Abstract
Aim:
This work aimed to evaluate the antiproliferative activity of silver nanoparticles (AgNPs) biosynthesized with aqueous extract of Stenocereus queretaroensis peel (SAgNPs) in pancreatic ductal cancer cells PANC-1.
Methods:
Nanoparticles were synthesized using 2 mM silver nitrate (AgNO3) and a 1% aqueous extract of Stenocereus queretaroensis peel. SAgNPs were characterized by ultraviolet-visible spectroscopy (UV-Vis) light spectroscopy, dynamic light scattering analysis, and transmission electron microscopy. The antiproliferative activity was evaluated in the PANC-1 cell line by measuring the viability percentage with the 3'-(4,5-dimethylthiazol-2-yl)-2,5-diphenyl tetrazolium bromide (MTT) method and subsequently the IC50 of SAgNPs.
Results:
The presence of AgNPs was confirmed by silver surface plasmon resonance at 420 nm. The average size obtained by dynamic light scattering analysis was 98.96 nm, with a spherical and uniform shape according to transmission electron microscopy analysis. SAgNPs were tested at concentrations from 10 µg/mL to 0.3125 µg/mL and presented inhibition percentages from 3.76% to 90.09% with an IC50 value of 3.04 µg/mL (p-value of 0.02, 95% confidence level) in PANC-1 cells.
Conclusions:
The biologically synthesized nanoparticles with Stenocereus queretaroensis peel showed antiproliferative activity in PANC-1 pancreatic cancer cells. Therefore, these results suggest their potential use in the prevention and treatment of pancreatic cancer with further investigation.
Keywords
Silver nanoparticles, biogenic synthesis, antiproliferative activity, pancreatic cancer, Stenocereus queretaroensisIntroduction
Pancreatic cancer is one of the most aggressive cancers, characterized by a five-year survival rate of less than 9% and a generally poor prognosis due to its high metastasis. Furthermore, alterations in cellular metabolism, including glucose, amino acid, fatty acid, and lipid metabolism, facilitate the survival of this cancer, making it very difficult to treat [1].
Nanotechnology has seen significant advancements in various applications, both industrial and the biomedical areas. This technology offers the possibility of treating multiple diseases [2].
Concerning cancer treatment, one of these nanomaterials, silver nanoparticles (AgNPs), has recently been proposed as an alternative for eliminating tumor cells due to its unique properties. Among the advantages these materials offer is their ability to cross biological barriers, such as the skin, gastrointestinal and respiratory mucous membranes, and the blood-brain barrier. In addition, they can be specifically targeted to an organ, tissue, or group of cells. AgNPs have multiple cellular targets that vary among cell types. Some authors suggest cytoskeleton deformations that cause chromosomal aberrations and ultimately inhibit cell proliferation [3]. It has also been seen that AgNPs, either by accumulation in the surrounding medium or through cellular endocytosis, induce toxicity in cancer cell lines [4, 5].
Biological synthesis, also called green synthesis, can be carried out through extracts from various plants since they contain different compounds that can act as silver reducers. This synthesis method, in addition to being cost-effective and having a lower environmental impact, uses a wide variety of compounds present in plants and allows the use of phenolic compounds and flavonoids present in plant residues, which can be used as part of the strategy of 203 sustainable development goals [6].
In this context, Stenocereus queretaroensis is a plant with wide distribution in Mexico, its fruit, commonly known as pitaya, has been consumed by the population since pre-colonial times. Phytochemical analysis of S. queretaroensis peel, a waste product shows the presence of phenolic compounds and flavonoids that can act as reducers for silver nitrate (AgNO3) and previously have been used for the synthesis of SAgNPs with demonstrated antimicrobial activity [7]. To our knowledge, there are no known reports about the effect of SAgNPs on pancreatic tumoral cells. The objective of this work was to evaluate the antiproliferative activity of SAgNPs biosynthesized with S. queretaroensis in PANC-1 pancreatic cancer cells (ATCC CRL-1469).
Materials and methods
Plant extract
The extract was made from the peel of pitaya (S. queretaroensis) acquired in a market in Guadalajara, Jalisco, which was treated according to the methodology reported by Loo et al. [8]. The pitaya peels were dried at 80°C for 5 days. They were then crushed into a fine powder. The aqueous extract was performed at 1% and 95°C for 10 min, keeping it boiling.
