Abstract
Left ventricular (LV) function is typically evaluated through LV ejection fraction (EF), a robust indicator of risk, showing a nonlinear increase in mortality rates below 40%. Conversely, excessively high EF values (> 65%) also correlate with elevated mortality, following a U-shaped curve, with its nadir observed between 50% and 65%. This underscores the necessity for improved identification of the hypercontractile phenotype. However, EF is not synonymous with LV contraction function, as it can fluctuate independently of contractility due to variations in afterload, preload, heart rate, and ventricular-arterial coupling. Assessing the contractile status of the LV requires more specific metrics, such as LV elastance (or contractile force) and global longitudinal strain. Current guidelines outline various parameters for a more precise characterization of LV contractility, yet further research is warranted for validation. The true hypercontractile phenotype is evident in cardiac pathologies such as hypertrophic cardiomyopathy, ischemia with angiographically normal coronary arteries, Tako-tsubo syndrome, heart failure with preserved EF, and may also stem from systemic disorders including anemia, hyperthyroidism, liver, kidney, or pulmonary diseases. The hypercontractile phenotype constitutes a distinctive hemodynamic substrate underlying clinical manifestations such as angina, dyspnea, or arrhythmias, presenting a target for intervention through beta-blockers or specific cardiac myosin inhibitors. While EF remains pivotal for clinical classification, risk stratification, and therapeutic decision-making, integrating it with other indices of LV function can enhance the characterization of the hypercontractile phenotype.
Keywords
Left ventricle, contractility, supernormal, cardiac function, echocardiographyIntroduction
Over five decades ago, Gorlin et al. [1] introduced the “hyperkinetic heart syndrome”, delineating a new clinical entity marked by an unexplained primary elevation (in contrast to all conceivable secondary causes) in stroke volume (SV), cardiac output (CO), pulse pressure, and left ventricular (LV) ejection rate. This syndrome, observed predominantly in young, mostly asymptomatic individuals, also exhibited mild systolic hypertension, a positive cold pressor test, and heightened oxygen consumption [1–4]. The authors ascribed the “hyperkinetic heart syndrome” to a “defect in central (neurohumoral) regulation of cardiac output”. Later, they concluded that the common denominator in the syndrome appears to be an increased ejection rate by the heart, rather than an increased CO. The characteristics of the ejection rate and the arterial pressure pulse indicate a greatly enhanced vigor of contraction, regardless of minute output [2].
Animal experiments discovered that in vivo, the inbred hypertrophic heart rat exhibits pronounced diastolic dysfunction while maintaining relatively preserved systolic function. Surprisingly, in vitro cardiomyocyte functional studies of Ca2+ handling and shortening performance revealed a hypercontractile state underlying the in vivo diastolic dysfunction. Additional notable features include focal and relatively discrete areas of interstitial fibrosis, as well as an in vivo susceptibility to dysrhythmia and sudden death. Preservation of LV ejection fraction (EF) in hypertrophic heart rat is achieved through hypercontractility, compensating for cardiomyocyte deficit and structural slippage at fibrotic foci. This hypercontractility likely stems from a significant increase in the density of the L-type Ca2+ channel [5]. Researchers have also found that hyperdynamic contraction and impaired relaxation in young hypertrophic cardiomyopathy (HCM) patients with gene mutations. This hypercontractility and blunted contractile reserve were associated downregulation of sarcoplasmic reticulum Ca2+-ATPase (SERCA-2) mRNA, resulting in altered calcium handling [6]. In brief, hyperdynamic contraction of LV usually stems from cardiomyocyte hypercontractility through Ca2+ handling.
In recent years, an increasing number of studies proposed a concept of “supra-normal LVEF”, which is, to some extent, analogous to the concept of “hypercontractile phenotype” (HYPER-Phen) [7–9]. To date, there is no uniform definition of HYPER-Phen.
Pathophysiologic basis: EF is not synonymous with LV contractile function
The primary and most widely used approach to evaluate LV systolic function in clinical settings involves estimating the LVEF. This is typically derived from dimensions obtained through various methods, M-mode, 2D, 3D techniques, or cardiac magnetic resonance (CMR). LVEF, a volume ratio to assesses LV “pumping function”, expressed by ventricular ejection SV to preload [end-diastolic volume (EDV)]. Ideally, a contractility index should respond to variations in myocardial contractile status while remaining unaffected by loading conditions [10]. However, LVEF cannot be regarded as a pure indicator of LV systolic function due to its influence from LV contractility, afterload, ventricular-arterial coupling, and LV mechanical efficiency [10, 11]. Studies have demonstrated poor correlation between LVEF and changes in LV contractility when employing LV end-systolic elastance (Ees) as a load-independent measurement of cardiac contractility, particularly in cases of altered afterload [12]. For instance, normal LVEF may persist despite severely impaired LV intrinsic contractility, as observed in conditions such as sepsis, characterized by markedly reduced arterial tone [13]. Moreover, the reliability of changes in LVEF following the administration of vasoconstrictors has been questioned, as they may indicate genuine alterations in LV contractility or alternatively reveal preexisting LV dysfunction once LV afterload is corrected [13, 14]. Chronic mitral regurgitation (MR) establishes an alternative low-resistance pathway for LV ejection, elevating LVEF despite significant LV contractile dysfunction, potentially leading to misinterpretation regarding the necessity and timing of intervention [15]. Present guidelines employ a supranormal LVEF cutoff of 60% as the benchmark for LV systolic function. This threshold indicates the need for surgical intervention in cases of asymptomatic severe MR [16].
