Abstract
Neuroinflammation can be caused by disease, aging, infection, brain injury, toxicity, or stress. It is a contributory factor in the neuropathology of serious conditions that include multiple sclerosis (MS), Alzheimer’s disease, Parkinson’s disease, amyotrophic lateral sclerosis (ALS), and autoimmune encephalomyelitis (EAE). The neuroinflammatory response involves the activation of microglia, astrocytes, the endothelial cells of the blood-brain barrier, and peripherally-derived immune cells. The endocannabinoid system is composed of the natural cannabinoids, anandamide and 2-arachidonoyl glycerol (2-AG), enzymes regulating their synthesis/catabolism, and the cannabinoid CB1 and CB2 receptors. It regulates multiple systems in the body including inflammation and endocannabinoid system dysregulation is involved in numerous inflammatory conditions. The Cannabis sativa plant produces over 100 phytocannabinoids, some of which interact with the endocannabinoid system. The major phytocannabinoids are delta-9-tetrahydrocannabinol (delta-9-THC), cannabidiol (CBD), and cannabigerol (CBG). Compelling evidence is emerging that many phytocannabinoids have anti-inflammatory and antioxidant properties. Phytocannabinoids including delta-9-THC, CBD, and CBG bind to a wide variety of targets in the endocannabinoid and/or other systems, which probably accounts for their diversity of effects in non-clinical and clinical studies. The benefits of certain phytocannabinoids have been proven by regulatory approval for medical use of CBD (Epidiolex®), chemically synthesized delta-9-THC (Marinol® and Syndros®) and 1:1 delta-9-THC/CBD (Sativex®). Furthermore, the widely recognized therapeutic properties of Cannabis have been a key driver in legalizing the medical use of Cannabis in 38 USA states. In this review, the potential of phytocannabinoids as effective treatments in neuroinflammatory disorders is discussed based on a critical evaluation of the non-clinical and clinical evidence. We focused on delta-9-THC, CBD, and CBG because they are the most abundant phytocannabinoids in Cannabis sativa and a substantial body of scientific data exists to describe their respective pharmacological mechanisms.
Keywords
Phytocannabinoids, delta-9-tetrahydrocannabinol, cannabidiol, cannabigerol, neuroinflammation, antioxidant activity, neuroprotectionIntroduction
Neuroinflammation in the brain and spinal cord is a response, often with consequent pain, that develops from acute, chronic, or auto-inflammatory disorders and can lead to compromised organ function. Neuroinflammation may be caused by disease, ageing, infection (viral or microbial), brain or spinal cord injury, toxicity, or stress. Types of diseases involving neuroinflammation include multiple sclerosis (MS), Alzheimer’s disease [1, 2], Parkinson’s disease, amyotrophic lateral sclerosis (ALS), and autoimmune encephalomyelitis (EAE) [3].
The neuroinflammatory response involves the activation of microglia, astrocytes, endothelial cells of the blood-brain barrier, and peripherally-derived immune cells [4, 5]. Microglia play a key role in the mediation of neuroinflammatory responses. They carry out primary immune surveillance functions and macrophage-like activities of the CNS, which include the production of cytokines [e.g., interleukin-1β (IL-1β), IL-6, and tumor necrosis factor-α (TNF-α)], secondary messengers (nitric oxide and prostaglandins) and reactive oxygen species [5]. Cytokines are small proteins important in cell signalling and they have been shown to be immunomodulatory agents. Cytokines include chemokines, interferons, ILs, lymphokines, and TNFs, and they are produced by numerous types of cells, such as immune cells [3].
The endocannabinoid system is involved in the modulation of systems in the body including those for cognition, anxiety, neurogenesis, pain sensation, immune system activity, and inflammation [6, 7]. Dysregulation of this system is implicated in various inflammatory conditions. The brain and peripheral nervous systems of humans and many animal species synthesize various endocannabinoid molecules which act as neurotransmitters (Figure 1).
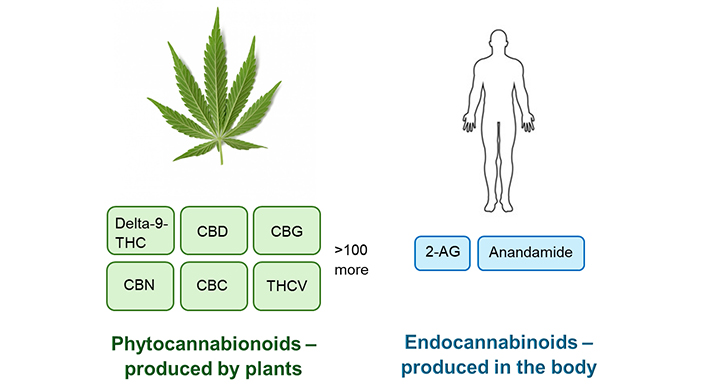
Phytocannabinoids and endocannabinoids. 2-AG: 2-arachidonoyl glycerol; CBC: cannabichromene; CBD: cannabidiol; CBG: cannabigerol; CBN: cannabinol; delta-9-THC: delta-9-tetrahydrocannabinol; THCV: delta-9-tetrahydrocannabivarin
The endocannabinoid system comprises (i) the endocannabinoids [natural cannabinoid substances in the body including 2-AG and arachidonoyl ethanolamide (anandamide)], (ii) enzymes involved in endocannabinoid synthesis and catabolism that regulate their concentration in the brain, and (iii) the cannabinoid receptor types 1 and 2 (CB1 and CB2) which transmit their signals in tissues and organs (Figure 2) [8]. The CB1 receptor is located in the brain and in various peripheral tissues, while the CB2 receptor is predominantly located in the periphery on immune cells, as well as in the CNS, mostly on microglia, and at low levels in the brainstem [9, 10]. Although the CB1 receptor is located on immune cells, the endocannabinoid system primarily regulates inflammation via activation of CB2 receptors. CB2 receptor stimulation controls oxidative stress processes and decreases neuroinflammation via their activation in microglia leading to the release of anti-inflammatory molecules [11].
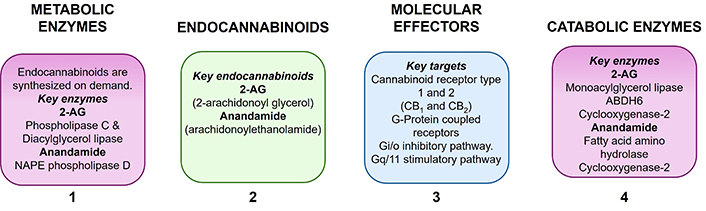
Components of the endocannabinoid system. 2-AG: 2-arachidonoyl glycerol; ABDH6: alpha/beta-hydrolase domain containing 6; NAPE phospholipase D: N-arachidonoyl phosphatidyl ethanolamine phospholipase D
The Cannabis sativa plant produces phytocannabinoids that can interact with the endocannabinoid systems of humans and animals. Cannabis plants contain over 100 phytocannabinoids, the major ones being delta-9-tetrahydrocannabinol (delta-9-THC), cannabidiol (CBD), and cannabigerol (CBG). Other cannabinoids include cannabidivarin (CBDV), delta-9-tetrahydrocannabivarin (THCV), cannabichromene (CBC), delta-8-THC, and cannabinol (CBN) (Figure 1). Delta-9-THC is the major psychoactive (mind-altering) component of the Cannabis plant with the minor cannabinoids, THCV, CBN, and delta-8-THC also having psychoactive properties, albeit much weaker. CBD, CBG, and CBDV are not psychoactive. Delta-9-THC produces its intoxicating, emotional, perceptual, and hedonic effects (“high”) by activating the CB1 receptor subtype in the CNS. This action is also responsible for delta-9-THC’s adverse impact on attention, learning, memory, motor (movement), and cognitive performance. Delta-9-THC and CBD are the most abundant cannabinoids in the Cannabis sativa plant, whereas CBG (the direct biological precursor to delta-9-THC and CBD) is present at lower levels in the plant biomass. The levels of individual cannabinoids can, however, vary considerably and this is dependent on the strain and age of the plants. Hemp is one type of Cannabis plant species that is characterized by low delta-9-THC content, less than 0.3% as defined by USA federal law [8, 12].