Biosynthesis of AgNPs with S. queretaroensis fruit peel
The SAgNPs were synthesized by mixing a solution of AgNO3 (2 mM) with S. queretaroensis fruit peel extract, the pH was adjusted to 8 with NaOH in a ratio of 1:20 of silver extract-nitrate and temperature of 90°C for 30 min with agitation, and after that, it was allowed to cool to room temperature with a reaction time of 24 hours. The formation of colloidal SAgNPs was perceived by the change of color to reddish brown. Subsequently, the mixture obtained was centrifuged at 10,000 rpm for 30 min at 4°C and the supernatant was discarded. The pellet was washed to remove impurities with distilled water and finally, it was dried in an oven at 90°C to obtain SAgNPs [7, 9].
Characterization of SAgNPs
There are several analytical techniques to characterize nanoparticles like X-ray diffraction, Fourier transform infrared spectroscopy, scanning electron microscopy, atomic force microscopy, and Raman spectroscopy, however, we decided to use the most representative ones.
Ultraviolet-visible spectroscopy
The reaction that occurred with AgNO3 and the aqueous peel extract was determined with the help of an ultraviolet-visible spectroscopy (UV-Vis) microplate spectrophotometer (Bio-Rad), loading the samples (extract, AgNO3, SAgNPs) in a plate of 96 wells, performing a scan of 300 nm to 700 nm [10].
Dynamic light scattering
The size of SAgNPs was obtained through a dynamic light scattering (DLS) study in a Zetasizer (Nano ZS, Malvern), the measurement was made in triplicate at 25°C [7].
Transmission electron microscopy
The size and morphology distribution of the biosynthesized AgNPs were evaluated by transmission electron microscopy (TEM) using a TECNAI G20 (FEI Company) instrument, operating at an acceleration voltage of 200 kV. The electron micrographs were acquired with an Eagle 4k × 4k CCD camera (FEI Company), at nominal magnifications of 11,500×, 50,000×, and 62,000× [7, 11].
Cell culture
Antiproliferative activity PANC-1 is a pancreatic epithelial carcinoma cell line, originally obtained from the pancreatic duct of a 56-year-old Caucasian male patient diagnosed with epithelioid carcinoma, was acquired from the American Type Culture Collection codified as ATCC CRL-1469 and kept under standard conditions for cell culture. The supplier of cell line grants a certificate with the analysis information of possible contamination with bacteria, fungi, and mycoplasma, as well as short tandem repeat (STR) profiling. The proliferation of the PANC-1 cell line was carried out with supplemented medium Dulbecco’s modified Eagle’s medium (DMEM) + 10% fetal bovine serum + 1% glutamine + 1% streptomycin, in an incubator at 37°C and 5% CO2. Once a confluence of 98% was reached, 3'-(4,5-dimethylthiazol-2-yl)-2,5-diphenyl tetrazolium bromide (MTT) tests were carried out for cell viability [12].
For the MTT assay, 96-well plate seeding was carried out at a ratio of 20,000 PANC-1 cells/well. After 24 hours of incubation in the plate, the different concentrations of nanoparticles (from 10 µg/mL to 0.3125 µg/mL) and the control (Triton X-100, Sigma-Aldrich Co) were tested for another 24 hours at 37°C. The cells were washed with salt phosphate buffer (PBS 1X, Sigma-Aldrich Co), to replace the medium with a 10% MTT solution from the MTT stock solution. After 4 hours the MTT was removed, and the plate was stored in darkness for 24 hours. Subsequently, dimethyl sulfoxide (DMSO, Sigma-Aldrich Co) was used to dissolve the crystals formed and the plate was read at 570 nm in a plate reader. The experiments were conducted in triplicate and expressed as the average, with the standard deviation calculated thereafter [13].
Results
Synthesis and characterization of nanoparticles
Figure 1 shows the results of the color change in the reaction of extract with AgNO3, where the reaction mechanism between the aqueous extract of S. queretaroensis peel, with the AgNO3 solution, formed an initial mixture of light-yellow color and ending with a dark reddish-brown color (after 24 h), consequently, from the reduction of ionic silver to reduced silver, obtaining a synthesis of SAgNPs.