Severe aortic stenosis and other conditions with significantly increased afterload might exhibit contrasting effects. Bradycardia could potentially inflate LVEF value due to elevated SV. Conversely, decreased SV at tachycardia or irregular R-R intervals could result in an underestimate of LVEF. Occasionally, LV malperfomance might only become apparent with alterations in loading conditions at post-surgical or interventional correction, resulting in the emergence of overt LV dysfunction and congestive heart failure (HF). Consequently, LVEF primarily serves as an integrated indicator of cardiovascular performance and LV mechanical efficiency rather than a direct measure of LV contractility. Monge García et al. [17] proposed an afterload-adjusted LVEF (adjusted to Ea), which was expressed as EF*
The clinical identification of the HYPER-Phen
The linear slope of the end-systolic pressure-volume relationship (ESPVR) and preload recruitable stroke work stand out as the most reliable techniques for gauging intrinsic ventricular contractility. These methods exhibit less susceptibility to variations in loading conditions. The ESPVR slope, delineated by alterations in LV pressure relative to volume (or elastance), has been somewhat underutilized in clinical practice [18]. Sophisticated and costly technologies often excel in initial efficacy studies but frequently struggle to transition into widespread use in real-world scenarios, with genuine patients, practitioners, and challenges. Initially termed elastance by Suga et al. [19] in their groundbreaking experimental research, the end systolic pressure (ESP)/end-systolic volume (ESV) ratio, is now more commonly known as “force”, offering a simplified and preferable parameter means of assessing LV contractility [19, 20].
As mentioned earlier, LVEF, even with its intrinsic pros and cons, stands as the predominant metric for evaluating ventricular function among patients with established or suspected heart diseases. An extensive dataset has unveiled a U-shaped correlation between survival and LVEF evaluated via echocardiography in routine clinical settings, demonstrating elevated all-cause mortality rates among patients with LVEF ≥ 65%. These findings shed light on a novel phenotype distinguished by supra-normal LVEF, suggesting an overlooked entity necessitating deeper exploration [9].
HF is traditionally classified into two primary phenotypes based on LVEF: HF with reduced EF (HFrEF) and HF with preserved EF (HFpEF). In both phenotypes, CO diminishes (< 5 L/min), failing to meet metabolic demands. However, some patients display symptoms and signs of systemic or pulmonary congestion despite a high CO (> 8 L/min) or a cardiac index surpassing 4 L/min/m2, identified as high output HF [21]. The compensatory responses observed in low-output HF seem tailored to maintain arterial blood pressure [22–24], partly through an increase in systemic vascular resistance (SVR) and expansion of the blood volume. In all high-output HF scenarios where the CO is elevated, as seen in chronic severe anemia [25], chronic obstructive pulmonary disease [26], and large arteriovenous fistula [27, 28], the arterial blood pressure is concurrently jeopardized or diminished due to significant vasodilatation. The neurohormonal reaction witnessed in these high-output situations closely resemble that observed in low-output HF.
Notably, high CO patients often exhibit a hypercontractile state. However, it is essential to clarify that a HYPER-Phen does not equal to high CO states. High CO can mask LV contractile dysfunction in some clinical scenarios [21, 29]. Thus, accurately quantifying LV contractility remains a significant challenge. For instance, in the initial stages of severe MR, LV may exhibit a hypercontractility state (supra-normal LVEF), which then progresses to contractility impairment, potentially eluding detection by traditional LV function assessment methods. de Isla et al. [29] showcased that utilizing longitudinal speckle tracking, interventricular septal strain rate offers precise detection of initial abnormalities in LV contractile function [29].
Furthermore, numerous studies have shown that global longitudinal strain (GLS) is a superior prognostic marker compared to LVEF, and patients with HFpEF have significantly impaired GLS compared with normal controls [30]. However, whether GLS follows a similar U-shaped hazard ratio curve as LVEF or demonstrates a linear relationship remains unknown. Therefore, the definition of HYPER-Phen needed to be identified by a more precise parameter.
Echocardiography, whether at rest or under stress, unmask the hemodynamics of hypercontractility, both of which highlighting different features of this condition.
