Abstract
Preterm birth, defined as delivery before 37 weeks of gestation, represents a global health concern linked to substantial cardiovascular risk later in life. Individuals born preterm, especially at earlier gestational ages, exhibit increased rates of hypertension, heart failure, and ischemic heart disease. The underlying mechanisms include disrupted fetal programming, impaired vascular remodeling, chronic neonatal inflammation, neuroendocrine immaturity, and epigenetic alterations. This review synthesizes current epidemiological evidence from large cohort studies and meta-analyses, integrating mechanistic insights from developmental biology. We discuss distinct prematurity categories—extremely preterm (< 28 weeks), very preterm (28–32 weeks), and moderate to late preterm (33–37 weeks)—highlighting their association with graded cardiovascular risk. Recent findings emphasize the role of non-transmitted parental genes and prenatal environmental toxic metal exposure as additional critical factors influencing fetal cardiovascular programming. A total of 57 articles, identified through a systematic search of PubMed, Embase, and Cochrane databases, were included to address these topics comprehensively. Early identification of preterm-born individuals as a high-risk cardiovascular group is essential for targeted screening, prevention, and interventions from childhood into adulthood. Future studies leveraging multi-omics and epigenetic approaches will further clarify these mechanisms, informing evidence-based guidelines to reduce cardiovascular morbidity associated with preterm birth.
Keywords
Preterm birth, cardiovascular risk, fetal programming, hypertension, heart failure, inflammation, epigenetics, vulnerable populationsIntroduction
Preterm birth (PTB) is a significant global health concern with far-reaching implications. It is generally categorized based on gestational age: “extremely” preterm refers to babies born before 28 weeks, “very” preterm applies to those born between 28 and 32 weeks, and “moderate to late” preterm includes births occurring between 33 weeks and 37 weeks [1, 2]. Gestational age is usually calculated starting from the first day of the last menstrual period, thus counting calendar days from conception and early fetal development [1].
The final trimester, particularly between 28 weeks and 37 weeks of gestation, is critical for cardiovascular system (CVS) development. During this period, there is significant maturation of cardiomyocytes and substantial vascular remodeling, processes essential for establishing a robust and functional heart. This phase allows for the refinement of myocardial architecture and the optimization of vascular networks, which collectively ensure adequate cardiac reserve and efficient hemodynamic performance. Disruption of these developmental processes by PTB can result in persistent alterations in cardiac structure and function, thereby predisposing individuals to cardiovascular diseases (CVDs) later in life [3–5].
While the survival of preterm infants has dramatically improved—due to neonatal intensive care advances, surfactant therapy, and better respiratory support—there is growing awareness that being born preterm can influence long-term health. Among the various adverse outcomes, cardiovascular risk has emerged as one of the most critical areas of concern. Multiple epidemiological studies, supported by mechanistic research, suggest that individuals born preterm face elevated risks of cardiometabolic disorders in adulthood, including hypertension, heart failure (HF), and ischemic heart disease (IHD) and other cardiometabolic conditions in adulthood [6–8].
The articles analyzed in this review were selected through a systematic search of the PubMed, Embase, and Cochrane databases, including original research articles, systematic reviews, and meta-analyses published in the past 20 years. Following an initial screening of 261 articles, 57 were ultimately included based on relevance criteria. This review aims to provide a comprehensive synthesis of current knowledge regarding the link between PTB and long-term cardiovascular risk, integrating epidemiological evidence, mechanistic research, and clinical perspectives. The ultimate goal is to emphasize the need for early identification and effective management of cardiovascular risk in individuals born preterm, in order to guide clinical strategies and public health policies aimed at reducing the burden of these complications in the general population.
PTB: definitions and growing global prevalence
Definitions and classifications
PTB occurs when delivery happens before 37 weeks of gestation [1, 2]. Within this broad category:
Extremely preterm (< 28 weeks): These infants require the most intensive neonatal support and have the highest morbidity and mortality [6, 8].
Very preterm (28–32 weeks): Infants face significant challenges, though often with better outcomes than extremely preterm newborns [7].
Moderate to late preterm (33–37 weeks): Although outcomes are comparatively better, moderate/late preterm infants are not exempt from long-term complications, including elevated cardiovascular risks [9].
Although gestational age remains the principal method of classifying PTB, literature underscores that other physiological or intrauterine factors may refine risk stratification [1, 10, 11]. For instance, an infant born at 35 weeks with significant fetal growth restriction (FGR) or compromised placental function could face outcomes similar to or even more severe than a 33-week infant with normal growth and adequate placental support. Moreover, the state of key organ systems—particularly pulmonary, cardiovascular, and neuroendocrine—can vary greatly within the same gestational age bracket, further influencing morbidity and long-term sequelae [12]. As a result, parameters such as fetal growth trajectories, maternal-fetal immune interactions, and uteroplacental circulation are increasingly recognized as critical adjuncts to the standard gestational-age classification for PTBs [13].