Increasing evidence is emerging that phytocannabinoids have anti-inflammatory and antioxidant properties. Scientific reports suggest that phytocannabinoids including, delta-9-THC, CBD, and CBG bind to many physiological targets in the endocannabinoid system, and/or other systems, possibly explaining the multiple effects reported in non-clinical and clinical studies that will be discussed in this review. In addition to the cannabinoid CB1 and CB2 receptors, phytocannabinoids also modulate non-cannabinoid receptors including the transient receptor potential vanilloid (TRPV), TRP ankyrin (TRPA), and TRP melastatin (TRPM) subfamilies, the G55 protein-coupled receptor (GPR55), voltage-gated calcium and sodium ion channels and nuclear receptor transcription factors, i.e., nuclear factor kappa-B (NF-κB), and peroxisome proliferator-activated receptor alpha (PPARα) and gamma (PPARγ) [13, 14]. TRP ligand-gated cation channels are found in many species and they are necessary for the transduction of sensory stimuli, including those that mediate noxious thermal and mechanical stimuli. Six TRP channels from three subfamilies mediate cannabinoid activity: TRPV1, TRPV2, TRPV3, TRPV4, TRPA1, and TRPM8 [15]. GPR55 is a lysophosphatidylinositol-sensitive receptor, which also mediates cannabinoid signalling and it has been suggested that GPR55 is the third cannabinoid receptor subtype. GPR55 receptors are ubiquitously located in the CNS including the brainstem area and GPR55 signalling is a key element in the descending pain control pathway and is involved in mechanical hyperalgesia that is associated with inflammatory and neuropathic pain [16]. Voltage-gated ion channels are integral membrane proteins that have an important function in the electrical signalling of excitable cells like neurons by the production of action potentials and release of neurotransmitters. The transcription factor, NF-κB, is a regulator of immune functions and is a primary inflammatory response mediator. NF-κB initiates the expression of pro-inflammatory genes, including those responsible for the production of cytokines and chemokines. In vertebrates, the innate (non-specific) immune system is one of the two main immunity systems, the second is the adaptive immune system. NF-κB is critical for regulating the survival, activation, and differentiation of innate immune cells and inflammatory T-cells [17]. PPARα and PPARγ are members of the nuclear receptor superfamily of transcription factors. They are important for regulating a diverse array of physiological processes including inflammation [14].
With regard to the receptor binding activity of individual phytocannabinoids, delta-9-THC is a high-affinity partial agonist at CB1 and CB2 receptors and also has an affinity for TRP channels, TRPV type-2 (TRPV2) channels and calcium ion channels. The form of CBD isolated from plants [(–)-CBD] has very low affinity (only micromolar range) for the CB1 receptor or CB2 receptor. Unlike delta-9-THC, CBD does not activate CB1 receptors but may act as a negative allosteric modulator at this receptor subtype. At CB2 receptors, CBD is a partial agonist. CBG has weak partial agonist activity at CB1 and CB2 receptors and also binds to TRPV channels, PPARγ, and other targets [18]. CBDV is an agonist of TRPA type 1 (TRPA1) channels, a moderate agonist at the TRPV type-1 (TRPV1) channel, and an antagonist at the TRPM8 channel. CBC is an agonist at CB2 receptors and TRP channels, which has potent activity at TRPA1 channels. CBN is a low-affinity partial agonist at CB1 receptors and CB2 receptors, a low-affinity agonist at TRPV and TRPA1 channels, with antagonist activity at TRPM channels [13, 19].
In this review, we assess the evidence that phytocannabinoids have the potential to be efficacious treatments in neuroinflammatory disorders and their proposed modes of action. We have focused on delta-9-THC, CBD, and CBG because they are the most abundant phytocannabinoids in the Cannabis plant and there is substantial scientific data describing their respective pharmacological mechanisms. This review evaluates and summarizes the non-clinical and clinical evidence describing the properties of these phytocannabinoids and their potential as treatments for neuroinflammatory diseases.
Non-clinical evidence for phytocannabinoid modulation of neuroinflammation and antioxidant activity
Models of inflammation and pain
Delta-9-THC treatment reduces proinflammatory cytokine secretion, including IFN-γ and TNF-α, and suppresses the proliferation of T cells through apoptosis and/or induction of regulatory immune cells [20]. In a model of delayed-type hypersensitivity in mice, delta-9-THC (20 mg/kg) was reported to decrease tissue swelling and immune cell infiltration and decrease lymphocyte activation at the site of an antigen challenge in the footpad. Inhibition of the spontaneous secretion of IFN-γ, TNF-α, and IL-17 was noted in delta-9-THC-treated mice compared with the control group [21].
Adenosine agonists have been demonstrated to have anti-inflammatory effects. One study administered a low dose of CBD to LPS-treated mice and reported decreased TNF-α production in mouse serum [22]. This effect was reversed by the administration of an adenosine A2A receptor antagonist and was abolished in the adenosine A2A receptor knockout mouse, showing that CBD produced its anti-inflammatory effect by enhancing adenosine signalling.
Recently, Karuppagounder et al. [23] evaluated the effects of CBD oil or CBG oil on pain and disease progression in a model of osteoarthritis in mice (surgical destabilization of the medial meniscus). The mice were administered vehicle, CBD oil, or CBG oil. CBD oil and CBG oil treatments improved the impaired gait of the mice over the whole of the disease course (weeks 2 to 8 post-surgery). Mechanical allodynia (pain resulting from a stimulus that would not normally cause pain) in the mice was not ameliorated by either CBD or CBG oils. However, CBD oil and CBG oil reduced cold-induced allodynia. In the open-field test, both CBD and CBG treatments normalized locomotor activity changes in the mice. The CBD oil and CBG oil treatments also resulted in decreased synovitis. CBG oil alone decreased cartilage degeneration, chondrocyte loss, and matrix metalloproteinase-13 expression, together with increased anabolic chondrocyte numbers. Subchondral bone remodelling in the control group of mice was not improved by either the CBD or CBG oils. These findings are supportive of the beneficial effects of CBD oil and CBG oil to reduce pain and inflammation and improve locomotor activity and gait. Only CBG oil was chondroprotective, indicating that it could provide superior efficacy in patients with osteoarthritis.
CBD and CBG were shown to be effective at reducing inflammation in the phenylbenzoquinone (PBQ, an inflammatory agent)-induced writhing test in mice [24]. The two cannabinoids inhibited PBQ-induced writhing at oral doses of up to 10 mg/kg. CBD was the more potent producing maximal inhibition at a very low dose of 0.1 mg/kg. Other studies in mice have reported that CBG produces antinociceptive and anti-inflammatory effects in various pain models including those established by intraperitoneal administration of the inflammatory agents, formalin or carrageenan [25].