Rest transthoracic echocardiography
Patients presenting with chest pain or HF are currently stratified using EF, but a normal EF does not adequately discriminate between different phenotypes. Over time, we have learned that a normal EF can coexist with abnormal longitudinal function identified by subnormal values of GLS. This subset is more likely to respond effectively to therapies typically used for HFrEF. Conversely, patients with normal EF may exhibit a HYPER-Phen, which requires recognition for effective treatment. The main clinical and red flag resting hemodynamic findings leading to suspect a HYPER-Phen are summarized in Table 1 and Figure 1. This condition can be suspected with a simple resting volumetric transthoracic echocardiography (TTE) examination showing a small, hypertrophic heart with increased force and diastolic dysfunction [7]. Resting GLS showing supernormal values and Doppler showing LV outflow gradients at rest may likely help. Different kinds of LV function phenotype based on volumetric TTE are illustrated in Figure 2.
Main clinical and resting TTE features suggestive of a HYPER-Phen
Parameters | HYPER-Phen | Normocontractile phenotype |
---|---|---|
Clinical features | ||
Female | More frequent (> 70%) [31] | Less frequent (< 60%) [8, 31, 32] |
Advanced age | > 65 yrs [7, 31] | < 65 yrs [33] |
Hypertensives | > 150 mmHg [8, 33] | Normal |
Diabetes | > 35–40% [7] | < 35–40% [7, 33] |
Obesity | > 19% [7] | < 19% [7] |
BNP | High or borderline [8] | Normal or much higher than HYPER-Phen in HfrEF [33] |
Resting TTE | ||
LVMI | > 71 g/m2 | < 71 g/m2 |
Relative wall thickness | > 0.48 | < 0.37 [7, 34] |
Small EDV | < 50 mL | > 100 mL [7, 34] |
Small ESV | < 16 mL | > 30 mL [7] |
High force | > 5 mmHg/mL | 3–5 mmHg/mL [35] |
High EF | > 60–70% [7, 31] | < 60% [31] |
Arterial elastance (SBP/SV) | More frequently high (> 4) | More frequently normal (< 4) [31] |
SV | More frequently low (< 30 mL) | More frequently normal (35–50 mL) [35] |
GLS | Normal-supernormal (> 25%) | Normal-abnormal (< 20%) [36] |
LAVI | > 34 ml/m2 | < 34 ml/m2 [7] |
E wave velocity | > 94 m/s | < 62 m/s [7, 34] |
e’ | < 6 cm/s | > 10 cm/s [7] |
E/e’ | > 13 | < 7.2 [7] |
SPAP | > 48 mmHg | < 34 mmHg [7] |
BNP: Brain Natriuretic Peptide; HYPER-Phen: hypercontractile phenotype; TTE: transthoracic echocardiography; LVMI: left ventricular mass index; E: pulsed wave Doppler-derived peak flow velocity of early filling wave; EDV: end-diastolic volume; ESV: end-systolic volume; EF: ejection fraction; SBP: systolic blood pressure; SV: stroke volume; GLS: global longitudinal strain; LAVI: left atrial volume index; SPAP: systolic pulmonary artery pressure
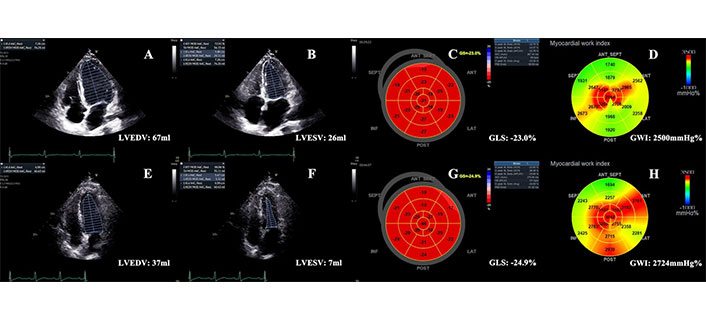
Normocontractile phenotype and hypercontractile phenotype. (A–D) A normocontractile patient with LVEF (Biplane-Simpson’s) about 61%, GLS about –23%, and global myocardial work index (GWI) of 2,500 mmHg%; (E–H) A hypercontractile patient with small LVEDV and LVESV, supranormal LVEF about 81%, high GLS about –24.9%, and also high GWI of 2,724 mmHg%. LVEDV: left ventricular end-diastolic volume; LVESV: left ventricular end-systolic volume; GLS: global longitudinal strain; LVEF: left ventricular ejection fraction
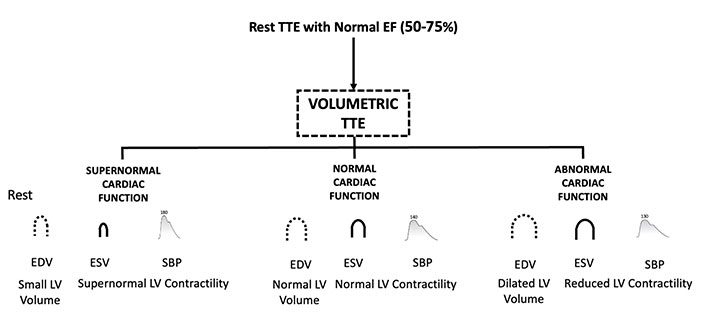
Different kinds of cardiac phenotypes based on volumetric TTE are divided according to the LV contractility. TTE: transthoracic echocardiography; EF: ejection fraction; EDV: end-diastolic volume; ESV: end-systolic volume; SBP: systolic blood pressure; LV: left ventricular
Note. Adapted from “Hemodynamic heterogeneity of reduced cardiac reserve unmasked by volumetric exercise echocardiography” by Bombardini T, Zagatina A, Ciampi Q, Arbucci R, Merlo PM, Haber DML, et al.; On Behalf Of The Stress Echo Study Group Of The Italian Society Of Cardiovascular Imaging. J Clin Med. 2021;10:2906 (https://doi.org/10.3390/jcm10132906). CC BY.