Global epidemiology of PTB
Recent estimates reveal that 14.84 million births were preterm in 2014 [14]. The highest rates are found in low- and middle-income countries (LMICs) such as those in Sub-Saharan Africa (~12.0%) and parts of Asia (~13.4%), whereas lower rates are reported in Europe (8.7%) and Oceania (10.0%). This discrepancy reflects socioeconomic, environmental, and healthcare-related factors that significantly influence the incidence and outcomes of PTB [14].
Moreover, the global trend appears to be rising, in part due to:
Impact on long-term health
PTBs carry immediate neonatal complications (respiratory distress syndrome, intraventricular hemorrhage, necrotizing enterocolitis) but also longer-term sequelae involving cardiometabolic outcomes [6, 8]. These likely result from incomplete organ development, disturbances in fetal programming, and postnatal neonatal intensive care unit (NICU) exposures (high-flow oxygen, mechanical ventilation, infections), as demonstrated by recent studies that have highlighted persistent cardiovascular alterations following such exposures [15, 16]. In the following paragraph, we will specifically explore how the degree of prematurity influences the onset of cardiovascular and renal diseases in adulthood.
PTB risks for cardiovascular and renal outcomes
Inverse correlation with gestational age
Numerous epidemiological studies emphasize the inverse relationship between gestational age and risk of HF, IHD, and chronic kidney disease (CKD) [6–8]. In extremely preterm infants, the risk of HF may be increased up to 17-fold, while very PTB confers ~3.6-fold higher risk [6]. Figure 1 (original timeline diagram) illustrates critical phases of cardiac development and how they may be disrupted by PTB.
Cardiovascular implications in late preterm
Late preterm (34–37 weeks), once thought relatively lower-risk, have also been associated with hypertension, HF, venous thromboembolism, and type 2 diabetes in adulthood [9]. Even moderate degrees of prematurity may incur long-term health impacts due to suboptimal intrauterine organ development and subsequent catch-up growth that predisposes to metabolic and vascular abnormalities.
Risk of CKD
In parallel with cardiovascular issues, CKD risk is higher in preterm-born individuals, particularly those with extremely PTB. Reduced nephron endowment—due to truncated renal organogenesis—compromises long-term renal function [8]. Maternal obesity, preeclampsia, and FGR may further exacerbate neonatal vulnerability.
Impact of maternal high blood pressure
Maternal BP and pregnancy outcomes
Elevated maternal blood pressure before or during early pregnancy correlates with adverse maternal outcomes [gestational hypertension, preeclampsia, gestational diabetes mellitus (GDM)] and is associated with higher rates of PTB, perinatal mortality, and NICU admissions [17].
Interaction with other maternal risk factors
Maternal body mass index (BMI)
Maternal body mass index (BMI) strongly influences PTB risk and neonatal outcomes. Kalk et al. [18] reported that higher maternal BMI correlates with an increased rate of preterm delivery and neonatal complications. Similarly, Chen et al. [19], focusing on women undergoing IVF/ICSI, found that elevated maternal BMI negatively affects pregnancy success and raises PTB rates. Obesity-related metabolic derangements (insulin resistance, chronic inflammation) may exacerbate fetal stress, increasing PTB vulnerability.
IVF and assisted reproduction
IVF/ICSI pregnancies exhibit a higher risk of PTB, likely due to a combination of maternal factors (e.g., advanced age, comorbidities) and iatrogenic interventions (e.g., multiple embryos). These pregnancies require specialized monitoring and potentially earlier interventions to reduce complications [20].
Vitamin D deficiency
Growing evidence implicates vitamin D deficiency in pregnancy as a risk factor for PTB and subsequent developmental programming issues. Inadequate maternal vitamin D status can influence placental function, fetal growth, and possibly predispose offspring to cardiometabolic disorders [21, 22].
In addition to elevated blood pressure, maternal smoking, metabolic syndromes, and specific conditions like GDM further exacerbate the probability of PTB [23]. For instance, a large French dataset indicated that GDM was associated with an increase in prematurity, respiratory distress, and congenital malformations [23]. The combination of maternal hypertension and GDM can create a compounding effect, potentially intensifying fetal stress and the risk of adverse neonatal outcomes.
Relevance to neonatal cardiovascular development
Maternal hemodynamic and metabolic conditions (hypertension, obesity, GDM, vitamin D deficiency) can affect the cardiovascular profile of offspring through placental changes, inflammatory mediators, and epigenetic modifications [24]. These prenatal exposures may intersect with postnatal NICU factors (mechanical ventilation, high-flow oxygen) to compound cardiovascular risks.
Global burden of CVD and PTB
CVD remains one of the leading causes of morbidity and mortality worldwide. As more preterm-born individuals survive into adolescence and adulthood, epidemiological data show a consistent pattern of increased cardiovascular risk [6, 8, 25]. These findings highlight significant public health and economic concerns, as healthcare systems must handle higher rates of hospital admissions, costly treatments, and long-term follow-up requirements.
Pathophysiological mechanisms
Fetal programming and developmental origins of health and disease (DOHaD)
The DOHaD hypothesis posits that adverse or suboptimal intrauterine environments can induce permanent developmental shifts in organ structure and regulatory systems [26]. Key mechanisms include:
Epigenetic modifications: DNA methylation, histone modifications, and microRNA (miRNA) expression changes profoundly affect cardiomyocyte proliferation, vascular reactivity, and metabolic pathways.