The efficacy of topical CBD for reducing inflammation and pain was assessed in a rat model of pain while simultaneously investigating the appearance of any adverse effects. CBD gels (0.6, 3.1, 6.2, or 62 mg/day) were applied once daily for 4 consecutive days after arthritis induction. On the fourth day, measured CBD concentrations showed that transdermal absorption was efficient and linear with the 0.6–6.2 mg/day doses. Topical CBD gel dose-dependently decreased joint swelling and pain, infiltration of immune cells, and synovial membrane thickening. Tissue analysis of the spinal cord and dorsal root ganglia showed that CBD dose-dependently reduced the pro-inflammatory biomarkers, calcitonin gene-related protein (CGRP), and OX42, in the spinal cord and TNF-α in dorsal root ganglion. The 6.2 and 62 mg/day CBD doses were effective in producing improvements, while not adversely affecting higher brain function as animal activity appeared normal [26].
The GPR55 receptor is activated by delta-9-THC and THCV, whereas CBD is a selective antagonist of GPR55 [27]. Activation of GPR55 with a selective agonist enhances pro-inflammatory responses in macrophage-derived foam cells and causes induction of pro-inflammatory, TNF-α levels and a reduction in anti-inflammatory, IL-10 levels [28]. The antagonism of GPR55 by CBD provides a mechanism to indicate its possible involvement in reducing inflammatory responses. Studies in GPR55 knockout mice have shown reduced inflammatory and neuropathic pain in models of inflammation induced by adjuvants and partial nerve ligation [29]. After adjuvant administration into the paws of the animals, inflammatory mechanical hyperalgesia was abolished in the GPR55 knockout mice for up to 14 days post-treatment. Increased levels of the anti-inflammatory cytokines, IL-4 and IL-10, were observed in the paws of the GPR55 knockout mice when compared against levels in wild-type mice (no knockout of the GPR55 receptor). These results suggest GPR55 signalling can affect the regulation of some cytokines, possibly contributing to the absence of inflammatory mechanical hyperalgesia in the knockout mice [29]. In the model of neuropathic hypersensitivity, GPR55 knockout mice also did not develop mechanical hyperalgesia for up to 28 days post-insult. These research findings indicate that CBD effects on GPR55 could offer the potential for the treatment of inflammatory and neuropathic pain [29]. An earlier study by Staton et al. [30] also used a GPR55 knockout mouse line in models of adjuvant-induced inflammation and partial nerve ligation. After intraplantar administration of adjuvant, inflammatory mechanical hyperalgesia was not present in GPR55 knockout mice for up to 14 days post-administration. Interestingly, there were increased levels of IL-4, IL-10, and IFN-γ in the paws of the knockout mice compared with GPR55 wild-type mice.
Yimam et al. [31] employed widely used inflammatory and non-inflammatory animal pain models to assess the antinociceptive and anti-inflammatory activity of CBD when used alone and also when combined with bioflavonoid compositions. These models were the hotplate tail-flick test, the visceral pain model (writhing test), and carrageenan-induced rat paw oedema. CBD was tested at 5% topically and 5, 10, 20, and 40 mg/kg orally. Administered alone topically or orally, CBD produced dose-related, amelioration of pain in all models. Reduction of pain and inflammation in these models was noted when CBD was administered orally in combination with the oral administration of bioflavonoid compositions compared with the administration of CBD alone.
CBC has also been shown to decrease carrageenan-induced and LPS-induced inflammation in rats and mice, respectively [32, 33]. The carrageenan-induced increase in the levels of the cytokine-inducible nitric oxide synthase, IL-1β, and IL-6 was abrogated following treatment with CBC. It has also been observed to modestly inhibit thermal nociception and potentiate delta-9-THC-induced antinociception in mice [32, 34].
Models of ALS
The anti-inflammatory and antioxidant properties of CBG and CBD were demonstrated in an in vitro model of ALS using a motor neuron-like cell line. Pre-treatment with CBG alone and in combination with CBD (each at 2.5 and 5 µM concentrations) reduced neuroinflammation induced by LPS-stimulated macrophages. Pre-treatment with 5 µM CBD/CBG reduced the expression of pro-inflammatory, TNF-α and increased that of anti-inflammatory, IL-10 and IL-37 [35]. LPS stimulation increases oxidative stress, as shown by the upregulation of inducible nitric oxide synthase expression. CBD/CBG at the 5 µM dose combination decreased inducible nitric oxide synthase expression in the cells [35].
Activation of PPARγ produces beneficial anti-inflammatory effects in CNS diseases including ALS. The anti-inflammatory effect of PPARγ may be due to reduced production of inflammatory markers including NF-κB. In this regard, using the motor neuron-like cell line model of ALS, CBG, alone, and in a CBD/CBG combination treatment reduced the activation of NF-κB nuclear factor and elevated expression of PPARγ [35].
The anti-apoptotic properties of CBG and CBD were also demonstrated in this model [35]. Neuroinflammation produced apoptosis resulting in a loss of motor neurons and upregulation of Bax protein (a pro-apoptotic member of the Bcl-2 protein family). The Bcl-2 family regulates mitochondrial integrity via the controlled release of factors that are involved in apoptosis. A Bcl-2/Bax imbalance results in altered mitochondrial membrane potential and structure leading to the induction of apoptosis. A CBD/CBG combination treatment downregulated Bax protein expression and upregulated Bcl-2 expression, demonstrating the anti-apoptotic effect of this phytocannabinoid treatment.
Models of Alzheimer’s disease
Neuroinflammation is involved in various neurodegenerative diseases, including Alzheimer’s disease. Delta-9-THC was effective at removing intraneuronal amyloid-beta (Aβ) in a model of Alzheimer’s disease using a neuroblastoma cell line, as were CBD, CBG, CBN, CBC, delta-8-THC, and CBDV. They blocked the accumulation of Aβ and stimulated the degradation and removal of preformed Aβ aggregates [36]. This result was confirmed for CBD in another in vitro model of Alzheimer’s disease, where CBD pretreatment protected against Aβ toxicity in the cells [37].
Oxidative stress is a primary component of aging and is increased in Alzheimer’s disease. Oxytosis is a novel, oxidative stress-induced programmed cell death pathway that is distinct from apoptosis. In oxytosis, glutamate inhibits cystine uptake into neurons and consequently reduces glutathione synthesis, stimulates the production of mitochondrial reactive oxygen species, oxidative stress, calcium influx, and finally cell death. In cultured hippocampal neurons, delta-9-THC, delta-8-THC, CBD, CBN, CBG, CBC, and CBDV prevented oxytosis, which for CBN was shown to result from the targeting of mitochondria and promotion of antioxidant defences independently of cannabinoid receptors [38].
In Alzheimer’s disease models in mice, delta-9-THC inhibits acetylcholine esterase-induced aggregation of Aβ and improves spatial memory performance in the radial arm water maze [37]. A separate study reported that CBD reduced cortical IL-6 levels, although not TNF-α levels after Aβ intraventricular administration to mice and prevented the cognitive impairment of the animals in the Morris water maze [39]. In another mouse model of Alzheimer’s disease, Aβ was administered intracerebroventricularly with CBD given orally. CBD reduced the cognitive deficits induced by Aβ via modulation of microglial activity, promotion of neurotrophic factor release, and regulation of inflammatory genes, such as TNF-α and monocyte chemoattractant protein-1(MCP-1) [30]. Complementary to these studies, Silvestro et al. [40] demonstrated that CBD treatment improved cognitive function and reduced neuroinflammation in a mouse Alzheimer’s disease model.
Models of Parkinson’s disease
In a rat model of Parkinson’s disease induced by injecting the neurotoxin, 6-hydroxydopamine into the medial forebrain bundle of the brain, intracerebral administration of CBD for 3 days into the globus pallidus improved the motor asymmetry and fine motor skills of the animals when compared with the control group of rats [41].