Stress echocardiography
When LV function at rest appears too good to be normal, the first consequence is that the LV has an impaired capacity to increase CO during stress (Table 2), indicating an impaired cardiac reserve. This impaired cardiac reserve can be the final common pathway of three coexistent hemodynamic mechanisms: the reduced contractile reserve (differences of contractility between stress and rest), the reduced preload reserve (increase in EDV), and the reduced chronotropic reserve (the increase in heart rate from rest to peak) [35].
Stress echocardiography | HYPER-Phen | Normocontractile phenotype |
---|---|---|
Chronotropic reserve | More frequently low (< 1.6) | More frequently normal (> 1.8) |
Preload reserve | More frequently low (stress < rest) | More normal (stress > rest) |
Inotropic reserve | More frequently low (< 1.6) | More frequently normal (> 1.8) |
Cardiac reserve | Reduced (< 2.0) | More frequently normal (> 2.0) |
B-lines ≥ 2 | More frequent | Less frequent |
LVOTO (gradient < 30 mmHg) | More frequent | Less frequent |
LVOTO: left ventricular outflow tract obstruction; HYPER-Phen: hypercontractile phenotype
The hemodynamic adaptation to the HYPER-Phen
HYPER-Phen usually demonstrates a supra-normal LVEF. According to the definition, a supra-normal LVEF can be caused either by a relatively high SV (usually occurs in case of a small ESV) or by decreased LV EDV, both of which are characterized by a reduced preload reserve and/or excessive LV afterload [31]. This hyperdynamic state often leads to LV outflow tract obstruction (LVOTO), a dynamic phenomenon defined by intraventricular peak gradient ≥ 25 mmHg identified through Doppler echocardiography. It is deemed hemodynamically significant when gradients reach ≥ 50 mmHg [37]. Typically, LVOTO fluctuates based on loading conditions. Factors such as preload and afterload, the Valsalva maneuver, drug administration, or meals can reveal or worsen intraventricular obstruction in patients without a significant resting intraventricular gradient [38, 39]. LVOTO is a characteristic feature of HCM, although the prevalence of various LVOTO causes remain incompletely explored. In a case series involving 73 patients with an intraventricular pressure gradient ≥ 50 mmHg, associated conditions included HCM (74%), hypertensive hypertrophy (9%), post-cardiac surgery (7%), sigmoid septum (4%), hyperkinetic LV (3%), Takotsubo syndrome (TTS) (1.5%), and subaortic stenosis (1.5%) [40].
A hyperdynamic state typically manifests as augmented circulatory volume and reduced SVR [41–43]. This heightened CO state may correlate with elevated heart rate and pulse pressure [21, 44, 45]. Moreover, increased CO can induce pulmonary hypertension through multiple pathways, including augmented blood flow within the pulmonary circuit [46, 47], increased LV end-diastolic pressure [46], and shear stress on the pulmonary vasculature. Consequently, this may trigger pulmonary vascular remodeling and/or vasoconstriction of pulmonary vessels due to the release of vasoactive compounds by the endothelium [47, 48]. In cases of high-output HF, systemic arterial vasodilation emerges as the primary mechanism driving compensatory CO elevation [21]. A diminished SVR results in decreased afterload and renal perfusion, prompting fluid retention and augmented circulatory volume, both of which contribute to a compensatory rise in CO [21].
To date, there’s no uniform definition of “hypercontractile phenotype”. We should bear in mind that the intrinsic hypercontractility of myocardium, supra-normal LVEF, and/or high SV/CO are all different types of manifestations for HYPER-Phen.
