Abstract
Indoor air pollution (IAP) is an important cause of concern for human health, leading to millions of deaths worldwide each year. Since people spend most of their time indoor the quality of the air inhaled during routine activities is of primary importance. IAP include particulate matter (PM), volatile organic compounds (VOCs), chemical gases, heavy metals, and biological contaminants. Unfortunately, their sources are various and widespread all over the household and other indoor environments, causing relevant health consequences. This narrative review aims to provide a comprehensive framework of the indoor pollutants effects on subjects affected by asthma, allergic rhinitis, and atopic dermatitis. As pivotal barriers against pollutants, in fact, respiratory and cutaneous districts can be particularly affected by IAP, especially in case of atopic diseases. On the other hand, the application of targeted adjustments, such as the avoidance of cigarette smoking, the use of hoods while cooking, the choice of adequate ventilation systems, and the use of low-emitting building materials and furniture may result in the improvement of indoor quality.
Keywords
Allergic rhinitis, asthma, atopic dermatitis, atopic diseases, heavy metals, indoor pollution, particulate matter, volatile organic compoundsIntroduction
Indoor air pollution (IAP) represents an important but usually overlooked public health problem even though people tend to spend 80–90% of their time indoor [1]. According to the latest World Health Organization (WHO) data, 3.2 million people prematurely die each year from illnesses attributable to the IAP: 32% of these deaths are due to ischemic heart disease, 23% to stroke, and 46% to lung diseases comprising lower respiratory infections, chronic obstructive pulmonary disease (COPD) and cancer [2].
IAP comprises a heterogeneous mixture of solid (particulate) matter, liquid, and various gaseous components, differently composed depending on: (I) human activities in buildings, (II) household characteristics (i.e., construction materials, equipment, and furniture sources), (III) ventilation conditions, and (IV) outdoor pollution, especially in case of proximity to heavy emissions [2, 3]. Predictably, these factors may play different roles worldwide according to the different socioeconomic and environmental conditions. In low-middle income countries, about 3 billion people rely on the combustion of biomass fuels for cooking and heating. Such solid fuels (i.e., wood, manure, agricultural crop waste, and coal) are usually burned in inefficient and poorly vented combustion devices such as open fires or traditional stoves [4, 5]. Despite a lower use of biomass fuels, IAP in high-income countries originates also from cooking and heating but involves further indoor pollutants more typical of urbanized regions, including indoor particulate matter (PM), environmental tobacco smoke (ETS), allergens from indoor pests, toxic cleaning chemicals, and molds [4].
Atopic diseases, particularly asthma, allergic rhinitis (AR), and atopic dermatitis (AD), are associated with both IgE-mediated and non-allergic mechanisms that may coexist and that often result in interrelated clinical phenotypes expressing a multi-morbidity clinical picture [6]. Asthma is a chronic inflammatory disease of the airways characterized by cough, wheezing, shortness of breath, and chest tightness. It is very frequently sustained by a type 2 inflammation, involving T helper 2 (Th2) cells and type 2 innate lymphoid cells (ILC2) releasing key interleukins, such as IL-4, IL-5 and IL-13 [7]. AR is characterized by sneezing, nasal congestion, nasal itching, and nasal discharge [8], whereas AD is a common chronic inflammatory skin disease manifesting itself with pruritus and eczematous lesions in specific patterns across the body [9]. They both depend on a type 2-mediated reaction to environmental exposure acting on a predisposed genetic background [8, 9].
Indoor pollutants, especially PM and volatile organic compounds (VOCs), are known to influence the manifestation of atopic diseases but, despite an increasing awareness about this argument, the research performed on this topic is still relatively scarce. Literature is poor in a comprehensive framework investigating indoor pollutants effects on both children and adults affected by asthma, AR, and AD. The aim of this review, hence, is to provide a better insight into the current state of the art regarding the role of IAP in the exacerbation, development, and severity of atopic diseases, with particular regard for those involving respiratory and cutaneous districts, which represent pivotal barriers against pollutants.
Indoor pollutants
Indoor pollutants may generally be inorganic, organic, biological, or a blend of the previous categories [3]. Hereinafter, an in-depth overview of the major indoor pollutants.