Also using this rat model, Giuliano et al. [42] administered CBD chronically for 28 days. Animals treated with CBD showed reduced nigrostriatal degeneration and neuroinflammatory response together with improved motor performance. CBD treatment increased expression of the TRPV1 receptor in astrocytes, but not in microglial cells in this brain region.
Using the 1-methyl-4-phenyl-1,2,3,6-tetrahydropyridine (MPTP) model of Parkinson’s disease in mice, CBD was administered for five weeks [43]. Although CBD did not produce a significant improvement in tests of motor function, it did induce morphological changes in brain microglia, which was considered to result from an anti-inflammatory response.
Models of Huntington’s disease
Reduced numbers of CB1 receptors in the striatum is an important pathogenic factor in Huntington’s disease [44]. Delta-9-THC was found to improve deficits in motor coordination in a transgenic mouse model (R6/2 mice) of Huntington’s disease and mitigate striatal atrophy and huntingtin protein aggregate accumulation [44, 45]. A later study [46] investigated the effects of CBG on Huntington’s disease pathology in a 3-nitropropionate-induced model in mice. Treatment with CBG inhibited neuronal death by approximately 50% and reduced the upregulated expression of COX-2, inducible nitric oxide, and pro-inflammatory cytokines including TNF-α and IL-6. Moreover, CBG treatment decreased the motor deficits in the animals. The potential neuroprotective properties of CBG were also evaluated in a transgenic mouse model of Huntington’s disease [46]. Treatment with CBG produced a smaller, but significant, recovery in motor deficits assessed by performance in the rotorod test. Genes linked to Huntington’s disease that are dysregulated in these mice were partially normalized by CBG treatment. There was also a moderate improvement in the altered gene expression of the anti-inflammatory peptide, PPARγ.
Models of autoimmune encephalomyelitis
A delta-9-THC/CBD combination treatment (10 mg/kg each), but not delta-9-THC or CBD used alone, attenuated experimental autoimmune EAE in mice through reduction of neuroinflammation and suppression of T cells. These effects were reported to be mediated via CB1 and CB2 receptors as the THC/CBD combination treatment did not reduce neuroinflammation in mice that were deficient in these receptor subtypes. The delta-9-THC/CBD combination treatment also decreased levels of the pro-inflammatory cytokines (IL-17, INF-γ, TNF-α, IL-1β, and IL-6), and increased anti-inflammatory types including IL-4, IL-10, and TGF-β [47].
Models of cerebral hypoxia, ischemia and brain injury
In hypoxic-ischemic, immature mouse brains, CBD treatment significantly reduced the expression of inflammatory markers including IL-6, TNF-α, COX-2, and inducible nitric oxide in ex vivo brain slices. By using selective antagonists, this effect was fund to be mediated by CB2 and adenosine A2A receptors, but not by CB1 receptors [48].
In a model of transient cerebral ischemia in rats, intracerebral CBD administration produced a decrease in brain lesion size compared with the control group of rats. Additionally, there were significant correlations between the reduction of infarct volume and TNF receptor 1/NF-κB expression, indicating that CBD reduced the size of the brain lesion possibly through the reduction of TNF receptor 1/NF-κB-induced neurotoxicity [49].
In a model of traumatic brain injury in rats, delta-9-THC when given post-injury improved anxiety-like behavior in an elevated plus maze, but not in an open field task [50]. Performance in the forced swim task (a model of depression) was improved in male rats, but a more severe depression-like behavior was reported in female rats. Increased microglial activation was observed, similar to findings in previous studies [51, 52]. However, there was no effect of delta-9-THC treatment on microglial activation in brain limbic system structures. In another rat model, delta-9-THC did not improve deficits in motor function caused by traumatic brain injury [53].
Traumatic brain injury in mice produced impaired sociability, increased aggression, and tactile allodynia 14 days post-insult. Daily treatment with 10% CBD oil orally for 14 days improved these behavioral parameters and also reduced the post-injury increases of the neurotransmitters, D-aspartate, glutamate, and GABA in the medial prefrontal cortex [54]. In a separate study in rats, CBD administered 30 min before traumatic brain injury and again 6 hours post-injury, produced improvements of blood-brain barrier integrity. CBD treatment also reduced concentrations of the proinflammatory mediators TNF-α and IL-1β when compared with the control group animals [55].
Summary of the non-clinical studies
In the numerous non-clinical studies described in the above sections, delta-9-THC, CBD, and CBG as well as other minor phytocannabinoids have been shown to have positive effects to reduce the activity of various mediators of inflammation (including cytokines, TRP channels, GPR55 and nuclear transcription factors) on oxidative activity and on programmed cell death. These reports show that phytocannabinoids can exert their effects through multiple physiological mechanisms. The molecular mechanisms of action underpinning the effects of phytocannabinoids on inflammation are, however, complex and are still to be clarified fully by further research.
Clinical evidence for the therapeutic role of phytocannabinoids in neuroinflammatory conditions
It is often incorrectly asserted that the therapeutic benefits of the phytocannabinoids have not been adequately demonstrated in clinical trials and claims for their medical use are based on anecdotal evidence and case reports. While this opinion is undoubtedly true in some cases, phytocannabinoids have well-established clinical efficacy with products approved for the treatment of a range of neurological and other disorders. Sativex® (nabiximols) is an approximately 1:1 mixture of delta-9-THC and CBD extracted from Cannabis sativa that has been approved in Europe to provide symptom improvement in adult patients with moderate to severe spasticity due to MS [56]. Although not yet approved in the USA, Sativex® is currently in Phase 3 clinical trials in MS as a prerequisite to evaluation by the FDA (Food and Drug Administration) for clinical use. Epidyolex® (Europe) or Epidiolex® (USA) is a highly purified (> 98% pure) CBD extract from Cannabis sativa that is approved in Europe, USA, and many other countries to reduce or prevent seizures in three rare childhood epilepsies, i.e., Lennox-Gastaut syndrome, Dravet syndrome, and tuberous sclerosis complex [57]. Although Marinol® and Syndros® (dronabinol) is comprised of chemically synthesised delta-9-THC, it is chemically and pharmacologically identical to the delta-9-THC extracted from Cannabis sativa leaves and flowers. Marinol® and Syndros™ are not approved for clinical use in Europe but are approved to treat anorexia associated with weight loss in patients with AIDS (acquired immune deficiency syndrome) and to prevent nausea and vomiting caused by cancer chemotherapy in the USA [58, 59]. Although it is not a phytocannabinoid, mention should also be given to nabilone (Cesamet®) which is a synthetic chemical analogue of delta-9-THC that is approved in Europe and the USA to control nausea and vomiting caused by chemotherapeutic agents used in the treatment of cancer [60]. Like delta-9-THC, nabilone is a potent CB1 and CB2 receptor agonist.
In May of 2024, the US Department of Justice announced that the Attorney General was initiating the process of requesting that the scheduling of marijuana under the Controlled Substance Act (CSA) to be changed from C-I to C-III [61]. The criterion for placing a drug in C-I is because it poses a significant risk of abuse and dependence and has no approved medical use. The rescheduling of marijuana to C-III marks a seismic shift in the attitude of USA legislators and lawmakers because first, it implicitly accepts that marijuana has medical benefits, and second, C-III scheduling is commensurate with the abuse/dependence risk of marijuana being classed as moderate. At the time of writing this review, the rescheduling process is in progress, but the recommendation has not been accepted or ratified by USA legislators. It is important to emphasize that rescheduling marijuana into C-III is not the equivalent of legalization for medical use, which at the Federal level, is still a long way off.
In addition to the clinically proven efficacy of phytocannabinoids, there is a wealth of trials that are seeking to investigate the therapeutic potential of these interesting molecules in a range of diseases and disorders. In the following section, we describe the clinical evidence relevant to the use of various phytocannabinoids in the management of neuroinflammatory disorders and their associated comorbidities.