Intrauterine hypoxia or malnutrition: restrict organ growth, leading to reduced organ reserve.
Infections or inflammation: trigger immune dysregulation with lifelong consequences.
PTB amplifies these DOHaD pathways, as critical periods of organogenesis are truncated. Abrupt exposures to the NICU environment (e.g., oxygen fluctuations, mechanical ventilation, infection) can reinforce or further modify epigenetic “programming”, heightening long-term vulnerability [26, 27]. Recent advances indicate that parental genetic factors significantly influence fetal outcomes such as PTB and susceptibility to cardiovascular and metabolic diseases later in life. Notably, genetic variants carried by parents, including maternal and paternal alleles that encode adverse effects, may affect offspring outcomes even when these alleles are not directly transmitted to the subsequent generation. Such non-transmitted parental genetic effects imply mechanisms acting independently of traditional Mendelian inheritance, potentially mediated by the parental environment, epigenetic alterations, or intrauterine signaling pathways. These effects are also reported to vary depending on the sex of both parent and offspring, contributing further complexity to the inheritance patterns and developmental trajectories of diseases linked to PTB and metabolic-cardiovascular risk in adulthood [28–32].
Role of multi-omics
Recent multi-omics approaches (transcriptomics, proteomics, metabolomics) offer deeper insights into how PTB confers cardiometabolic risk. Li et al. [33] identified PCaaC38:6 via metabolomics as a potential biomarker related to PTB and later adverse outcomes. Such findings underscore the utility of advanced molecular profiling for early risk stratification and personalized interventions.
Role of miRNA
Recent data suggest that specific miRNAs significantly influence gene expression in the placenta and fetal tissues. In a study by Ramos et al. [34], distinct miRNA signatures were observed in small extracellular vesicles (sEV) among women undergoing preterm labor (PTL) and preterm premature rupture of membranes (PPROM). miR-612 and others implicated in endocytic and senescence pathways could modulate inflammatory, apoptotic, and metabolic processes. Although direct translation to neonatal cardiovascular outcomes requires further research, these findings highlight the mechanistic potential of miRNA-mediated fetal programming (Figure 2).
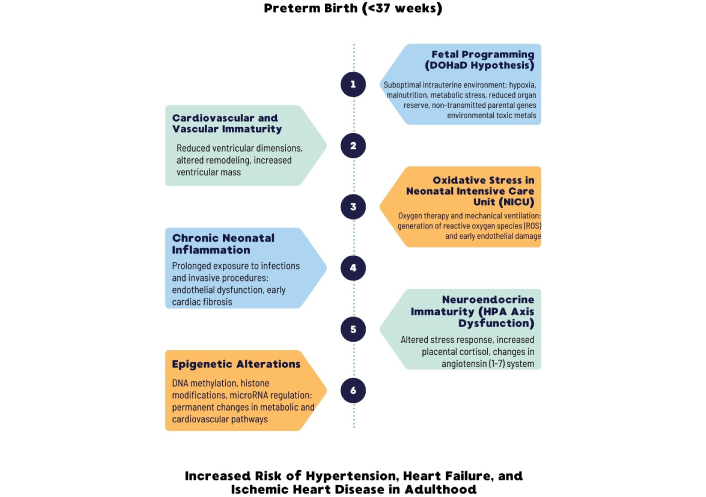
Schematic representation of the primary pathophysiological mechanisms linking preterm birth (PTB) to an increased risk of cardiovascular disease (CVD) in later life. Each mechanism interacts with the others, leading to structural and functional alterations that predispose preterm-born individuals to hypertension, heart failure, and ischemic heart disease
Vascular and cardiac alterations
Interruption of late gestational remodeling
Late gestational remodeling is essential for hyperplastic cardiomyocyte growth and optimal vascular development [4, 5]. When birth occurs prematurely:
Reduced ventricular dimensions: smaller left and right ventricles in preterm neonates [3].
Elevated LV mass and concentric geometry: Young adults born preterm frequently exhibit higher left ventricular mass, thicker walls, and shorter chamber lengths [4, 35].
Impaired cardiac strain: echocardiographic evaluations [including tissue doppler imaging (TDI)] reveal compromised systolic and diastolic strain [35].
TDI and echocardiographic evidence
A meta-analysis encompassing multiple developmental stages (neonates, children, adolescents, and young adults) confirmed that preterm-born individuals tend to have lower ejection fractions and worse diastolic function than term-born peers [36]. Early echocardiographic parameters like TDI velocities (S′, E′) and indices such as E/E′ highlight persistent myocardial dysfunction. Neonates born preterm (31–36 weeks) already exhibited significantly lower S′, E′, and EF values compared to term neonates, suggesting a continuum of risk that may manifest clinically as hypertension, left ventricular hypertrophy (LVH), or HF later in life [3, 36].
Anteroposterior abdominal aorta diameter (APAO)
Additional early biomarkers, such as the anteroposterior abdominal aorta diameter (APAO), could provide insight into vascular health. APAO in term newborns correlated with indices like length, head circumference, gestational age, and insulin resistance [homeostasis model assessment (HOMA) index] [37]. Although that study primarily involved term infants, it indicates that maternal factors (e.g., smoking, hyperglycemia, dyslipidemia) might influence neonatal vascular dimensions and possibly future atherosclerotic risk.