PM
PM is a mixture of small particles and liquid droplets consisting of organic chemicals, metals, and dust particles [10]. PM is generally categorized by its size: particles are defined as “coarse” when their diameter is between 2.5 μm and 10 μm, as “fine” when it is between 2.5 μm and 0.1 μm, and as “ultrafine” when lesser than 0.1 μm [11]. Nose and throat deposition relates to PM ranging in size from 2.5 mm to 10 mm, whereas ultrafine PM tends to deposit in the small airways and alveoli, eventually acting as carriers for metals and other organic compounds translocating from the alveoli to the pulmonary circulation [12].
Indoor sources of PM include above all tobacco smoke, which is the major source of PM2.5, and cooking (through the burning of oil, wood, and food), but also heating, machine operation, and cleaning (through the re-suspending of dust particles and the facilitation of outdoor particles penetration inside) [3, 13].
Studies conducted on human and animal models found that diesel exhaust particles (DEPs), some of the major contributors to ambient PM, are implicated in pulmonary inflammation by modulating cell signaling pathways that cause the release of inflammatory mediators [14, 15]. The possible involvement of DEPs in the pathogenesis of asthma may be represented by the induction of an overexpression of IL-1b and granulocyte-macrophage colony-stimulating factor (GM-CSF), as well as chemokines, like C-C motif ligand 2 (CCL2)/monocyte chemotactic protein-1 (MCP-1), CCL20/macrophage inflammatory protein-3 alpha (MIP-3a), and chemerin. These molecules play a role in the recruitment of dendritic cells that internalize DEPs towards the pulmonary tissue, inducing a type 2 response, with consequent reduction of airway remodeling and increase of hyper-responsiveness [16, 17].
Relatively few studies have been conducted on the association of PM and asthma morbidity. The Baltimore Indoor Environment Study of Asthma in Kids (BIESAK) study was a longitudinal trial conducted on 150 inner-city preschool children with asthma that found indoor coarse PM concentrations to be associated with the increase in asthma symptoms [18]. For every 10 μg/m3 increase in indoor coarse PM concentration (due mainly to smoking, sweeping, and stove use), a 6% increase in the number of days of cough, wheezing, or chest tightness was reported. Moreover, for every 10 mg/m3 indoor increase in PM2.5, there was a 7% increase in days of severe wheezing and a 4% increase in days on which rescue medication was needed [18]. Another study conducted on more than 3,000 children in India found high levels of indoor PM, typical of industrial areas, to be associated with increased occurrence of asthma [17]. Similar findings were found in a recent cross-sectional study reporting a higher prevalence of asthma in the children of an Indian village, where both biomass and liquefied petroleum gas fuels were used for cooking [19]. Furthermore, a relationship between PM levels and asthma cases, especially in the dry season [20], as well as with acute asthma exacerbations [21] was also highlighted.
Fewer studies have been conducted to assess the relationship between exposure to PM and lung function in asthmatic patients. White et al. [22] observed decreased lung function in individuals with asthma frequently exposed to residential wood burning, with a 164 mL decrease in forced expiratory volume in the first second (FEV1) and a 2% decrease in FEV1/forced vital capacity (FVC) ratio compared to unexposed. In addition, frequent wood burning was not found associated with any significant difference in pulmonary function in healthy controls, contrary to airway inflammation. FeNO (fraction of exhaled nitric oxide), in fact, was found to increase in all highly exposed individuals, regardless of their asthma status [22]. A study conducted on 44 asthmatic children found that for every one-unit increase of indoor PM, mostly composed of PM2.5 and ultraviolet light absorbing PM released by smoldering organic, a 4.1% reduction in FEV1/FVC ratio was performed. Interestingly, outdoor PM was not found associated with a reduction in lung function [23]. A consistent reduction of FEV1 and maximal mid-expiratory flow (MMEF)—an indicator of small airway function was previously reported in children exposed to indoor PM2.5 levels, with significant mitigation mediated by the use of anti-inflammatory drugs [24].
As regards AR, an in vitro study showed how nasal epithelial cells exposed to PM2.5 may lose their barrier effect because of a decreased expression of junction proteins and an increased release of pro-inflammatory cytokines [i.e., thymic stromal lymphopoietin (TSLP)], and this could increase susceptibility to rhinitis and rhinosinusitis [25]. A confirmation of this hypothesis might come from the results of European multi-center studies reporting an association between long-term exposure to outdoor PM2.5 pollution and the severity score based on the intensity of disturbance reported by questionnaires [26]. On the other hand, such an association was not reported considering exposure to PM10 at school [27].