Multiple sclerosis
MS is an auto-immune disease in which inflammation of the brain and spinal cord causes damage to the myelin sheaths surrounding nerves and the nerve axons that impede neurotransmission. The deficits can produce an enormous range of adverse physiological manifestations including impaired autonomic, motor, sensory, cognitive, and behavioral functioning, and also, psychiatric problems. The recurrent pattern of MS episodes occurs because the nerve fibres partially repair the damage. However, recovery is only temporary resulting in progressively shorter periods of remission and more severe and debilitating relapses [62–65].
According to its European Medicines Agency (EMA) Summary of Product Characteristics, the efficacy and safety of Sativex® oromucosal spray for alleviating spasticity in MS has been evaluated in pivotal trials in more than 1,500 patients [56]. Although the efficacy of Sativex® was not encouraging in initial clinical trials, subsequent trials that focused on the ability of Sativex® to maintain neurophysiological function demonstrated significant efficacy in reducing spasticity and slowing the progressive decline in neuromotor function.
There are three scientifically plausible and complementary mechanisms that would deliver therapeutic benefit in MS, i.e., the anti-inflammatory properties of phytocannabinoids, inhibition of excessive glutamatergic neurotransmission/excitotoxicity mediated via CB1 and CB2 receptor activation, and direct action to facilitate neuronal firing mediated by CB1 receptor activation [66–69]. As discussed in earlier sections of the review, numerous studies have shown that delta-9-THC has anti-neuroinflammatory properties. For instance, delta-9-THC increases the production of anti-inflammatory cytokines while simultaneously decreasing pro-inflammatory cytokine production in vitro and in vivo e.g., [21, 35, 70, 71]. All of these mechanisms, which are dependent on activation of CB1 and/or CB2 receptors, are independent of a pharmacological contribution from CBD because this molecule does not interact with either cannabinoid receptor subtype at clinically relevant doses. This conclusion does not imply that CBD makes no therapeutic contribution to the efficacy of Sativex® in the treatment of MS; rather it highlights that the mechanism or mechanisms responsible for its benefits have not yet been identified.
The potential medical benefits of the phytocannabinoids present in Cannabis sativa have been extensively studied in patients with MS. Most trials have employed fixed-dose delta-9-THC/CBD combinations, but some have investigated whole-plant extracts, and the effects of smoked Cannabis on spasticity in MS have been studied in 1 trial.
Vaney et al. [72] conducted a double-blind, placebo-controlled trial in 57 patients with MS using an active treatment consisting of a whole-plant Cannabis extract containing 2.5 mg delta-9-THC and 0.9 mg CBD. At the end of 2 weeks, there were trends for improvement, but no statistically significant effects of the active treatment on spasticity or mobility. This outcome was encouraging and consistent with the relatively modest beneficial effect of Sativex® in much larger clinical trials. Wade et al. [73] carried out a small placebo-controlled trial to evaluate the efficacy of whole-plant extracts of Cannabis sativa to compare delta-9-THC, CBD, and 1:1 delta-9-THC/CBD in 24 subjects consisting mainly of patients with MS (n = 18), but also including subjects spinal cord injury (n = 4), brachial plexus damage (n = 1), and limb amputation due to neurofibrobromatosis (n = 1). They reported that delta-9-THC and delta-9-THC/CBD significantly reduced spasticity and CBD decreased pain in this mixed group of patients. Pain relief with the active treatment was significantly superior to placebo, and in some patients, impaired bladder control, muscle spasms, and spasticity were also improved. Following on from this preliminary study, Wade et al. [74] conducted a much larger Phase 2 trial in MS using 1:1 delta-9-THC/CBD (Sativex®) to determine whether it would have a beneficial impact on the panoply of MS symptoms by reducing the most troubling symptom for each patient as the primary outcome. Although the study failed to meet its primary objective, it did observe that delta-9-THC/CBD significantly reduced spasticity in these patients. This was an important finding because it revealed that these cannabinoids were unlikely to be a panacea in treating MS when administered alone or in combination, and it pointed to key facets of MS that could benefit from this treatment.
Zajicek et al. [75–79] have conducted a sequence of large-scale randomized clinical trials to evaluate the possible therapeutic benefits of delta-9-THC/CBD combinations and delta-9-THC as monotherapy and in the management of MS. The initial Phase 3 CAMS (CAnnabinoids for Multiple Sclerosis) trial was a 15-week, randomized, double-blind, placebo-controlled trial conducted on more than 600 individuals with MS across 33 UK centres. Its aim was to investigate whether synthetic delta-9-THC (Marinol™) or a 2:1 fixed-dose delta-9-THC/CBD combination would reduce overall spasticity scores relative to placebo (primary outcome) and ameliorate some other debilitating effects of MS. The outcome of the CAMS trial was disappointing because both treatment interventions failed to achieve the primary outcome, although some statistically significant therapeutic effects, notably reductions in patient-reported spasticity and pain were observed [75]. The CAMS trial was extended using a sub-cohort of individuals from each treatment arm who agreed to continue on their allocated intervention on a double-blind basis out to 12-months (delta-9-THC: n = 138; delta-9-THC/CBD: n = 125; placebo: n = 120) [76]. The statistically significant, modest treatment effects of both cannabinoid treatments were confirmed together with potential improvements in some aspects of disability being observed in the delta-9-THC-treated subjects [75]. Complementing these preliminary positive signals was the observation that delta-9-THC and delta-9-THC/CBD treatments significantly reduced urinary urge incontinence episodes in MS patients [79]. Uncontrolled tremor is a common, disabling neurological consequence of MS. Fox et al. [78] conducted a small, placebo-controlled crossover trial to evaluate whether self-titrated delta-9-THC (≤ 25 mg/day) would reduce tremor in MS patients, but found no beneficial effect on this symptom. Clear evidence of the benefits of cannabinoids in treating MS emerged from a second Phase 3 trial which studied an ethanolic extract from the Cannabis sativa plant containing delta-9-THC/CBD (2.5 mg delta-9-THC/0.8–1.8 mg CBD) [77]. In this 12-week, randomised, double-blind, placebo-controlled, multicentre trial in 279 individuals with MS, the Cannabis extract (maximum daily delta-9-THC dose = 25 mg/day) produced highly significant reductions in muscle stiffness, muscle spasms, and pain relative to placebo together improved sleep quality that was evident at week-4 and maintained throughout the trial. The Cannabis extract intervention was safe and well tolerated in this group of MS subjects [77].
As described above, the ability of cannabinoids to reduce spasticity in MS is a robust and reproducible outcome that is predominantly based on pharmaceutical delta-9-THC/CBD combinations, e.g., Sativex®. However, Sativex® is not an approved drug in the USA and many other countries, and in addition, many MS sufferers seek to self-medicate their symptoms using Cannabis. In a placebo-controlled, crossover trial in a mixed population of Cannabis-experienced and Cannabis-naïve subjects with MS with substantial spasticity, smoked Cannabis (4% delta-9-THC) on 3 occasions reduced the level of spasticity by ~50% compared with smoking placebo cigarette. The side effects of smoked Cannabis were similar to oral delta-9-THC with the addition of unacceptable levels of feeling “high” in some subjects [80].