Role of inflammation and oxidative stress
Neonatal inflammatory state
PTB is often associated with a heightened inflammatory environment, both intra- and extrauterine. Immaturity of the immune system, frequent need for invasive procedures, and prolonged hospitalization in NICUs predispose these infants to infections. Cortese et al. [38] distinguishes early onset sepsis (EOS) from late onset sepsis (LOS), emphasizing that prematurity and low birth weight are major risk factors for these life-threatening infections.
Chronic or recurrent inflammatory insults may lead to endothelial dysfunction, reduced nitric oxide (NO) availability, and smooth muscle hypertrophy—key processes in vascular remodeling that elevate blood pressure [27]. Systemic inflammation can also directly impact myocardial tissue, increasing the likelihood of hypertrophic responses and myocardial fibrosis.
Oxidative stress in the NICU
High-flow oxygen therapy, often critical to support respiratory function in preterm infants, can generate reactive oxygen species (ROS). When antioxidant defenses are insufficient, oxidative stress ensues, compounding endothelial injury, as clearly reported in recent neonatal and clinical literature [39, 40]. The combined action of inflammation and oxidative stress can thus shape the vascular trajectory of a preterm newborn, predisposing to early arterial stiffness and higher blood pressure in later life.
Neuroendocrine and metabolic axis
Immaturity of the hypothalamic-pituitary-adrenal (HPA) axis
The hypothalamic-pituitary-adrenal (HPA) axis in preterm infants may be underdeveloped, limiting the neonate’s ability to respond to stress [4, 5]. This immaturity can disrupt cortisol regulation, affecting glucose metabolism, protein turnover, and lipid homeostasis, ultimately raising the risk of cardiometabolic disease. Moreover, stressful neonatal experiences—mechanical ventilation, repeated painful procedures—may further dysregulate the HPA axis.
Placental cortisol and angiotensin (1-7)
Aufdenblatten et al. [41] linked high placental cortisol in preeclampsia with PTB, while Chen et al. [42] found altered maternal/fetal angiotensin (1-7) levels correlated with PTB risk.
Catch-up growth and adolescent metabolism
Rapid postnatal growth—often observed when preterm infants “catch up”—may heighten insulin resistance and predispose to obesity or dyslipidemia. Overlapping inflammatory and oxidative mechanisms can further accelerate vascular aging, culminating in early manifestations of atherosclerosis or hypertension. These processes underscore the need for careful nutritional management and metabolic follow-up during childhood and adolescence (Figure 3).
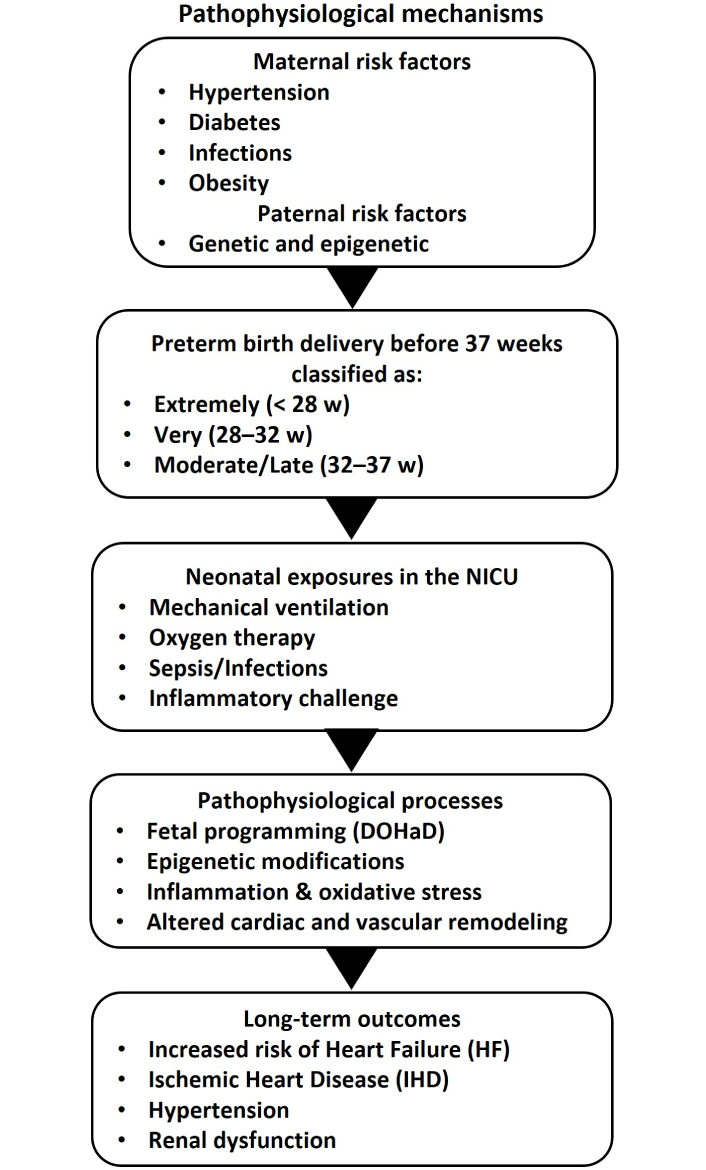
Flowchart summarizing pathophysiological mechanisms. W: week; NICU: neonatal intensive care unit; DOHaD: developmental origins of health and disease
Epidemiological evidence from Nordic cohorts
Large Nordic registries
Nordic countries maintain comprehensive national health registries, enabling large-scale, population-based research. These studies demonstrate that individuals born preterm (< 32 weeks) exhibit substantially increased risk of HF and IHD beginning in adolescence [6, 7].