Conversely, PM2.5 and PM10 were found both to affect AD manifestations, probably due to an increased reactive oxygen species (ROS)-mediated damage in the stratum corneum [28]. Retrospective Asian studies performed on large cohorts of patients, in fact, reported a significant positive association between the levels of these pollutants and the number of visits for AD [29, 30], as well as for other skin diseases such as psoriasis, seborrheic dermatitis and rosacea [30].
VOCs
VOCs are organic chemicals that easily vaporize and spread in the environment under normal conditions. Being highly volatile, mobile, and resistant to degradation, they are able to be transported long distances in the environment [31]. The definition of VOCs varies slightly among different regulating bodies, but generally, this term refers to chemicals with a high vapor pressure at room temperature and at standard atmospheric pressure; they may also be defined as a compound characterized by an initial boiling point lower than 250°C [32]. The origin of VOCs may be both natural (volcanic eruptions, forest fires, etc.) and anthropogenic (exploitation, storage, refining, transport, usage of fossil fuels, and cooking). Isoprene, terpenes, and alkanes, as well as formaldehyde, benzenes, toluene, ethylbenzene, and xylenes, are all examples of biogenic VOCs, the latter being the most abundant compounds in the air [11, 33, 34].
Exposure to VOCs such as propylene glycol emitted by acrylic paints is able to cause dehydration of the respiratory mucus gel layer, leading to an overproduction of mucus due to goblet cell metaplasia. This also results in the thickening of the basement membrane and of the mucosa, which in turn causes decreased mucociliary clearance. This may lead to exacerbations of asthma, with coughing and wheezing [35].
The most widely studied VOC is formaldehyde, which is predominantly emitted by construction materials such as particleboard, fiberboard, and plywood. A case-control study conducted in Australia on 88 asthmatic patients found that subjects highly exposed to formaldehyde showed a 39% increase in the risk of developing asthma compared to those less exposed, especially in patients presenting symptoms such as runny nose and hay fever [36]. Another research defined ethylbenzene, among VOCs, as the most important cause of emergency room access for asthma [37]. A broader view of the impact of VOCs and PM on the upper airways comes from an Argentinian trial performed on children living near a petrochemical plant. Higher rates of asthma (24.8% vs. 10.1–11.5%), asthma exacerbations (6.7% per year vs. 2.9–3.6% per year), respiratory symptoms (current wheeze, dyspnea, nocturnal cough, and rhinitis), and lower lung function (> 13% decrease in FEV1 percent predicted) were reported compared to children living in other regions [38].
Cleaning products are a further source of VOCs. A certain exposure during infancy is related to a higher risk of recurrent wheezing, recurrent wheezing with atopy, and asthma diagnosis [39].
VOCs were described also as correlated to AD [40]. Measurements of urinary metabolites of toluene and mandelic acid were reported as associated with the presence of AD assessed through scoring AD (SCORAD) positive results [41]. Toluene exposure, in fact, has been described as an upregulation of mRNA expression of TSLP, which influences keratinocytes in the pathogenesis of AD [42].
Heavy metals
Heavy metals can be found in indoor dust because of the infiltration of outdoor pollutants (dust and soil), but also in smoke, fuel consumption products, and building materials. They can enter the human body through inhalation, ingestion, or dermal contact, performing several adverse effects [3]. For instance, the investigation of the effects of short-term indoor exposure to vanadium, an outdoor pollutant emitted also from the burning of residual oil fuel for residential heating, highlighted the alteration in DNA methylation of allergic and pro-inflammatory asthma genes (such as IL-4) in children, but not decrements in lung function [43].
Heavy metals can also be irritant, causing the worsening of AD symptoms. Patch tests using nickel, cobalt, and chromium may be performed to evaluate sensitivity to heavy metals, and such positivity is related to increased odds of a history of atopic eczema [44]. Furthermore, even indoor lead levels below the acceptable concentration (0.5 µg/m3) may affect AD [45].
Chemical gases
Sulfur dioxide (SO2), nitrogen dioxide (NO2), and carbon monoxide (CO) are products of the combustion of fossil fuels [34]. The most important sources of NO2 include tobacco smoke and gas-, wood-, oil-, kerosene-, and coal-burning appliances, such as stoves and fireplaces [46]. Few studies have investigated the association between indoor gas exposure and asthma morbidity. For instance, Hansel et al. [47] found higher indoor NO2 concentrations to be related to increased asthma symptoms in preschool inner-city children. Similarly, a study conducted on adult asthmatic patients showed worse disease control, but not a major risk of developing asthma, in those exposed to higher concentrations of NO2 in a home environment [21]. A study conducted among Nigerian children found no significant association between indoor exposure to NO2 and SO2 and increased prevalence of asthma, in contrast to what was reported for higher concentrations of household CO [20]. On the contrary, Taiwanese children living next to a petrochemical complex and exposed to high levels of SO2 had significantly higher incidences of asthma, bronchitis, and AR [48].