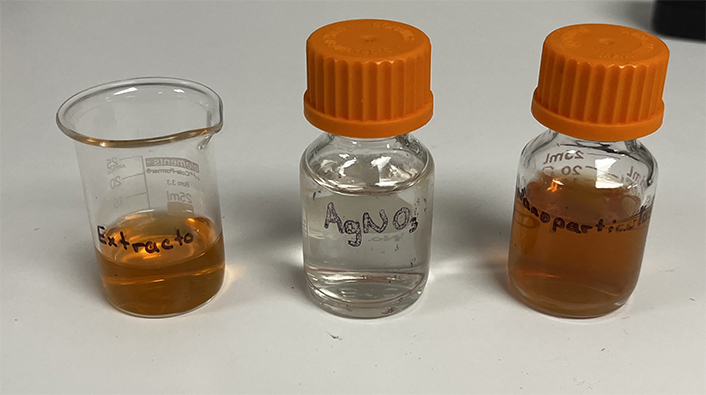
Synthesis of nanoparticles. From left to right, aqueous extract of Stenocereus queretaroensis, silver nitrate (AgNO3), and biosynthesized SAgNPs
Figure 2 shows the spectroscopy results. The biosynthesized SAgNPs had a peak at 420 nm, compared to the AgNO3 solution and the extract, which showed a descending behavior progressively decreasing the absorbance as the wavelength increases, the difference between the spectrum of nitrate and extract is the site where the extract’s readings begin since this at a wavelength of 300–380 nm shows absorbances that come out of the linearity of the method. On the other hand, biosynthesized SAgNPs show an upward curve after 340 nm, whose maximum peak is at 420 nm. The UV absorbance peak around 400–500 nm was a distinct property of silver nanoparticles [14].
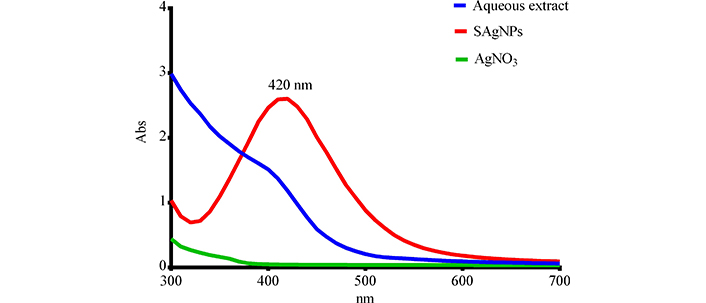
Spectrophotometric analysis of nanoparticles. Abs: absorbance. SAgNPs refer to Stenocereus queretaroensis peel nanoparticles and AgNO3 refers to silver nitrate
Figure 3 represents the size of SAgNPs with a DLS analysis, which showed an average distribution size of 98.96 nm with different peaks in the range of 40 nm to 400 nm. DLS could overestimate the size when compared to TEM because DLS measures the hydrodynamic size of SAgNPs [15]. With TEM, it was demonstrated that the morphology of nanoparticles is variable spherical without large visible agglomerations.
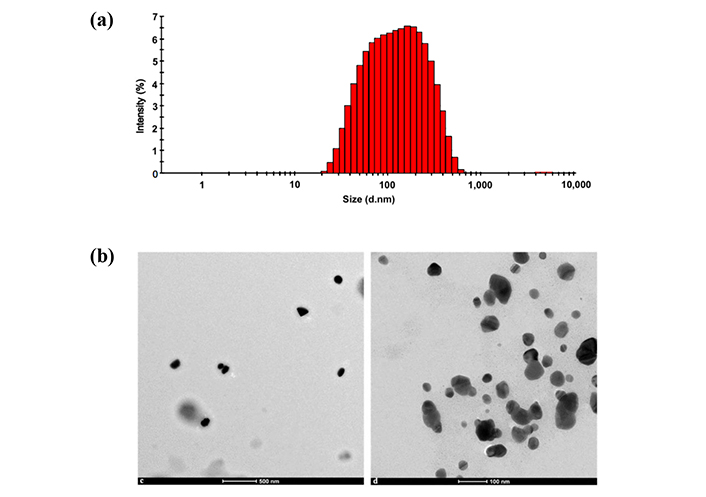
Characterization of nanoparticles. Particle size analysis in Zetasizer (a) and two TEM photographs of nanoparticles (b). These data were obtained from a previously published work by us “Biosynthesis of Silver Nanoparticles Using Stenocereus queretaroensis Fruit Peel Extract: Study of Antimicrobial Activity”. Adapted from [7], © 2021 by the authors. CC BY 4.0
Cytotoxicity assay
From the graph of the in vitro assay (Figure 4), an inverse relationship is observed between the concentration tested and cell viability in the PANC-1 cell line by the MTT method using triton as a control. As the concentration of SAgNPs decreases, cell viability increases, with evaluated values from 10 μg/mL to 0.3125 μg/mL. This indicates that SAgNPs have antiproliferative activity at these concentrations, decreasing the viability of PANC-1 cells. This behavior has a dose-dependent effect.