In addition to the other adverse manifestations of MS, pain is a common symptom that affects ≤ 70% of sufferers. Rog et al. [81] had previously reported the therapeutic benefits of delta-9-THC/CBD to reduce pain intensity and sleep disturbance caused by MS in a group of 66 patients with MS accompanied by central pain (n = 59 with dysesthetic pain, n = 7 with painful spasms). In these patients, treatment with 2.5 mg delta-9-THC/2.7 mg CBD (Sativex® oro-mucosal spray, maximum of 25 sprays/day) produced moderate but highly significant improvements on both outcome measures. Delta-9-THC/CBD was well tolerated, but the expected delta-9-THC side effects of dizziness, somnolence, and mild cognitive impairment were observed.
Russo et al. [82] conducted a small, open-label trial in 20 patients with MS divided equally between groups with and without neuropathic pain. Subjects were permitted to use Sativex® (average dose 8 sprays/day) for 4 weeks. Treatment with Sativex® improved a number of outcomes in both patient groups, but crucially, it markedly and significantly decreased the self-rated pain scores and improved the quality of life in MS patients with neuropathic pain.
Given that delta-9-THC and the other psychoactive peptides present in Cannabis sativa have abuse and dependence potential and can also induce troublesome psychiatric adverse events, the safety risk associated with initiating Cannabis-naïve patients to delta-9-THC-containing medicines is an important consideration. Aragona et al. [83] conducted an 8-week (3-weeks on Sativex® or placebo with a 2-week washout period between treatments), crossover trial in 17 Cannabis-naïve MS patients with and found that the intervention was well tolerated with no evidence of dependence or withdrawal. That said, there were significant correlations between the plasma concentration of delta-9-THC and scores for “anxiety”, “aggression”, and “paranoia”. Other side effects associated with Sativex® therapy were dry mouth, fatigue, nausea/vomiting, drowsiness, dizziness, and lower limb weakness.
Collectively, the clinical evidence provides sound support for the use of delta-9-THC/CBD to treat spasticity in MS with preliminary evidence indicating benefits in reducing pain.
The Sativex® (delta-9-THC/CBD)—EMA Summary of Product Characteristics [56] also reports data obtained in a 12-week randomized, double-blind, placebo-controlled trial to investigate whether it reduced spasticity in 72 children and adolescents suffering from cerebral palsy or traumatic brain injury. Unfortunately, the outcome of the trial was Sativex® did not produce a statistically significant reduction in the severity of spasticity in this patient population.
A literature search for reports from clinical trials that have investigated CBD or delta-9-THC as monotherapy treatments for MS unearthed only 2 small clinical trials. The preliminary investigation by monotherapy with delta-9-THC or CBD [74] has been discussed earlier in this section. Svendsen et al. [84] evaluated the effect of delta-9-THC (Marinol®) on central pain in a group of 24 subjects with MS and observed modest, but statistically significant, reductions in spontaneous pain and some related measures; however, treatment with delta-9-THC did not improve a raft of sensory testing outcomes in these patients.
Pain
Neuroinflammation can induce chronic neuropathic pain that is characterized by increased sensitivity to painful stimuli (hyperalgesia), the perception of innocuous stimuli as painful (allodynia), and spontaneous pain. Neuropathic pain may occur after a bout of shingles (post-herpetic neuralgia) or as a secondary consequence of nerve trauma or trapping. Neuropathic pain is typically unresponsive to treatment with conventional analgesics, e.g., paracetamol, salicylates, and opioids. Antidepressants, e.g., amitriptyline and duloxetine, and anticonvulsants, e.g., gabapentin and pregabalin, are effective in treating neuropathic pain in some patients, but this condition remains an enormous area of unmet clinical need.
Sativex® has undergone clinical testing as a potential treatment for neuropathic pain characterized by allodynia [85], diabetic neuropathy [86], and neuropathic pain associated with MS [77, 81, 82]. In addition, Sativex® has also been evaluated for the management of pain caused by rheumatoid arthritis [87]. The positive effects of Sativex® in treating neuropathic pain caused by MS [77, 81, 82] were discussed in the previous section. Nurmikko et al. [85] evaluated the efficacy and safety of Sativex® in a 1:1 randomized, double-blind, placebo-controlled 5-week clinical trial in 125 subjects with neuropathic pain of peripheral origin. Compared with the placebo, Sativex® significantly decreased scores on the Numerical Rating Scale of pain intensity, the Neuropathic Pain Scale composite score, measures of dynamic and punctate allodynia, and the Pain Disability Index. In the open-label extension trial, the attenuation of neuropathic pain by Sativex® was maintained out to 1 year. A less successful outcome was recorded when Sativex® was evaluated as a treatment for painful diabetic neuropathy [86]. This small, randomized, placebo-controlled trial was conducted in a group of 30 subjects with painful diabetic neuropathy who took Sativex® or a placebo for 12 weeks (2-weeks titration and 10-weeks maintenance). Sativex® produced no statistically significant improvement in either the mean daily pain scores (primary outcome measure) or quality-of-life assessments (secondary outcome measures). The authors suggest the failure was due to the influence of depression in some subjects, but given the small size of the trial, this proposal is no more than conjecture.
Several studies have also demonstrated the pain-relieving effects of inhaled or smoked Cannabis in post-traumatic, post-surgical, and diabetic neuropathy [88–90]. Although the studies report that efficacy was generally accompanied by the emergence of CNS side-effects typically associated with taking psychoactive cannabinoids, Wilsey et al. [89] reported that the pain-relieving effect of vaporized Cannabis was also evident at doses that did not impair daily functioning. Viewed overall, there is reasonable evidence to support the proposal that Cannabis is an effective treatment for neuropathic pain. In contrast, Cannabis offers no relief against nociceptive pain or hyperalgesia as demonstrated by its inability to reduce pain induced by electrical stimulation, heat, capsaicin, or secondary sunburn induced hyperalgesia in a well-designed placebo-controlled crossover study in 18 subjects [91].
Blake et al. [87] performed a randomized, double-blind, parallel-group, placebo-controlled trial to evaluate whether Sativex® could reduce the burden of pain in patients with rheumatoid arthritis. The trial enrolled 58 subjects who received daily treatment for 5 weeks with either Sativex® or placebo. Compared with placebo, Sativex® produced significant reductions in several pain endpoints (pain on movement, pain at rest and pain at present, and Short-Form McGill Pain Questionnaire) and improvements in quality of sleep and disease activity. Most adverse effects were mild or moderate, no serious adverse effects were reported in the Sativex®-treated subjects, and there were no withdrawals due to adverse events.
CBD has also been studied in clinical trials to determine whether it has analgesic properties.
Xu et al. [92] determined the efficacy and safety of topically applied CBD oil in a 4-week, double-blind, randomized controlled trial in patients with neuropathic pain. Twenty-nine subjects were treated up to four times daily with CBD (250 mg, n = 15) or placebo oil (n = 14). The Neuropathic Pain Scale rating was completed twice-weekly to assess the change from baseline to the end of the treatment period. The study population included 62% males and 38% females with a mean age of 68 years. Compared with placebo, topically applied CBD significantly reduced intense pain, sharp pain, cold, and itchy sensations caused by peripheral neuropathy and showed a strong trend for reducing deep pain. There were no adverse events associated with topically applied CBD.
Low doses of orally administered CBD (20 or 30 mg/day) had no beneficial analgesic effect on pain associated with osteoarthritis or psoriatic arthritis in a double-blind placebo-controlled trial in 136 subjects [93]. CBD also produced no positive effects on sleep quality, depression, anxiety, or pain catastrophizing scores [93]. Very recently, Bawa et al. [94] conducted an open-label, feasibility trial in 15 subjects to evaluate a topically applied CBD gel as a potential treatment for osteoarthritis-induced pain and impaired manual function. With the caveat there was no placebo control, the results were promising with the application of CBD (4%) gel applied thrice daily for 4 weeks producing significant reductions from baseline in all pain measures, increased grip strength, and improvement in the quality-of-life scores for sleep, anxiety, stiffness, and fatigue. The penetration of CBD into the body was confirmed by urinary measurement of CBD and its major metabolite [94].