Current guidelines about contractility
LV pumping function served as the primary cardiac performance to maintain the body’s blood supply. Compared to per-minute LV output (CO, cardiac index), per-beat LV output (SV index) better reflects LV pumping function [49]. However, LVEF has involved into a fundamental aspect of contemporary clinical practice, serving as a robust independent prognostic factor in patients with HF and acute myocardial infarction, guiding therapeutic strategies [50, 51]. The European Association of Cardiovascular Imaging (EACVI) and the American Society of Echocardiography (ASE) suggest evaluating LVEF in 2D using a modified Simpson’s method of discs, obtaining LV volumes from apical 4- and 2-chamber views, or alternatively, when accessible and feasible, by 3D images [52]. Even with ideal imaging conditions, LVEF might provide an incomplete or inaccurate assessment of LV function, particularly in instances of significant concentric remodeling, HCM, or diminished cavity size, where substantial systolic dysfunction with decreased SV is present despite a normal or supranormal LVEF. Strain image offers a more accurate reflection of systolic function in these patients with preserved EF due to geometric factors.
Speckle tracking derived strain captures solely the active contraction of myocardial fibers, thus negating the “tethering effect” error, which involves the passive movement of fibrous tissue through adjacent viable myocardium. GLS has demonstrated greater sensitivity as an indicator of LV systolic function compared to LVEF, offering superior prognostic value across various heart diseases characterized by a broad spectrum of myocardial dysfunction [53]. This quantitative approach to assessing regional and global LV function has garnered recognition for its ability to detect LV systolic dysfunction in cardiomyopathies, cardio-oncology, or cases of cardiac remodeling where LVEF remains within normal limits. GLS delivers a more precise evaluation of systolic function in conditions featuring increased LV mass or altered LV geometry, particularly notable in HCM where severely depressed systolic function may coexist with a supranormal LVEF. The ESC (European Society of Cardiology) guidelines for diagnosis and management advocate for myocardial strain imaging in early HCM disease assessment [37]. The ASE/EACVI consensus statement incorporates a relative percentage decrease in GLS of > 15% from baseline as an indicator of cancer therapeutics-related cardiac dysfunction [54].
It’s essential to emphasize that myocardial strain is influenced by loading conditions [18]. Strain rate, representing the rate of deformation, exhibits less reliance on loading. Consequently, there’s been curiosity about employing it as an indicator of contractility. Nonetheless, reproducibility with tissue Doppler methods is constrained by angle dependency, diminished spatial resolution, and decreased signal-to-noise ratio, notwithstanding excellent temporal resolution. Alternative techniques may lack the requisite frame rate to precise quantification of deformation velocity [55]. These constraints have thus far impeded its integration into routine practice.
Data are in progress with the estimation of HYPER-Phen with LV force and stroke work. In theory, the use of force holds potential since it is a relatively pure index of contractility, less affected by afterload or preload changes than EF, and has already been shown to be more efficient than EF in stratifying prognosis in patients with reduced EF [35]. Another potentially beneficial method involves evaluating myocardial function by combining strain analysis with noninvasive estimation of LV pressure using pressure-strain loop analysis. By integrating diverse myocardial work elements like index, efficiency, and constructive and wasted work, a comprehensive evaluation of LV mechanics and energetics can be attained [56].
Indeed, normal systolic function arises from the contraction of myocardial fibers arranged in a right-handed helix within the subendocardium, a left-handed helix in the subepicardium, and a layer of circumferential fibers in between [57]. Simply put, the interaction of these double helical-oriented muscle layers induces longitudinal and circumferential shortening, alone with radial thickening, coupled with a twisting motion along the long axis during systole. Thus, a HYPER-Phen can be characterized either by viewing the LV as a unified entity (e.g., myocardial work, GLS) or as a more intricate assembly spheres (e.g., longitudinal, circumferential, radial strain, or strain rate, etc.).
The LV contractile phenotype as an actionable therapeutic target in different diseases
The hyperfunction of cardiac sarcomeric myosin, whether primary or secondary to increased sympathetic drive, presents a promising therapeutic target. For example, modulating stressed volume, targeting arterial stiffness and/or vascular load would be beneficial. This can be addressed through interventions such as cardiac myosin inhibitors and/or beta-blockers. Currently, therapeutic indications rely on suboptimal indices such as LVOTO in HCM or EF with beta-blockers. The resurgence of interest in the hypercontractile state as an actionable therapeutic target has intensified our focus on noninvasive methods for identifying this condition. As many hypercontractile states are treatable, identifying the underlying cause of hypercontraction is pivotal to determining the suitable remedy, such as identifying the characteristics of reduced preload reserve and/or excessive LV afterload, distinguishing high-CO from normal-to-decreased CO variants, and validating easily accessible parameters like force and SV based on volumetric echocardiography. Today, this is also operator-independent and highly reproducible with artificial intelligence software already on board in commercially available machines.