Cosibling analyses
Comparing siblings where one is preterm and the other term—strengthen causal inferences by controlling for genetic and environmental confounders. These consistently reveal higher cardiovascular event rates in the preterm sibling, reinforcing a likely direct effect of PTB on cardiovascular risk [7].
Influence of gestational age strata
When stratifying by gestational age in these cohorts:
< 28 weeks (“extremely” preterm): The highest incidence of cardiovascular complications, with a notably earlier onset (late teens or early 20s).
28–32 weeks (“very” preterm): Elevated risk, though not as pronounced as extremely preterm.
33–37 weeks (“moderate/late” preterm): Modestly increased risk, often confounded by maternal conditions or FGR.
These gradients align with the physiologic logic of reduced organ development time, increased NICU interventions, and possibly more severe perinatal complications.
Insights from meta-analyses on hypertension and CVD
Meta-analytical findings
Meta-analyses of cohort and case-control studies corroborate an inverse gradient between gestational age and cardiovascular outcomes [43, 44]. Extremely preterm children show the highest predisposition to early-onset hypertension and subclinical cardiac dysfunction [36].
Methodological variability
Challenges in these meta-analyses include:
Heterogeneity: Definitions of prematurity (e.g., cutoff at 37 weeks vs. 36 weeks) and outcome measures vary.
Different eras: Neonatal care has evolved significantly over the decades, making older studies less comparable to contemporary data.
Follow-up duration: Many studies track individuals only up to early adulthood, possibly underestimating the full burden of adult-onset CVD.
Blood pressure assessments: Hospital or single-time measurements may differ from 24 h ambulatory assessments, affecting prevalence estimates of hypertension.
Nevertheless, the overarching conclusion is that prematurity is an independent risk factor for later CVD, and risk rises inversely with gestational age [7, 9, 44, 45].
Identification of vulnerable populations
Socioeconomic and ethnic disparities
PTB disproportionately affects low-resource settings, often correlating with socioeconomic disadvantage, limited prenatal care, and higher exposure to infectious or environmental stressors [14]. Genetic predispositions also shape PTB susceptibility, with recent genome-wide association studies [32, 46] identifying variants linked to preterm delivery.
Pre-existing maternal pathologies
Gestational diabetes mellitus (GDM): Billionnet et al. [23] demonstrated that infants born to mothers with GDM, especially insulin-treated cases, have increased perinatal risks, including preterm delivery and congenital heart defects.
Hypertension, obesity, and preeclampsia: These conditions can elevate the risk of prematurity and subsequently intensify the child’s susceptibility to hypertension or IHD [17, 43].
IVF/ICSI pregnancies: Exhibit higher PTB rates, necessitating specialized perinatal management [20].
Neonates with intrauterine growth restriction (IUGR)
IUGR babies are often considered a separate category from preterm, but the two can overlap. IUGR specifically denotes infants who fail to meet their growth potential in utero [47]. Similar to extremely preterm infants, IUGR neonates are prone to endothelial dysfunction, metabolic syndrome, and eventually CVD in adulthood. The interplay of IUGR and prematurity can further amplify these risks.
Intersection of disparities, maternal pathologies, and IUGR
Socioeconomic deficits, ethnic factors, and maternal complications create a complex environment where PTBs bring additional cardiometabolic vulnerabilities [14, 23]. Infants with both IUGR and prematurity, especially in the context of maternal hypertension or GDM, exhibit some of the highest likelihoods of adverse long-term outcomes, emphasizing the need for targeted interventions (Figure 4—conceptual Venn diagram). Timely identification of these high-risk subgroups enables the implementation of specific clinical pathways and personalized preventive strategies, significantly improving long-term cardiovascular outcomes.