Ozone (O3) is produced in the atmosphere by oxidation of hydrocarbons and CO catalyzed by hydrogen oxide radicals and nitrogen oxide radicals. Indoor sources of O3 include O3 generators, commonly sold as air-freshening or air-cleaning devices, but also copy machines [49]. High exposure to O3 in bleachery workers from three Swedish pulp mills was related to an increased prevalence of adult-onset asthma, wheezing, and current asthma symptoms compared to unexposed individuals. They also had a higher median concentration of FeNO compared with the ones less exposed [19.2 part per billion (ppb) vs. 15.7 ppb] [50].
Regarding the role played by chemical gases on AD, a significant correlation between increases in SO2 and CO, but not in O3, concentrations and the number of visits was observed, whereas conflicting evidences were reported for NO2 [30]. According to a recent meta-analysis, even a short time exposure (a few days) to SO2 could be related to the exacerbation of AD, with a risk increase of 2.9% per 10 μg/m³ increment in SO2 exposure [51].
Biological contaminants
Biological contaminants are pollutants of biological origins. Major components in the air include bacteria, viruses, unicellular organisms, fungi, algae, mites, pests, and animal epithelia. Temperature and relative humidity are important factors that favor the growth and survival of biological contaminants, in particular molds and dust mites. The main source of survival and transport of microorganisms in the indoor air is represented by humans, carpets, stuffed toys, cushions, and pets [52].
Leaving aside the well-known effects of house dust mites (HDMs), cockroaches, and pet allergens on atopic diseases, also microbiome contaminants are worthy to be considered. Molds, in fact, are among the most widespread sources of biological IAP [53]. Many studies investigated the association between mold exposure and the risk of developing asthma. Asthmatic patients sensitized to molds may be associated with a higher degree of broncho-obstruction compared to those non-sensitized (75% vs. 79% FEV1/FVC ratio). Considering sensitization to specific fungal species, patients mainly sensitized to Aspergillus fumigatus have decreased pulmonary function compared to controls (71% vs. 79% FEV1/FVC ratio), whereas those mostly sensitized to Alternaria alternata, Penicillium spp., and Cladosporium spp. have comparable lung function to controls [54]. Hayes et al. [55] found that allergic asthmatic patients sensitized to Cladosporium were more likely to have a more severe form of asthma, whereas sensitization to Alternaria, Cladosporium, and Aspergillus was associated with a higher likelihood of previous hospitalization. Several mechanisms have been proposed to explain how fungal exposure can be involved in the pathogenesis of allergic asthma. For instance, fungi are recognized through their pathogen-associated molecular patterns by fungal receptors and therefore endocytosed by dendritic cells, which in turn activate Th2. The fungal proteases can directly damage the lung epithelium resulting in the release of alarmins, such as IL-33 and IL-25, which in turn stimulate Th2 and ILC2. Dendritic cells may also promote the Th17 pathway, resulting in the production of IL-17 [56]. As a confirmation of a type 2 activation, also a significant association between exposure to indoor mold or water damage was associated with increased prevalence of AD [57, 58]. Moreover, among VOCs, microbial VOCs (MVOCs) are VOCs released by the microbiome, already known as potential risk factors for AR, as in the case of 1-octen-3-ol [59], and for asthma [60].
Regarding microbiome contaminants, also indoor bacteria might contribute to the direct and indirect effects of IAP on atopic diseases. A Chinese study conducted on preschool children highlighted a positive association between Collinsella and Cutibacterium from actinobacteria and rhinitis and eczema, respectively. Curiously, the increase in annual average of outdoor PM10 and NO2 was positively related to the indoor presence of Prevotella, which in turn was associated with a protective effect on rhinitis, together with Lactobacillus iners and Dolosigranulum from Firmicutes [58].
Following, a summarizing table (Table 1) describing their effects on atopic diseases is reported.