The development of simple-to-use and prognostically validated indices will facilitate defining their prognostic correlates in various patient subsets. It will also enable assessing the feasibility and value of echocardiography-driven therapeutic interventions. These efforts align with ongoing large-scale multicenter studies utilizing force and other indices derived from volumetric echocardiography across diverse patient subsets, ranging from chronic coronary syndromes and ischemia with non-significant coronary artery disease to HCM and HFpEF.
A common underlying hypothesis in all subprojects of SE2030 is that therapy guided by a more precise characterization of the hypercontractile state can enhance the current indications, efficacy, and safety of treatments based on imperfect biomarkers of LV contractility, such as EF or LVOT gradient. So far, there is no standardized definition for the “hypercontractile phenotype”. So, we will introduce different kinds of diseases in hypercontractile state according to various manifestations, such as the intrinsic hypercontractility of myocardium (increased afterload or decreased preload), supra-normal LVEF, and high SV/CO.
Pressure overload—hypertension
Hypertension is considered to be the most common HYPER-Phen, observable during the compensatory stage. However, in instances of hypertension with preserved or supranormal EF, there seems to be inconsistency between assessment of regional myocardial strains and global EF. CMR study have demonstrated that in compensated LV remodeling resulting from hypertension, there is augmented LV wall thickening alongside retained principal strain for maximal shortening and enhanced torsion compared to normal controls. This contributes to the supranormal LVEF observed in hypertension patients despite diminished longitudinal and circumferential strains [58]. Roughly 30% of newly diagnosed hypertension in Caucasian individuals without complications exhibited elevated levels of endogenous ouabain, a type of endogenous cardiac glycoside. This elevation correlated with decreased heart rate and increased LV mass and SV [59]. Also, in patients with advanced hypertension, the levels of circulating endogenous ouabain correlate directly with both blood pressure and total peripheral resistance [60]. Thus, targeting on endogenous cardiac glycoside might be a useful strategy [60]. In the early stage of hypercontractility, the primary approach should involve reducing pressure overload and addressing other concomitant pathophysiological factors. Most importantly, screening should focus on identifying the underlying mechanism behind EF alteration, rather than solely assessing EF itself.
Ischemic heart diseases—TTS
TTS, also recognized as stress cardiomyopathy or “broken-heart syndrome”, is marked by acute and typically transient LV dysfunction [61]. The prevailing morphological variant of TTS, termed “apical ballooning”, is characterized by widespread hypokinesia or akinesia of the apical and midventricular myocardium (circumferential pattern) alongside basal hyperkinesia. The emergence of LVOTO during the acute phase of TTS poses a complex challenge, with its severity contingent on hemodynamic status. The surge of plasmatic catecholamine creates a paradoxical scenario of mid-apical myocardial stunning and compensatory hypercontractility of the basal segments. Coupled with myocardial structural alterations, this may foster rheological conditions conducive to dynamic obstruction. Moreover, predisposing factors for LVOTO encompass a diminutive ventricular cavity and asymmetric hypertrophy of the interventricular septum (septal bulge). Beyond dynamic intraventricular obstruction, SAM (systolic anterior motion) may trigger secondary MR due to leaflet malcoaptation. Additionally, the elevated afterload associated with LVOTO sets in a vicious circle to exacerbate apical myocardial dysfunction through elevated shear stress and disruption in the oxygen supply/demand ratio. These alterations detrimentally affect CO and may synergically precipitate cardiogenic shock and pulmonary artery hypertension, contingent upon the extent of MR and left atrial compliance [62–64]. In suspected TTS cases, timely identification or exclusion of LVOTO is pivotal for directing patient care and preventing inappropriate therapies that could exacerbate hemodynamic instability.
In the absence of randomized clinical trials, there are no established guidelines to endorse specific treatment approaches in TTS. Consequently, existing recommendations draw solely from case reports, retrospective studies, and expert opinions [65, 66]. The long-term management and outcomes of TTS patients remain inadequately explored, precluding definitive evidence-based recommendation for persistent symptom management.
Ultimately, regular echocardiographic monitoring should be initiated to verify the resolution of wall motion abnormalities, intraventricular gradient, and secondary mitral valve regurgitation. The clinical profiles, long-term outcomes and management of critically ill patients with TTS and LVOTO remain inadequately explored thus far and necessitate further investigation in larger cohorts.
