mouse model and corroborated that the occurrence of TIA within 24 h was positively correlated with neurological recovery in rats. In a prospective study of 1,269 patients with nonlacunar stroke, 21.7% of those with a history of TIA had a favorable outcome, compared with 15% of those without a history of TIA [105]. The difference was statistically significant. The findings of this study imply that TIA might possess neuroprotective properties by eliciting ischemic tolerance. In another clinical study involving 887 AIS patients treated with endovascular thrombectomy, TIA occurring within 96 h before symptom onset was independently associated with 3-month functional outcomes but not with a reduction of severity of stroke [106]. In light of this, several researchers have endeavored to investigate the neuroprotective properties of ischemic preconditioning. Remote ischemic conditioning (RIC) refers to restricting blood flow into the limb and then releasing blood into the ischemic site to promote neuroprotection. The neuroprotective impact of RIC on stroke remains inconclusive in clinical practice [107]. To further explore this phenomenon, investigation into additional mechanisms and treatment methods is needed.
Annotations on neuroprotective agents
Given the limitations and complexity of stroke treatment, particularly drug delivery across the BBB, there is increasing interest in vectors that cross the BBB. Intranasal delivery of nanoparticles and apoptotic vesicles induced by stem cells is now under investigation to increase the dose of neuroprotective drugs across the BBB [108, 109].
Combining multiple neuroprotective drugs or treatments often yields superior outcomes compared to single-agent approaches. The challenging intracranial microenvironment during ischemia poses difficulties for the survival of MSCs. Li et al. [110] combined anti-inflammatory curcumin with MSCs in rats with I/R injury. The researchers demonstrated that this combined treatment mediated anti-inflammatory microglial polarization through the Akt/glycogen synthase kinase-3 beta (GSK-3β)/beta-transducin repeat containing protein (β-TrCP)/nuclear factor erythroid 2-related factor 2 (Nrf2) axis. The anti-inflammatory and anti-oxidative stress effects were significantly improved compared with a single drug, ultimately leading to improved neurological function following AIS (Figure 2).
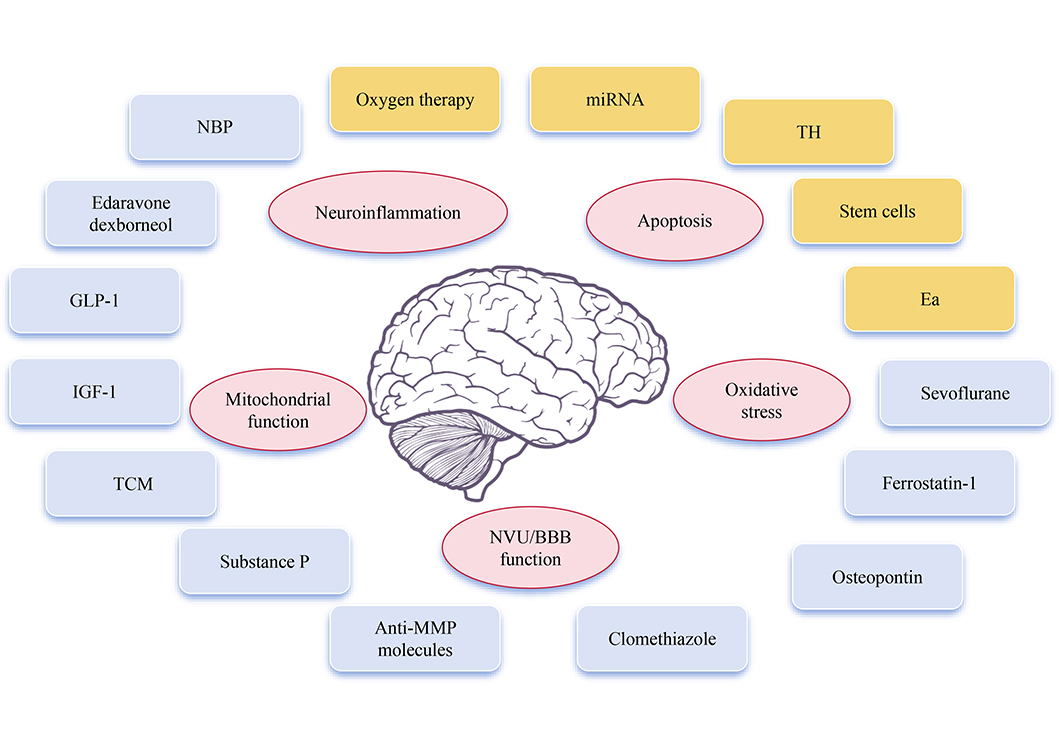
Promising neuroprotectants and neuroprotective therapy involved in AIS and its mechanism
Note. Brain schematic reprinted from scidraw.io (https://doi.org/10.5281/zenodo.3925989) by Chilton J. CC BY.