Effects of indoor pollutants on atopic diseases
Pollutants | Effects on asthma, AR, and AD |
---|---|
PM | Asthma:
AR:
AD: |
VOCs | Asthma and AR:
AD: |
Heavy metals | Asthma:
|
Chemical gases | Asthma and AR:
AD: |
Molds | Asthma:
|
AD: atopic dermatitis; AR: allergic rhinitis; CCL2: C-C motif ligand 2; CO: carbon monoxide; FeNO: fraction of exhaled nitric oxide; FEV1: forced expiratory volume in the first second; FVC: forced vital capacity; GM-CSF: granulocyte-macrophage colony-stimulating factor; ILC2: type 2 innate lymphoid cells; MCP-1: monocyte chemotactic protein-1; MIP-3: macrophage inflammatory protein-3; MMEF: maximal mid-expiratory flow; NO2: nitrogen dioxide; O3: ozone; PM: particulate matter; ROS: reactive oxygen species; SCORAD: scoring AD; SO2: sulfur dioxide; Th2: T helper 2; TSLP: thymic stromal lymphopoietin; VOCs: volatile organic compounds
Main sources of indoor pollution
As already stated, the main sources of pollutants include cooking, heating, cigarette smoke, carpets and building materials, furniture, office equipment, and even mold, pests, and pets [61–63]. Moreover, outdoor pollutants may enter due to ventilation and cracks in the building envelope [64].
Tobacco and electronic cigarettes smoke
In the context of IAP, ETS is an important contributor, containing PM, oxidative gases, heavy metals, and at least 50 carcinogens [65]. A strong body of evidence relates the exposure to ETS with both asthma onset and worse control. Bentouhami et al. [66] observed a strong association between the occurrence of a first diagnosis of asthma and recent exposure to ETS. A systematic review and meta-analysis found that children with asthma exposed to ETS are twice as likely to be hospitalized for asthma exacerbations and are more likely to have lower pulmonary function test results [67]. Another systematic review conducted on 79 prospective studies found that pre- and post-natal ETS exposure is associated with a 30–70% increased risk of developing wheezing and a 21–85% increase in the risk of developing asthma [68]. Such a risk has also been confirmed among adults, as stated in a study performed in South Finland on the working-age population. Researchers found that the probability of developing asthma was significantly related to total and workplace ETS exposure during the previous 12 months [69]. A possible explanation of the effect of tobacco smoke on asthma onset is that it upregulates airway mucus production, impairs mucociliary clearance, and induces low-grade inflammation within the lungs, collectively changing the micro-environmental conditions and eventually promoting airway remodeling [70]. Curiously, exposure to ETS has been related to a slightly lower risk of seasonal AR [71]. On the opposite, prenatal exposure was reported as linked to reduced regulatory T-cell activity with consequent increased AD development after birth [72].
In the last years, electronic cigarettes (e-cigarettes) have been perceived as a safer alternative to conventional tobacco, especially among adolescents. E-cigarette liquids and aerosols contain propylene glycol, vegetable glycerin, nicotine, and aldehyde used as flavoring agents [73]. Propylene glycol and vegetable glycerin, when heated, result in the formation of formaldehyde and acetaldehyde [74]. Recent data revealed that adolescents with asthma are more likely to use e-cigarettes compared to their non-asthmatic peers (12.4% vs. 10.2%) and that current users have an increased prevalence of asthma and wheezing, even in the case of second-hand exposure [75–77]. Kotoulas et al. [78] found that pulmonary function in asthmatic patients (measured as FEV1/FVC ratio) rapidly decreased after vaping, and FeNO measured 30 min after e-cigarette use increased by 3.60 ppb.
Heating and cooking devices
During combustion, a wide variety of pollutants can be released from fireplaces or stoves, such as PM2.5, PM10, ultrafine PM, O3, CO, and SO2 [3]. Household sources include both heating devices (e.g., fireplaces, woodstoves, kerosene heaters, and flued or non-flued gas heaters) and gas stoves for cooking. Exposure to indoor combustion sources appears to increase the risk of asthma or asthma severity, particularly in children [79]. In general, the risk due to indoor combustion was more consistently associated with heating than cooking, probably due to a minor period of exposure for the latter [79]. Regarding cooking fuels, both biomass and liquefied petroleum gas were found related to the risk of asthma [19].