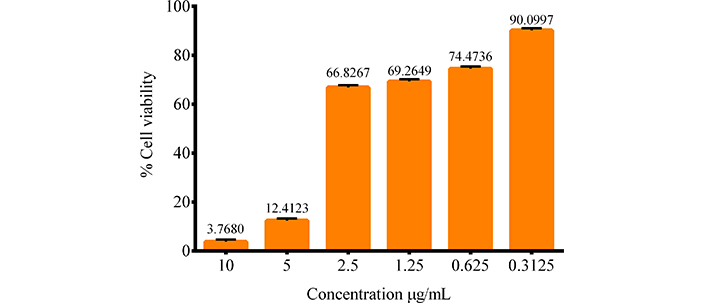
Antiproliferative activity of PANC-1. The effect of different concentrations of SAgNPs on cell viability is observed
The IC50 (inhibitory concentration 50) of the substance was determined by the Statgraphics software version XVI, and the value obtained is 3.04 μg/mL 0.02 p-value. 95% confidence level with chi-square goodness-of-fit test. This value represents the concentration needed to reduce cell viability to 50%, indicating that the substance is effective in inhibiting cell growth at low concentrations.
Discussion
The biosynthesis, as mentioned in the results, showed a color change from transparent to dark brown, which has previously been associated with the reduction of silver ions [16, 17]. These color changes and their intensity have also been associated with a higher concentration of nanoparticles by other authors [18]. The presence of a peak at 420 nm in the spectroscopy analysis shows that an absorbance peak between 400–500 nm is confirmatory and has been previously reported for AgNPs [16].
The DLS analysis showed a particle size of 98.96 nm. Depending on nanoparticle size, their biodistribution in different organs can increase or decrease. For example, nanoparticles smaller than 30 nm have of greater distribution than AgNPs of 100 nm [19]. Additionally, the spherical shape observed in the TEM analysis may be linked to specific biological activity. However, a limitation of this study is that the effect of the variation in the size of the nanoparticles shown in the DLS study was not considered. In summary, the size and shape of AgNPs biosynthesized in this study could serve as predictors for future research on their biological activity [20, 21].
The cytotoxicity results of SAgNPs show low cell viability, when comparing these values obtained with the cytotoxicity results of the S. queretaroensis extract in a pancreatic cancer cell line MIA PaCa-2 in another study by González-Garibay et al. [22], we can observe high viability of the extract about AgNPs of the same origin as the one reported here, presenting a similar effect expected from the extract for this study due to the number of similarities with González-Garibay et al. [22].
In the case of cytotoxicity of SAgNPs, the IC50 value found at 3.04 μg/mL is lower than that reported in other studies, as in that of Balkrishna et al. [23] which used AgNPs from Putranjiva roxburghii extract obtaining an IC50 value of 360 μg/mL, or the study of Guo et al. [24] whose AgNPs biosynthesized with Berberis thunbergii showed an IC50 value of 259 μg/mL, or the work of An et al. [25] with AgNPs from Ocimum basilicum leaves with an IC50 of 154 μg/mL in the same cell line, however, we can also find IC50 values lower than those found in our study such as in the case of George et al. [26] with an IC50 value of 0.49 μg/mL. These results demonstrate that SAgNPs have a higher cytotoxic activity than those reported in other works with plant extracts. These differences in IC50 could be attributed to the variety of nanoparticle sizes, since if we compare these results with those of Zielinska et al. [27], who investigated the activity of purchased AgNPs as a function of their size and concentration in PANC-1 and hTERT-HPNE cells, we observed in the case of PANC-1, AgNPs of 2.6 nm size with 1.67 μg/mL of IC50 and AgNPs of 18 nm with 26.81 μg/mL of IC50. We concluded that the smaller nanoparticles of 2.6 nm showed greater antiproliferative activity than the AgNPs of 18 nm. However, in our investigation, we found that this relationship between effectiveness and size does not apply to all cases. Since our 98.96 nm SAgNPs with IC50 of 3.04 μg/mL have a similar value to that of Zielinska et al. [27] for their smallest AgNPs (2.6 nm = 1.67 μg/mL). This is evident when comparing the aforementioned 18 nm AgNPs with IC50 of 26.81 μg/mL, with those of Wang et al. [28] 12.27 nm with an IC50 of 312 μg/mL in the same cell line, the variation in nanoparticle effectiveness found for SAgNPs. These discrepancies in effectiveness could be due to the presence of compounds from the extract in the nanoparticles, influencing not only cytotoxicity but also other biological activities, as Wang et al. [28] also observed that, by combining these nanoparticles with their extract, there was a significant increase in the pancreatic antioxidant and anticancer effect.