Although some areas of disagreement exist between studies, the picture emerging from clinical trials is one of accumulating evidence to show that neuropathic pain and its complications are responsive to treatment with delta-9-THC/CBD combinations, and also to delta-9-THC or CBD as monotherapy. More research is required to support these hypotheses, but areas of interest would be to enlarge the therapeutic window between efficacy and CNS side-effects for delta-9-THC-containing products, and the use of topical formulations of CBD that deliver high levels of drug to its site of action.
Alzheimer’s disease
Accumulation of Aβ and neuroinflammation play a critical role in the pathogenesis and development of Alzheimer’s disease. Activated microglia in the CNS and increased levels of inflammatory mediators around Aβ plaques in the brains of patients are linked to the pathogenesis of the disease. Aβ and neurofibrillary tangles can indirectly activate the immune response and/or microglia, leading to the release of various pro-inflammatory cytokines and neurotoxic mediators, including pro-inflammatory cytokines, such as IL-1β, IL-6, and TNF-α, plus superoxide, nitric oxide, reactive oxygen species and the expression of inducible nitric oxide synthase e.g., [37, 39, 40]. Alterations to the endocannabinoid system in Alzheimer’s disease have also been observed [95]. CB2 receptor upregulation has been consistently observed in ex vivo brain tissue taken from Alzheimer’s patients and in rodent models of the disease [96]. In contrast, reductions in CB1 receptor density have been reported to occur in areas of microglial activation in the brains of Alzheimer’s sufferers and also in animal models of the disease [96]. CB2 receptor expression can be initiated by immunomodulation induced by pathogenic events occurring in the disease. In clinical studies, increased levels of fatty acid amide hydrolase and CB2 receptor density have been found in microglia adjacent to plaque-associated hippocampal tissue [97]. Moreover, these changes were positively correlated with Aβ levels and plaque deposition [98]. Conversely, stimulation of microglial CB2 receptors initiated the removal of native Aβ from human brain tissue sections [99].
In patients with Alzheimer’s disease or dementia, some studies have reported that delta-9-THC improved non-cognitive symptoms (behavioral and psychological symptoms of dementia) such as agitation [100, 101], whereas other studies have observed no beneficial effect of delta-9-THC on dementia symptoms [102, 103]. For example, 21 days of treatment with a low-dose, oral delta-9-THC (1.5 mg, 3-times daily) did not affect dementia-related neuropsychiatric symptoms, although it was well tolerated with no clinically relevant side effects [103].
In a placebo-controlled, crossover trial, 12 patients with Alzheimer’s disease who were treated daily for 6 weeks with dronabinol (synthetic delta-9-THC) displayed improvements in disturbed behavior [104]. Adverse reactions that were more frequently reported by subjects taking dronabinol than placebo included euphoria, somnolence, and tiredness. However, it should be noted that these adverse events did not require discontinuation of treatment. In an open-label pilot study in 5 Alzheimer’s patients, 2 weeks treatment with dronabinol (2.5 mg daily) reduced unwanted night-time activity and resulted in improvements in the Neuropsychiatric Inventory total score, aberrant motor behavior, and agitation in patients with advanced stages of the disease [105].
The synthetic, delta-9-THC analog, nabilone (1–2 mg/day administered orally) significantly reduced agitation and improved overall neuropsychiatric symptoms in a group of 39 patients with Alzheimer’s disease in a randomized, double-blind, crossover trial (6-weeks active treatment, 6-weeks placebo separated by a 1-week washout period). Mild sedation was the only adverse event of note [100].
There is the possibility that CBD’s anti-inflammatory and antioxidant properties have neuroprotective actions that could slow the progression of Alzheimer’s disease. Enhancing the brain concentration of the endogenous cannabinoids, anandamide, and 2-AG, by preventing their enzymatic catabolism is a therapeutic approach worthy of consideration. In support of this approach, frontal and temporal cortical tissues taken from 38 post-mortem patients who had been suffering from Alzheimer’s disease had significantly reduced levels of anandamide and its precursor compared with samples taken from 17 age-matched control subjects [106]. Clinical studies have shown that treatment with CBD increases the plasma concentration of anandamide [107]. CBD may elevate levels of the endocannabinoid, anandamide, within body tissues via two potential mechanisms: First, by restricting the transport process facilitated by fatty acid binding proteins, and second, by inhibiting fatty acid amide hydrolase, an enzyme responsible for the catabolism of anandamide.
Huntington’s disease and Parkinson’s disease
Promising benefits of Cannabis and medical marijuana have been reported for the alleviation of neurological symptoms in patients with movement disorders including Huntington’s and Parkinson’s disease.
Akinyemi et al. [108] conducted a meta-analysis on the results from 22 trials conducted after 2002 that have investigated the therapeutic effects of medical marijuana or Cannabis in Huntington’s disease. The outcome measures were changes in psychomotor- and sleep-related symptoms. The meta-analysis provided strong evidence for significant improvements in the neurological symptoms of spasms, tremors, spasticity, chorea, and quality of sleep following treatment with medical marijuana. Analysis of specific motor symptoms using Huntington’s disease-specific rating scales also found significant treatment-related improvements in the severity of tremors and rigidity. In contrast to the positive findings with marijuana or Cannabis, a double-blind, randomized, placebo-controlled trial to evaluate CBD in patients with Huntington’s disease observed no beneficial effect of 10 mg/kg/day oral CBD (i.e., roughly 700 mg/day) on Huntington’s disease-related symptoms (e.g., chorea sensitivity, sustained tongue protrusion, functional ability, or information recall and storage) [109]. One explanation for the lack of efficacy of CBD in this trial could have been due to the low dose that was tested.
In an exploratory, double-blind trial in 7 subjects with Parkinson’s disease who did not have dementia or other comorbid psychiatric disorder, a 6-week regimen of oral CBD therapy (300 mg/day) did not improve motor function (assessed via the Unified Parkinson’s Disease Rating Scale), but it did improve the patient’s quality of life [110]. CBD also improved REM (rapid eye movement) sleep behavior disorder in 4 patients without producing side effects [110]. In an open-label pilot study in 6 Parkinson’s disease patients, CBD starting at 150 mg/day for 4 weeks, improved psychotic symptoms without adverse side-effects or negative effects on motor neurons [111]. In a later open-label trial, 10 individuals with Parkinson’s disease were given CBD treatment (20–25 mg/kg/day) for 10–15 days. The results showed mean decreases in total and motor Movement Disorder Society Unified Parkinson Disease Rating Scale scores at the maximal CBD dose compared with a baseline of 17.8% and 24.7%, respectively. Notably, participants also reported enhancements in non-motor functions like improved night-time sleep and a decline in emotional or behavioral dysregulation (e.g., irritability and restlessness) [112].
Traumatic brain injury
Traumatic brain injuries destroy neural tissue resulting in impaired blood perfusion, excessive release of the neurotransmitter, glutamate (excitotoxicity), inflammation, and progressive secondary neuronal cell death. Very few studies have been conducted to explore the effects of phytocannabinoids in patients with traumatic brain injury, and no findings from randomized, controlled trials have been published.