Building materials and furniture
Building materials and furniture are relevant sources of VOCs, chemical gases, and heavy metals. A study by Saif et al. [46] found that asthmatic children exposed to paint were 10 times more likely to experience an asthma attack than unexposed asthmatic children. Further studies conducted on school students in northern China reported that recent indoor painting was associated with a two-time higher prevalence of wheezing, nocturnal asthmatic symptoms [80], and daytime attacks of breathlessness [81]. Furthermore, recently painted surfaces indoors, new furniture, and floorboards were also found to be more prevalent in the houses of asthmatic patients, even if the difference was not significant [36]. In addition, also wallpaper emits IAPs, including VOCs that can damage the epidermal barrier with consequent exacerbation, for instance, of the adverse effects of HDMs in patients with AD. A Korean study reported an improvement in the SCORAD index in children living in houses with environmentally friendly wallpaper [polyvinyl chloride (PVC)-free] compared to PVC wallpaper exposure [82].
Following is a small summary of the effects of IAP on atopic diseases based on the main sources. At the end of this section, the different indoor pollutants and their relative sources are listed in Table 2.
Characteristics of main indoor pollutants and their sources
Pollutants | Characteristics | Sources |
---|---|---|
PM | The mixture of small particles and droplets consisting of organic chemicals, metals, and dust particles Categorized by its size:
≥ 2.5 μm PM affects mainly high airways; < 0.1 μm PM affects small airways and is eventually trans-located into circulation |
|
VOCs | Organic chemicals that easily vaporize and spread in the environment under normal conditions (high vapor pressure at room temperature and standard atmospheric pressure; initial boiling point < 250°C)Highly volatile, mobile, and resistant to degradation (able to be transported to long distances in the environment)Most abundant: formaldehyde, benzenes, toluene, ethylbenzene, and xylenes |
|
Heavy metals | They can enter the human body through inhalation, ingestion, or dermal contact |
|
Chemical gases | SO2, NO2, and CO are products of combustionO3 is produced in the atmosphere by oxidation of hydrocarbons and CO catalyzed by hydrogen oxide radicals and nitrogen oxide radicals |
|
Biological contaminants | Bacteria, viruses, unicellular organisms, molds, algae, HDMs, pests, and animal epitheliaTemperature and relative humidity are important factors that favor the growth and survival (fungi and dust mites) | Humans, carpets, stuffed toys, cushions, and pets |
CO: carbon monoxide; DEPs: diesel exhaust particles; e-cigarettes: electronic cigarettes; HDMs: house dust mites; NO2: nitrogen dioxide; O3: ozone; PM: particulate matter; SO2: sulfur dioxide; VOCs: volatile organic compounds
Indoor air quality control
The more restricting regulations aiming at the avoidance of energy dispersion led to more air-tight constructions, with a consequent worsening of the air quality in some buildings [83]. One of the main mechanisms to control the concentration of indoor pollutants is represented by the proper air distribution through ventilation, which is useful to dilute gaseous contaminants and airborne particulates, but also to control internal humidity. Different ventilation systems may be affected by different limits: if mechanical ventilation (i.e., fans or blowers) can increase energy consumption, on the other hand, natural systems may sometimes reveal insufficient. In this perspective, hybrid ventilation may represent a good compromise [3].
The elimination of sources of moisture, such as intrusion, leaks, and condensation due to poor insulation can decrease the proliferation of molds and other microorganisms dampness-dependent. The use of dehumidifiers can be useful too. A systematic review of moisture control that included 12 studies with 8,028 total participants found moderate-quality evidence regarding decreased asthma‐related symptoms in adults when interventions, including moisture control of fungus-contaminated houses and offices, were made [84, 85].
Regarding human activities, simple interventions, such as using a hood while cooking can significantly decrease exposure to PM. A study conducted in Northern California found that not using a hood fan was correlated with a higher percentage of time of exposure to very high PM2.5 levels [86].
Moreover, using furniture made of materials releasing lower amounts of VOCs is also important. For instance, furniture made using fiberboard has a lower emission of VOCs compared to those made in particleboard. The emission of VOCs depends also on the type of surface finishing method. Water-based paint and ultraviolet paint release fewer VOCs compared to PVC and low-pressure melamine-impregnated paper [87]. In addition, the indoor concentrations of VOCs in new apartments decreased over time over a 24-month period after the occupants had moved in [88].