Hypovolemia, anesthesia-mediated vasodilatation or neuraxial anesthesia
SAM sign, causing LVOTO and MR may cause an acute fall in CO. This condition is commonly encountered in patients with cardiac disease, such as HCM, but it may occur in patients without cardiac history, triggered by absolute or relative hypovolemia and intense catecholaminergic stimulation [67–72]. While the primary factor predisposing to SAM is a hypercontractile LV, structural anomalies of the mitral valve apparatus, HCM, or reduced LV preload due to underfilling of the ventricle can also incite SAM. In the perioperative context, various hemodynamic conditions such as anesthesia-induced vasodilation (from either general anesthetics or neuraxial anesthesia), elevated catecholamine levels due to surgical stimulation or use of inotropic medications, and absolute or relative hypovolemia resulting from preoperative fasting or perioperative bleeding can all increase the risk and exacerbate the severity of SAM, even in the absence of underlying cardiac pathology [67, 71]. Recent studies suggest that the primary mechanism of SAM involves rapid blood flow velocities in the LVOT induced by LV hypercontractility, which pull the mitral valve leaflets anteriorly into the septum [73, 74]. The therapeutic approach for SAM revolves around increasing LV preload and mitigating the hypercontractile state [74, 75].
Utilizing echocardiographic as a primary diagnostic tool for SAM may prove crucial, as it enables direct visualization of the underlying mechanical pathology and aids in distinguishing it from other triggers of severe cardiopulmonary events. Consequently, prompt diagnosis prevents the administration of potentially detrimental therapies, such as diuretics, inotropes, or vasodilators.
High CO states
High CO states can occur in various systemic diseases, such as chronic severe anemia, obesity, arteriovenous fistula, pregnancy. These conditions can lead to fluid retention resistant to traditional treatment and potentially exacerbate pre-existing heart disease. Conversely, high-output states typically coincide with reduced peripheral vascular resistance. Thus, employing vasodilators to address congestion may exacerbate the issue. Consequently, effective treatment hinges on identifying the underlying pathophysiology and addressing the causative disease driving the high-output state.
Chronic severe anemia
In chronic severe anemia, numerous adaptations occur to sustain tissue oxygenation. Peripheral vasodilatation and elevated CO augment blood flow to the tissues [25]. SVR decreases, partly due to decreased blood viscosity and partly due to heightened basal activity of nitric oxide [76].
Obesity-related high output HF
In normotensive individuals with obesity, excessive accumulation of adipose tissue has been linked to an increase in central and overall blood volume. This, coupled with decreased SVR contributes to an elevation in CO. Heart rate tends to show minimal or no increase in obese subjects; thus, the predominant rise in CO stems from an increase in LV SV [77]. However, evidence suggests that not all normotensive obese individuals exhibit elevated CO. Neeland et al. [78] found that patients with peripheral obesity display higher CO and lower SVR, whereas those with central/visceral obesity exhibit lower CO and higher SVR. Obesity cardiomyopathy, defined as HF predominantly or entirely due to obesity [77, 79, 80], typically manifests in individuals with prolonged severe obesity. Although obesity cardiomyopathy is more prevalent in severely obese patients, the morphologic, hemodynamic, and ventricular function abnormalities contributing to this syndrome may predispose less severely obese individuals with other cardiac comorbidities like coronary artery disease or hypertensive heart disease to HF. Reddy et al. [21] conducted a retrospective analysis involving invasively confirmed cases of high-output HF, identifying morbid obesity as a significant cause of high-output HF in the modern era, a recognition that was previously lacking. Currently, there is no designated medication for obesity cardiomyopathy. Significant voluntary weight loss holds promise in reversing numerous hemodynamic, neurohormonal, and metabolic changes linked to obesity. This could lead to the reversal of cardiac remodeling and bolster ventricular function in obese individuals, along with improved functional capacity in those with HF.
Chronic arteriovenous fistula
When an arteriovenous fistula is present, SV rises owing to the decreased SVR. Despite the marked elevation in CO, diastolic arterial blood pressure stays low. Individuals with chronic arteriovenous fistula often contend with profound fluid retention. Remarkably, even momentary manual compression of a sizable fistula can raise blood pressure and induce diuresis and natriuresis [81].
Hepatic disease
Patients with severe hepatic disease and cirrhosis often exhibit abnormalities in the renal excretion of sodium and water. These abnormalities ultimately culminate in the development of ascites and hepato-renal syndrome, a condition associated with nearly 100% mortality [82]. The pathogenesis of renal sodium and water retention in cirrhosis is not attributed to an intrinsic kidney abnormality but rather to external mechanisms governing renal handling of sodium and water. Substantial evidence now indicates that heightened production of nitric oxide by endothelial cells significantly contributes to the development of peripheral arterial vasodilatation in severe hepatic diseases [83, 84]. Additionally, mesenteric, pulmonary, intrahepatic, and dermal arteriovenous shunts, coupled with deficiencies in the inactivation of circulating vasodilator substances, also play a role in decreasing SVR and the activation vasoconstrictive neurohormones in those patients [85, 86].
Other kind of high CO diseases
Various conditions characterized by high CO diseases, such as renal disease, cor pulmonale, acute mountain sickness, Paget’s disease of bone and polyostotic fibrous dysplasia, beriberi heart disease, and pregnancy, exhibit a correlation with diminished peripheral vascular resistance and arteriovenous shunting, thereby leading to elevated CO [87–90].