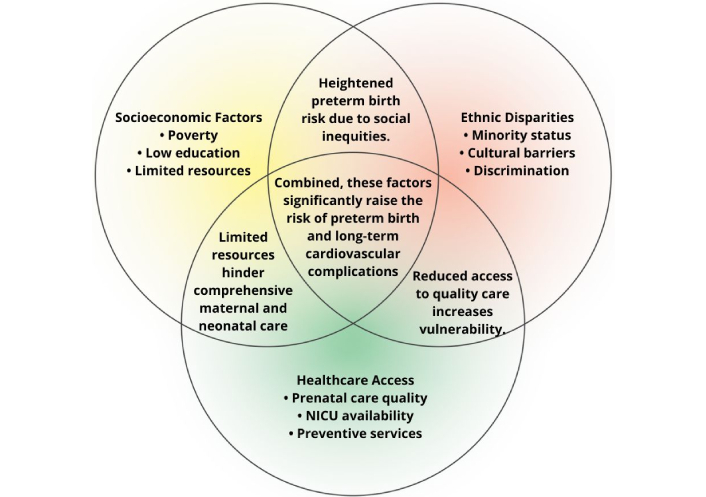
Venn diagram of socioeconomic, ethnic, and healthcare factors. NICU: neonatal intensive care unit
Environmental exposure to toxic metals
Prenatal exposure to lead, mercury, cadmium, arsenic can precipitate PTB and adversely affect postnatal cardiovascular outcomes, as highlighted in recent large-scale reviews and epidemiological studies [48–50]. Mechanisms include oxidative stress, DNA damage, and disruption of endocrine pathways, potentially compounding the baseline vulnerability of preterm infants.
Pediatric monitoring and prevention strategies
Guidelines for pediatric screening
Major organizations, including the American Academy of Pediatrics (AAP), recommend that children with a history of PTB undergo regular monitoring of:
Blood pressure.
Serum lipids.
Glycemic status [24].
Some authors advocate for echocardiographic evaluations or TDI in certain high-risk cases [3, 36]. The rationale is that early detection of hypertension or subclinical cardiovascular alterations (like LV hypertrophy, diastolic dysfunction) can guide timely interventions (Table 1).
Screening and follow-up recommendations
Age range | Recommended screening | Frequency | Notes/Reference values |
---|---|---|---|
Childhood (1–12 years) | Blood pressure and weight measurements | Every 6 months or annually | Follow pediatric guidelines for blood pressure levels |
Adolescence (13–18 years) | Echocardiography (including TDI) and metabolic evaluations | Annually or biannually | Monitor for early signs of ventricular dysfunction |
Young adulthood (19–30 years) | Comprehensive cardiovascular screening (BP, lipids, echocardiography) | Annually | Early intervention recommended if abnormalities are detected |
TDI: tissue doppler imaging
Nutritional counseling and lifestyle
Specific interventions
Vitamin D supplementation: Studies by von Websky et al. [22] and Reichetzeder et al. [21] suggest that correcting maternal and neonatal vitamin D deficiency may improve placental function and reduce long-term cardiometabolic sequelae.
Omega-3 fatty acid supplementation: Some RCTs have shown modest benefits for neonatal growth and neurodevelopment; potential cardiovascular benefits warrant further study.
Antihypertensive therapies: For adolescents or young adults born preterm with elevated BP, early initiation of ACE inhibitors or ARBs might reduce LV hypertrophy progression.
Structured exercise programs: Moderate-intensity exercise tailored to children’s age can foster healthy weight maintenance and cardiopulmonary fitness, offsetting catch-up growth-related metabolic risks [44].
Managing catch-up growth
“Catch-up” growth is often encouraged for preterm neonates; however, excessive catch-up can predispose to insulin resistance, obesity, and hypertension, as extensively documented in longitudinal studies on cohorts of preterm infants followed into adolescence [51, 52]. Close monitoring of weight gain trajectories, alongside balanced macronutrient intake, is essential to minimize adverse metabolic outcomes.
Early screening and telemedicine
Improvements in telemedicine offer promising avenues for consistent blood pressure measurements, metabolic checks, and consultations—especially for families in resource-limited settings. Telemonitoring can identify early deviations in blood pressure or growth curves, prompting referral to specialized care. As Andraweera et al. [24] and Azegami et al. [53] suggest, preterm-born adolescents already show higher blood pressure, underscoring the necessity of sustained monitoring approaches.
Managing neonatal inflammation and sepsis
Given the established role of inflammation in shaping long-term outcomes, minimizing infectious risk is crucial. Cortese et al. [38] delineates prophylactic antibiotic measures and stringent NICU infection-control protocols to reduce sepsis (EOS and LOS). Such efforts can limit chronic inflammation that might aggravate endothelial dysfunction. Additionally, controlling hyperoxia and providing careful ventilation strategies can help reduce oxidative stress.
Health policies and multidisciplinary coordination
Holistic neonatal follow-up
A coordinated, multidisciplinary approach—integrating neonatologists, pediatricians, cardiologists, nutritionists, social workers—ensures comprehensive management of PTB survivors [54, 55].
This synergy addresses the multifactorial nature of PTB, providing:
Structured screening protocols for BP, lipids, and glucose.
Echocardiographic surveillance in high-risk cases.
Nutritional support to manage catch-up growth effectively.
Psychosocial services to address socioeconomic barriers.
These interventions are not only beneficial clinically but also cost-effective by preventing severe outcomes like HF, stroke, or CKD in later life.
Resource allocation and socioeconomic interventions
Policymakers must prioritize:
Enhancing NICU infrastructure in high-burden regions.
Training local healthcare providers in recognizing and managing PTB-related risks.
Developing telemedicine networks to bridge geographic barriers.
Addressing environmental toxicants (heavy metals), especially in industrial or rural settings with high exposure rates.