Limitations of the review
Given the narrative nature of this review, observational studies (and whenever available case-control trials) about the effects of IAP on asthma, AR, and AD were included. Unfortunately, the literature about this argument is very dispersive. Due to the large number of molecules and compounds identifiable as indoor pollutants, their spread, the numerosity of their sources, and their geographic/socioeconomic variation, many considered studies did not report the effects according to single components of IAP, but rather to mutable and heterogeneous groups. Moreover, due to this wide variety, standardization in methods and a comparison of investigated outcomes were difficult to achieve. In this perspective, whenever possible, priority was given to studies focusing on specific categories (i.e., PM, VOCs, heavy metals, etc.) or specific indoor pollutants (i.e., PM2.5, toluene, lead, etc.), with particular regard for those reporting outcomes objectively measured by clinically validated tools (i.e., FEV1/FVC ratio for asthma and SCORAD index for AD), rather than by subjective reports.
Conclusions
IAP is an important problem concerning human health and causing millions of deaths worldwide each year. Common air pollutants, including PM, VOCs, chemical gases, heavy metals, and biological contaminants have an important influence on atopic diseases such as asthma, AR, and AD, being implicated in increasing the risk of their development and severity. The sources of IAP are various and widespread all over the household (Figure 1). The application of targeted adjustments, such as the avoidance of cigarette smoking, the choice of adequate ventilation systems and low-emitting building materials and furniture, and the use of hoods while cooking, may significantly limit the exposure to toxic substances, improving the quality of life of indoor occupants.
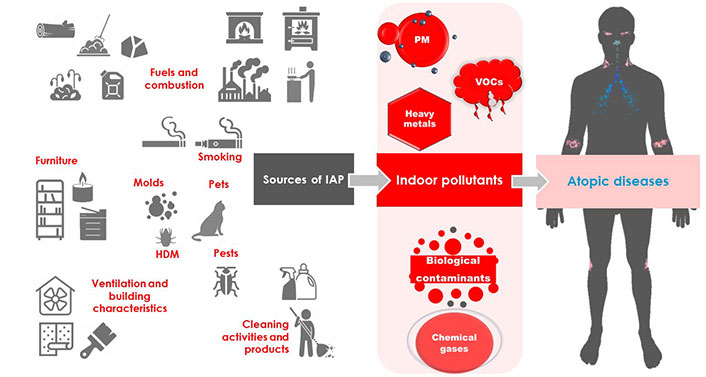
Indoor pollutants originate from different sources and may be categorized as PM (i.e., PM10, PM2.5, and ultrafine PM), VOCs (i.e., formaldehyde, toluene, etc.), heavy metals (i.e., lead, etc.), biological contaminants (i.e., molds, animal epithelia, HDM, and pests), and chemical gases [i.e., sulfur dioxide (SO2), nitrogen dioxide (NO2), ozone (O3), etc.]. The sources of indoor pollutants are different and, in some cases, may promote negative effects, leading to the onset or the worsening of asthma, allergic rhinitis (AR), and atopic dermatitis (AD). HDM: house dust mite; PM: particulate matter; VOCs: volatile organic compounds
Abbreviations
AD: | atopic dermatitis |
AR: | allergic rhinitis |
CO: | carbon monoxide |
DEPs: | diesel exhaust particles |
e-cigarettes: | electronic cigarettes |
ETS: | environmental tobacco smoke |
FeNO: | fraction of exhaled nitric oxide |
FEV1: | forced expiratory volume in the first second |
FVC: | forced vital capacity |
IAP: | indoor air pollution |
NO2: | nitrogen dioxide |
O3: | ozone |
PM: | particulate matter |
ppb: | part per billion |
PVC: | polyvinyl chloride |
SCORAD: | scoring atopic dermatitis |
SO2: | sulfur dioxide |
Th2: | T helper 2 |
VOCs: | volatile organic compounds |
Declarations
Author contributions
ER: Conceptualization, Supervision. AP: Investigation, Writing—original draft. AB, MO, and MC: Investigation. FN: Conceptualization, Investigation, Writing—original draft, Writing—review & editing, Visualization. All authors read and approved the submitted version.
Conflicts of interest
ER is a member of the editorial board of Exploration of Asthma & Allergy journal and had no involvement in the decision-making or the review process of this manuscript. All the other authors declare that they have no conflicts of interest.
Ethical approval
Not applicable.
Consent to participate
Not applicable.
Consent to publication
Not applicable.
Availability of data and materials
Not applicable.
Funding
Not applicable.
Copyright
© The Author(s) 2024.