Dexanabinol is a non-psychoactive, synthetic cannabinoid with anti-oxidant and anti-inflammatory properties. In a randomized, double-blind, placebo-controlled clinical study in 67 patients with severe traumatic brain injury, intravenous administration of dexanabinol (48 mg or 150 mg) within 6 hours of injury resulted in lower intracranial pressure and a reduced number of hypotensive episodes, indicating improvements in cerebral edema. Dexanabinol also improved recovery 1 month post-injury, and the intervention was safe and well tolerated in these patients [113]. Disappointingly, in the subsequent Phase 3 trial in 861 patients with severe traumatic brain injury, a single intravenous injection of 150 mg of dexanabinol administered within 6 hours of the injury failed to produce significant improvement versus placebo on the Glasgow Coma Scale at 6 months post-injury [114].
Summary of clinical trial results with phytocannabinoids
The therapeutic benefits of the phytocannabinoids are clearly demonstrated by the medical use of CBD (Epidiolex®) to prevent certain rare forms of childhood epilepsy, delta-9-THC (Marinol® and Syndros®) to manage nausea/vomiting associated with cancer chemotherapy and counteract anorexia associated with weight loss in AIDS patients, and CBD + delta-9-THC (Sativex®) to relieve spasticity in MS. In addition, nabilone (Cesamet®), which is a synthetic delta-9-THC analogue, is approved to treat chemotherapy-induced nausea/vomiting and AIDs-related weight loss. CBD and delta-9-THC exemplify the medical benefits of non-psychoactive and psychoactive phytocannabinoids, and also highlight diverse pharmacological mechanisms involved with delta-9-THC serving as a CB1/CB2 receptor agonist and CBD having no pharmacologically relevant affinity for these cannabinoid receptors. Although the findings are preliminary there is evidence emerging from clinical trials to indicate that delta-9-THC and CBD (alone or in combination) can help alleviate various forms of pain, e.g., neuropathic pain, inflammatory pain, and pain associated with MS. There is also evidence to suggest these phytocannabinoids may have beneficial effects in reducing agitation, improving sleep patterns and the quality of life in subjects suffering from a range of neurological diseases. Like all clinical investigations, there will be successes and failures, and as exemplified by dexanabinol, a positive result in a Phase 2 trial may not be replicated in larger, multi-site, Phase 3, clinical trials.
Viewed collectively, various outcomes observed in these clinical trials are consistent with CBD, delta-9-THC and potentially some other phytocannabinoids present in the Cannabis sativa plant having anti-neuroinflammatory properties, but more clinical research is required to answer the question definitively. The clinical trials definitively proving the therapeutic benefits of delta-9-THC/CBD and delta-9-THC monotherapy in MS were conducted well over 10 years ago, and further development work on these compounds is unlikely. Although legislators in many countries are relaxing their laws to allow self-medication with Cannabis, there is a dearth of new trials looking at the potential use of whole-plant Cannabis sativa extracts in new clinical indications. The development and approval of CBD to treat 2 rare forms of childhood epilepsy have produced an upsurge of interest in evaluating CBD in a range of other indications including anxiety, psychosis, autism, pain, and HIV. Since CBD is devoid of clinically relevant affinity for CB1 and CB2 receptors, the hypothesized pharmacological mechanisms predicting efficacy in these indications must, therefore, be independent of cannabinoid receptor activation and based on the interaction of drug novel targets. An area where we foresee new research is on the raft of lesser-known phytochemical and novel synthetic psychoactive cannabinoids that are now emerging [115]. It is highly probable that the pharmacological properties of these newer cannabinoids will be exhaustively investigated to determine whether they have potential as drug candidates for clinical development.
Conclusions
Research with phytocannabinoids and their synthetic analogues is long past the point at which their value as medical treatments was unproven. CBD, delta-9-THC, and synthetic analogues of these compounds have been evaluated in rigorous, placebo-controlled trials where their therapeutic benefit has been unequivocally demonstrated. The approval of CBD to prevent specific types of seizures in children opened the doors to investigating the therapeutic potential of phytocannabinoids through mechanisms that do not necessarily involve the activation of CB1 or CB2 receptors.
Preclinical research has demonstrated the anti-inflammatory and anti-neuroinflammatory properties of various phytocannabinoids, and in addition, has identified a plethora of mechanisms that could mediate the actions of these compounds. Early clinical trials have revealed outcomes that are consistent with delta-9-THC, CBD, and other phytocannabinoids delivering efficacy by relieving inflammation and preventing the production of inflammatory mediators. What is now required are more clinical trials to confirm or disprove these preliminary findings together with closer ties between non-clinical and clinical research to forge a link between molecular mechanisms and therapeutic outcomes.
It should also be appreciated that almost all clinical research on phytocannabinoids has concentrated on the most abundant compounds, i.e., delta-9-THC and CBD, there are more than one hundred other psychoactive and non-psychoactive cannabinoids present in the Cannabis sativa plant, and many of them have not even been pharmacologically characterized at the non-clinical level. They comprise a valuable resource for future research.
Attitudes to the legalization of Cannabis are changing and part of the driver for change is a recognition that the Cannabis sativa plant produces phytocannabinoids that have been claimed to have medical benefits. Although most of these claims have never been proven to the standard required for regulatory approval, the anecdotal evidence is sufficiently compelling for the medical use of Cannabis to have been legalized in 38 USA states and in the federal District of Columbia.
To conclude, the endocannabinoid system in the human body plays a key role in a multitude of physiological functions including inflammatory and immunological responses, pain, temperature control, endocrine regulation, feeding behaviour, learning and memory, emotional processing, and sleep. Many of these actions can be mimicked by the phytocannabinoid, delta-9-THC, which is an agonist at the human CB1 and CB2 receptor subtypes. However, the story is much more complex and interesting and the last decade has witnessed a seismic shift in our level of knowledge about the pharmacological mechanisms and clinical benefits that are offered by the phytocannabinoids present in Cannabis sativa. There is now tantalizing evidence to indicate that various phytocannabinoids have anti-inflammatory properties that could open the doors to the discovery and development of new treatments for a range of neuroinflammatory conditions.
Abbreviations
AIDS: | acquired immune deficiency syndrome |
ALS: | amyotrophic lateral sclerosis |
Aβ: | amyloid-beta |
CAMS: | CAnnabinoids for Multiple Sclerosis |
CB1: | cannabinoid receptor types 1 |
CBC: | cannabichromene |
CBD: | cannabidiol |
CBDV: | cannabidivarin |
CBG: | cannabigerol |
CBN: | cannabinol |
delta-9-THC: | delta-9-tetrahydrocannabinol |
EAE: | encephalomyelitis |
GPR55: | G55 protein-coupled receptor |
IL-1β: | interleukin-1β |
MS: | multiple sclerosis |
NF-κB: | nuclear factor-κB |
PPARγ: | peroxisome proliferator-activated receptor gamma |
THCV: | tetrahydrocannabivarin |
TNF-α: | tumor necrosis factor alpha |
TRP: | transient receptor potential |
TRPA: | transient receptor potential ankyrin |
TRPM: | transient receptor potential melastatin |
TRPV: | transient receptor potential vanilloid |
Declarations
Author contributions
SS: Conceptualization, Investigation, Writing—original draft, Writing—review & editing. TS and RS: Investigation, Writing—review & editing. DH: Investigation, Writing—review & editing. All authors read and approved the submitted version.
Conflicts of interest
David Heal and Sharon Smith are shareholders and employees of DevelRx Ltd. Ruarri Spurgeon is a Director of Medicanna Ltd and EthicaCBD. Taurri Spurgeon is Head of Marketing at Medicanna Ltd and EthicaCBD. The authors confirm they did not receive any external financial support for writing this article.
Ethical approval
Not applicable.
Consent to participate
Not applicable.
Consent to publication
Not applicable.
Availability of data and materials
Not applicable.
Funding
Not applicable.
Copyright
© The Author(s) 2024.