Socioeconomic improvements—including maternal education, access to family planning, and improved prenatal care—can reduce PTB incidence and improve follow-up quality, as confirmed by evidence showing a clear decrease in the risk of PTB in more favorable socioeconomic contexts and with better healthcare interventions [14, 56, 57].
Managing maternal conditions
Initiatives focusing on early detection and management of conditions like chronic hypertension, preeclampsia, and gestational diabetes are equally critical. Early referral to specialized centers can curb the incidence of iatrogenic PTBs and lessen the impact of maternal cardiovascular risk factors on fetal development. Dublin et al. [43] highlighted how antihypertensive drug choices and maternal blood pressure control modulate PTB rates.
Research gaps
Multi-omics approaches
Although promising, multi-omics (epigenomics, transcriptomics, proteomics, metabolomics) remain underutilized in large-scale PTB cohorts. Future research should integrate these methods to:
Identify early biomarkers of CVD risk in neonates.
Elucidate how maternal factors (BMI, vitamin D status, toxic metals) are translated at the molecular level.
Develop personalized prevention and intervention strategies [34].
Clinical implications
Recognizing preterm infants as high-risk
Healthcare providers should be trained to view PTB as a lifelong cardiovascular risk marker, in line with recent recommendations emphasizing the clinical importance of early identification of this vulnerability [4, 6, 7]. This paradigm shift mandates specialized screening protocols from childhood through adulthood, ensuring timely detection of hypertension, LV hypertrophy, or incipient HF (Figure 5).
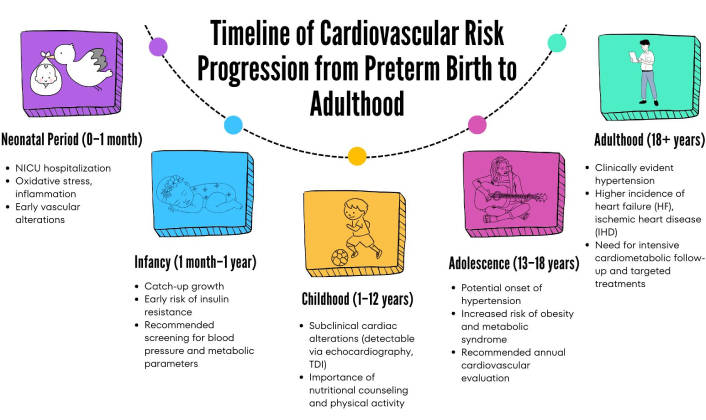
Timeline summarizing the progressive stages of cardiovascular risk associated with preterm birth. From initial neonatal alterations (oxidative stress, inflammation), the risk progresses throughout growth, highlighting the necessity of early screening and prevention efforts, ultimately leading to more severe clinical manifestations in adulthood such as hypertension, heart failure, and ischemic heart disease. NICU: neonatal intensive care unit; TDI: tissue doppler imaging
Integrating echocardiography and biomarkers
Advanced echocardiographic techniques (TDI, speckle-tracking) and biomarkers [e.g., N-terminal pro-B-type natriuretic peptide (NT-proBNP), inflammatory cytokines] can identify subclinical systolic or diastolic dysfunction, guiding early interventions. Metabolomic [33] and miRNA [37] signatures may further refine risk stratification.
Preventive approaches in NICUs
Improvements in neonatal care can mitigate long-term damage. Strategies include:
Minimizing hyperoxia and invasive ventilation to reduce oxidative stress.
Preventing and managing neonatal infections [38].
Optimizing nutrition: Sufficient but not excessive catch-up growth, mindful of micronutrient requirements.
Maternal-child health coordination
Given the interlinked nature of maternal conditions (e.g., hypertension, diabetes, obesity) and preterm outcomes, a continuum of care that starts before conception—extending through pregnancy and postpartum—could lower both PTB rates and subsequent cardiovascular sequelae in the offspring [43].
Final considerations
Summary of findings
This review highlights the significant association between PTB and increased risk of CVDs later in life. The major findings are:
Inverse gradient: The earlier the gestational age at birth, the higher the risk of HF, IHD, hypertension, and CKD [6–8].
Pathophysiological mechanisms: Fetal programming (DOHaD), incomplete cardiac and vascular remodeling, chronic inflammation, oxidative stress, and neuroendocrine immaturity all converge to raise cardiovascular vulnerability [26, 27, 35].
Vulnerable populations: Maternal obesity [18, 19], IVF pregnancies [20], LMICs with socioeconomic disadvantages, and toxic metal exposures [49, 50] face compounded risks [14, 23, 47].
Implications of neonatal care: NICU practices, including oxygen therapy and infection control, can modify these risks by reducing inflammation and stress on the developing CVS [38].
Echocardiographic and biomarker insights: Early changes in myocardial performance and molecular markers (e.g., PCaaC38:6, miRNAs) may forecast later cardiometabolic complications [3, 33, 37].
Importance of a structured follow-up
Given the consistent evidence linking PTB to long-term cardiovascular risk, health systems must adopt specialized, long-term monitoring for preterm-born individuals. This includes:
Regular BP and metabolic screening from infancy into adulthood.
Advanced echocardiography (TDI, speckle-tracking) for at-risk cohorts.