Limitations
This review has limitations. First, the evidence described in this article was current at the time of the search. Second, there are many types and mechanisms of neuroprotective therapies, and they are under investigation. This article cannot review all neuroprotective therapies. Third, some neuroprotective treatments lack higher-quality experimental and clinical evidence.
Conclusions
The neuroprotective treatment of AIS mainly targets ischemic cascades, such as mitochondrial dysfunction, oxidative stress injury, neuroinflammation, and programmed cell death processes including apoptosis, pyroptosis, and ferroptosis. To date, few neuroprotective agents have been used clinically due to the huge discrepancy between the results of animal studies and clinical trials. However, with further understanding of the pathophysiologic mechanisms underlying ischemic brain injury, combining neuroprotective methods and blood flow reconstruction, developing multi-targeted drugs, protecting various nerve cells in the NVU instead of single neuron protection, optimizing clinical trial protocols, and improving the efficiency of drug delivery into the central nervous system by biomaterials or stem cells, etc., will be the direction of future research on neuroprotective agents.
Abbreviations
AIS: |
acute ischemic stroke |
Akt: |
protein kinase B |
BBB: |
blood-brain barrier |
Ea: |
electroacupuncture |
ECM: |
extracellular matrix |
GLP-1: |
glucagon-like peptide-1 |
GLP-1R: |
glucagon-like peptide-1 receptor |
HT: |
hemorrhagic transformation |
I/R: |
ischemia/reperfusion |
IGF-1: |
insulin-like growth factor-1 |
MCAO: |
middle cerebral artery occlusion |
miRNA: |
microRNA |
MMPs: |
matrix metalloproteinases |
MQC: |
mitochondrial quality control |
MSCs: |
mesenchymal stem cells |
NA-1: |
nerinetide |
NBP: |
3-N-Butylphthalide |
NF-κB: |
nuclear factor-kappa B |
NSCs: |
neural stem cells |
NVU: |
neurovascular unit |
PI3K: |
phosphoinositide 3-kinases |
STAIR: |
Stroke Treatment Academic Industry Roundtable |
TCM: |
traditional Chinese medicine |
TH: |
therapeutic hypothermia |
TIA: |
transient ischemic attack |
TLR4: |
Toll-like receptor 4 |
Declarations
Acknowledgments
The authors would like to express their gratitude to the respective authors and scidraw.io for generously providing the original figure material in this article.
Author contributions
YY: Investigation, Writing—original draft. DG: Investigation, Writing—review & editing. Y Liu: Writing—review & editing, Validation, Supervision. Y Li: Conceptualization, Writing—review & editing, Validation, Supervision.
Conflicts of interest
The authors declare that they have no conflicts of interest.
Ethical approval
Not applicable.
Consent to participate
Not applicable.
Consent to publication
Not applicable.
Availability of data and materials
Not applicable.
Funding
Not applicable.
Copyright
© The Author(s) 2024.