Prognosis: higher risk with HYPER-Phen
Previous reports have shown the adverse effect of the outcome of an increased, supra-normal resting LV function [8, 34, 36, 91, 92] (Table 3). In particular, Wehner et al. [9] described a nadir of risk for LVEF values of 60% to 65%, with a symmetrical increase in risk for EF values > 70% [hazard ratio (HR) 1.71] and < 40% (HR 1.73) in a sizable cohort exceeding 400,000 individuals with established or suspected coronary artery disease assessed through resting TTE. Similar findings were reported by Stewart et al. [92] in a population of close to half a million patients, with a nadir of risk corresponding to EF values of 65–69% (reference group). However, Kondo et al. [33] found no evidence of worse outcomes in patients with supranormal EF in a merged dataset of 336,999 participants. Force is simpler to measure than EF (ESV instead of EDV and ESV), load-independent as opposed to EF, and a pure index of LV contractility rather than a hybrid index of the form (EDV) and function (ESV) as EF. In head-to-head comparisons with EF, force proved to be more effective in distinguishing normal from diseased patients and in predicting outcomes [92].
Hypercontractile phenotype and prognosis
Parameter | Cut-off | Population | EF method | Finding | Reference |
---|---|---|---|---|---|
EF | ≥ 65% | 5,127 females with ACS | Qualitative | HR = 2.0 for 6-month death and 2.5 for in-hospital death | Saab et al. [91], 2010 |
≥ 70% | 403,977 allcomers | Qualitative | HR = 1.71 (HR = 1.17 for EF 65–70%) for all-cause death | Wehner et al. [9], 2020 | |
> 70% | 499,153 allcomers | Quantitative | Mortality rate slightly increase for EF > 75% | Stewart et al. [92], 2021 | |
> 70% | 33,699 HF patients | Quantitative | HR = 1.33 for non-cv death | Kondo et al. [33], 2023 | |
> 65% | 6,128 acute HF patients | Quantitative | HR = 2.65 for non-cv death | van Essen et al. [8], 2023 | |
> 60% | 255 acute HF patients | Qualitative | Event-free survival was worse | Ohte et al. [34], 2024 | |
> 70% | 16,994 allcomers | Quantitative | HR = 1.56 for all-cause death | Gotsman et al. [7], 2023 | |
GLS | > 26.7% | 502 with EF ≥ 55% | Not significant for cv death/cardiac hospitalization | Verdonschot et al. [36], 2021 |
EF: ejection fraction; ACS: acute coronary syndrome; HF: heart failure; HR: hazard ratio; GLS: global longitudinal strain
Patients with HYPER-Phen identified with increased force were more often women and off beta-blockers, consistent with the findings of van Essen et al. [8] among a cohort of individuals experiencing acute HF with supranormal LVEF. In addition, the level of N-terminal pro-brain natriuretic peptide (NT pro-BNP) was significantly elevated although not as high as other types of HF.
Conclusions
The supernormal LV contractile phenotype is a newly recognized state associated with multiple cardiovascular or systemic diseases and increased adverse outcomes. The HYPER-Phen can be described by various noninvasive parameters. The most commonly used traditional systolic parameter, LVEF, is influenced by both preload and afterload, which leads us to seek more reliable and simple metrics with less dependence on loading conditions, such as force as mentioned above. Hypercontractility occurs in some acute and chronic pathophysiological states, and treating the hypercontractile state hinges on identifying the underlying cause, which usually leads to improvement and even resolution of this unstable hemodynamics.
Abbreviations
CO: | cardiac output |
EDV: | end-diastolic volume |
EF: | ejection fraction |
ESV: | end-systolic volume |
GLS: | global longitudinal strain |
HCM: | hypertrophic cardiomyopathy |
HF: | heart failure |
HYPER-Phen: | hypercontractile phenotype |
LV: | left ventricular |
LVOTO: | left ventricular outflow tract obstruction |
MR: | mitral regurgitation |
SAM: | systolic anterior motion |
SV: | stroke volume |
SVR: | systemic vascular resistance |
TTE: | transthoracic echocardiography |
TTS: | Takotsubo syndrome |
Declarations
Author contributions
YW: Formal analysis, Writing—original draft. LY: Conceptualization, Supervision, Writing—review & editing.
Conflicts of interest
The authors declare that they have no conflicts of interest.
Ethical approval
Ethics Committee of Sichuan Academy of Medical Sciences & Sichuan Provincial People’s Hospital [protocol code: NO: 2023-341]. The study complies with the Declaration of Helsinki.
Consent to participate
Informed consent to participate in the study was obtained from all participants.
Consent to publication
Not applicable.
Availability of data and materials
Not applicable.
Funding
Not applicable.
Copyright
© The Author(s) 2024.