Lifestyle and nutritional interventions to mitigate catch-up growth issues.
Policy recommendations and future directions
Policy initiatives: Allocate resources to NICU expansions, staff training, and maternal health programs, with an emphasis on cost-effectiveness.
Multi-omics research: Large longitudinal cohorts should integrate multi-omics to refine risk prediction and personalize interventions.
Expanded follow-ups: Track cohorts into middle and older age for a complete picture of CVD incidence.
Interventional trials: Evaluate the efficacy of targeted therapies (e.g., antihypertensives, vitamin D supplementation, lifestyle interventions) initiated in childhood or adolescence.
Environmental regulations: Reduce exposure to heavy metals (lead, mercury, cadmium, arsenic) through stricter environmental policies and public health measures.
Call to action
Clinicians must routinely screen PTB survivors for early cardiometabolic abnormalities. Researchers should prioritize large-scale, multi-omics, and intervention studies targeting preterm populations.
Policymakers must invest in maternal-child health, mitigate environmental hazards, and ensure equitable healthcare access—especially in LMICs—thereby reducing the global burden of PTB-related CVD (Table 2).
Evidence-based strategies for reducing cardiovascular risk in preterm-born individuals
Strategy | Evidence level | Key considerations |
---|---|---|
Nutritional interventions | Moderate (RCTs, observational) | Vitamin D supplementation, balanced catch-up growth, omega-3 fatty acids |
Blood pressure screening | High (guideline-based) | Regular pediatric checks, possible 24 h ambulatory BP monitoring for high-risk groups |
Echocardiographic follow-up | Moderate-high (cohort studies) | TDI, speckle-tracking for early detection of LV dysfunction, especially in extremely preterm survivors |
Lifestyle & exercise programs | Moderate (some RCTs in pediatrics) | Emphasize moderate activity to prevent obesity and insulin resistance |
Antihypertensive therapy | Emerging evidence | Early ACE inhibitors or ARBs may halt progression of LV hypertrophy; requires further pediatric trials |
Telemedicine & mHealth | Growing (pilot programs, LMICs data) | Addresses follow-up gaps, especially in rural/low-resource settings |
Environmental exposure mitigation | Moderate (epidemiological data) | Reduction of maternal exposure to toxic metals (lead, mercury, arsenic) and pollutants |
Maternal health optimization | High (strong epidemiological links) | Control of maternal BMI, BP, glycemic status (preconception & prenatal) to lower PTB and subsequent risks |
This table highlights selected evidence-based interventions; ongoing research may refine these strategies further. LMICs: low- and middle-income countries; TDI: tissue doppler imaging; BMI: body mass index
Conclusions
PTB represents a lifelong determinant of cardiometabolic health. Disentangling the multifactorial mechanisms—ranging from fetal epigenetic programming to neonatal inflammation and oxidative stress—and integrating data from diverse cohorts worldwide underscores the urgent need to optimize maternal and neonatal care. From improved NICU protocols to comprehensive screening across the lifespan, concerted efforts by clinicians, researchers, and policymakers are vital to reduce the cardiovascular burden in the growing population of preterm survivors. Ultimately, addressing PTB is not merely a perinatal challenge, but a public health priority to ensure healthier outcomes well into adulthood.
Abbreviations
AAP: | American Academy of Pediatrics |
APAO: | Anteroposterior Abdominal Aorta Diameter |
BMI: | body mass index |
CKD: | chronic kidney disease |
CVDs: | cardiovascular diseases |
DOHaD: | developmental origins of health and disease |
EOS: | early onset sepsis |
FGR: | fetal growth restriction |
GDM: | gestational diabetes mellitus |
HF: | heart failure |
HOMA: | homeostasis model assessment |
HPA: | hypothalamic-pituitary-adrenal |
IHD: | ischemic heart disease |
IUGR: | intrauterine growth restriction |
LMICs: | low- and middle-income countries |
LVH: | left ventricular hypertrophy |
miRNA: | microRNA |
NICU: | neonatal intensive care unit |
NT-proBNP: | N-terminal pro-B-type natriuretic peptide |
PPROM: | preterm premature rupture of membranes |
PTB: | preterm birth |
PTL: | preterm labor |
ROS: | reactive oxygen species |
sEV: | small extracellular vesicles |
TDI: | tissue doppler imaging |
Declarations
Author contributions
MFC: Writing—original draft, Conceptualization, Validation, Supervision. LS: Formal analysis, Investigation, Methodology, Visualization, Writing—review & editing. FC: Formal analysis, Investigation. GDA: Formal analysis, Investigation. SP: Writing—original draft, Conceptualization, Validation, Supervision. All authors read and approved the submitted version.
Conflicts of interest
The authors declare that they have no conflicts of interest.
Ethical approval
Not applicable.
Consent to participate
Not applicable.
Consent to publication
Not applicable.
Availability of data and materials
Not applicable.
Funding
Not applicable.
Copyright
© The Author(s) 2025.
Publisher’s note
Open Exploration maintains a neutral stance on jurisdictional claims in published institutional affiliations and maps. All opinions expressed in this article are the personal views of the author(s) and do not represent the stance of the editorial team or the publisher.