That is, our findings suggest that the relationship between the size of AgNPs and their effectiveness is not absolute and that other factors, such as the presence of other compounds, may play a crucial role in the biological activity of AgNPs.
The determining factors for the toxicity of AgNPs can be divided into endogenous and exogenous, the difference between whether one factor or the other applies has to do with the size and shape of these. As mentioned earlier, the size of the SAgNPs in this study was large (98.96 nm). Consequently, transport into cells occurs through ion channels, which, in a biological model, could affect the number of organs impacted. Smaller nanoparticles would have an easier time reaching target organs. Although a good cytotoxicity result is observed in pancreatic cancer cells, size could represent a problem when applied to in vivo studies [29].
Even so, the uptake of AgNPs by PANC-1 cells can be proposed as a mechanism of action, as reported by Barcińska et al. [30], which can occur in the cytosol. In their study, they revealed that changes in biochemical parameters caused by AgNPs were related to ultrastructural alterations in pancreatic cancer cells, attributable to oxidative stress. Such alterations included mitochondrial swelling, crest degradation, mitochondrial contraction and condensation, and nuclear condensation. These effects are characteristic of oxidative stress-induced apoptosis in in vitro models.
Similar to the data reported by Kummara et al. [31] where they performed a chemical synthesis with a nanoparticle size of 104 nm and a green synthesis with a size of 94 nm, where the activity of green AgNPs induced significant and selective cytotoxicity in cancer cells demonstrating an excellent induction of cellular apoptosis in cells and less toxic against human red blood cells (healthy cells), unlike chemical AgNPs where it is shown to have significant toxicity in cancer cells and normal cells, so they conclude that green AgNPs are more effective and safer. Another possible mechanism is that the presence of low doses of AgNPs over a prolonged period could cause chronic toxicity in many cell lines [29].
The characteristics previously described compared with Barcińska et al. [30] and Kummara et al. [31] coincide with the observations made in our in vitro assay using the MTT method in our research with AgNPs with the aqueous extract of S. queretaroensis in PANC-1 cells.
AgNPs were synthesized with an ecological approach with S. queretaroensis peel extract, shown to be a reducing and coating agent.
AgNPs with the aqueous extract of S. queretaroensis caused a dose-dependent decrease in cell viability in PANC-1 cells. To elucidate the mechanism by which the antiproliferative effect occurs, assays such as apoptosis induction and cell cycle analysis should be used. The stability of biosynthesized AgNPs is an important matter to consider and a limitation of this study because preliminary studies conducted by our working group suggest that after one to two weeks the nanoparticles begin to aggregate. Another aspect that must be considered is the safety of the use of metal nanoparticles; this may be a limitation of this study. However, our working group has carried out toxicological evaluations of SAgNPs where the absence of negative effects in animal models is evident [32]. Biocompatibility and predictability to modify these AgNPs could be a viable future option in applications for personalized cancer treatment. However, future research is not ruled out to propose models where the long-term effects of AgNPs in humans are evaluated.
Abbreviations
AgNO3: | silver nitrate |
AgNPs: | silver nanoparticles |
DLS: | dynamic light scattering |
IC50: | inhibitory concentration 50 |
MTT: | 3'-(4,5-dimethylthiazol-2-yl)-2,5-diphenyltetrazolium bromide |
SAgNPs: | silver nanoparticles biosynthesized with aqueous extract of Stenocereus queretaroensis peel |
TEM: | transmission electron microscopy |
Declarations
Author contributions
AAVA and IMSH: Conceptualization, Investigation, Formal analysis, Writing—original draft. EPC: Conceptualization, Investigation, Formal analysis, Writing—review & editing, Visualization, Supervision. All authors read and approved the submitted version.
Conflicts of interest
The authors declare that they have no conflicts of interest.
Ethical approval
Not applicable.
Consent to participate
Not applicable.
Consent to publication
Not applicable.
Availability of data and materials
The data underlying this article will be shared on reasonable request from the corresponding author.
Funding
Not applicable.
Copyright
© The Author(s) 2025.
Publisher’s note
Open Exploration maintains a neutral stance on jurisdictional claims in published institutional affiliations and maps. All opinions expressed in this article are the personal views of the author(s) and do not represent the stance of the editorial team or the publisher.