Abstract
Aim:
Evaluate the role of myocardial work by echocardiography and determine its utility as an early diagnosis of cardiotoxicity.
Methods:
Single-center included 180 patients over 18 years old undergoing chemotherapy, the definition of cardiotoxicity for this study was to observe a left ventricular ejection fraction (LVEF) less than 50% and, or a global longitudinal strain (GLS) less than 16%. With these parameters, we divided the population into two groups, with cardiotoxicity and without cardiotoxicity. ROC curves were performed to determine the best cut-off point for global myocardial work to define cardiotoxicity. 2 × 2 tables were made to calculate sensitivity, specificity, positive predictive value, and negative predictive value.
Results:
Cardiotoxicity was established by obtaining cutoff points for global myocardial work index (GWI) with values lower than 1,381.5 mmHg%, Global Constructive Work (GCW) of 1,722 mmHg%, and myocardial efficiency [Global Work Efficiency (GWE)] of 88.5%, with a sensitivity (58.8%, 65.6%, and 52.9%) and specificity (91.8%, 82.1%, and 89.6%) respectively.
Conclusions:
We propose the measurement of myocardial work as a diagnostic tool for cardiotoxicity, as it has good specificity and negative predictive value, serving as an early diagnostic tool for cardiotoxicity without waiting for a decrease in LVEF and without being a marker influenced by loading conditions, in patients undergoing antineoplastic treatment.
Keywords
Global myocardial work index (GWI), cardiotoxicity, cancerIntroduction
Chemotherapy-induced cardiotoxicity is defined as the adverse side effects that chemotherapeutic agents have on cardiac function and anatomy [1]. There are several types of chemotherapy-induced cardiotoxicity, including acute cardiotoxicity, chronic cardiotoxicity, and late cardiotoxicity.
Acute cardiotoxicity occurs during or shortly after chemotherapy administration and is associated with the development of arrhythmias, hypotension, hypertension, prolonged QT interval, myocarditis, and heart failure [2]. On the other hand, chronic cardiotoxicity develops after prolonged exposure to chemotherapy and may cause irreversible structural damage to the heart (myocardial fibrosis) [3]. Late cardiotoxicity may appear months or years after chemotherapy or radiotherapy administration and may increase the risk of cardiovascular diseases such as ischemic heart disease and heart failure.
Therefore, a comprehensive clinical evaluation and establishing cardiovascular risk factors in chemotherapy or radiotherapy patients are crucial. This includes assessing the presence of pre-existing heart disease, current clinical status, and any symptoms of heart disease [4].
In general, chemotherapy-induced cardiotoxicity harms patients’ quality of life and survival. Early identification of chemotherapy-induced cardiotoxicity and appropriate intervention can help prevent or minimize cardiac damage and improve patients’ prognosis [5, 6].
Many definitions have been proposed to describe cardiovascular toxicity related to oncologic therapy, leading to errors in diagnosis and treatment. The need to harmonize these definitions has been acknowledged, resulting in recent international definitions of cardiovascular toxicity about cancer therapy [2].
Overall, it is important to consider the risks of cardiotoxicity when choosing a chemotherapy regimen for a patient. In some cases, preventive measures such as regular cardiac function monitoring and administering cardiac protectors like dexrazoxane in patients treated with anthracyclines can be used. Additionally, in some patients at high risk of cardiotoxicity, modifying the chemotherapy regimen or considering therapeutic alternatives may be necessary [7].
Chemotherapy regimens associated with cardiotoxicity
There are different types of chemotherapy and radiotherapy agents, and some of them are considered more cardiotoxic than others (Figure S1) [8].
Among them are anthracyclines, such as doxorubicin and epirubicin, which are used in the treatment of different types of cancer, including breast cancer, lymphoma, and leukemia [9]. These chemotherapeutic agents can cause both acute and chronic cardiotoxicity, including congestive heart failure and cardiac arrhythmias.
Another chemotherapy regimen associated with cardiotoxicity is tyrosine kinase inhibitors, such as Ibrutinib, which is available orally for the treatment of chronic lymphocytic leukemia, lymphoma, and Waldenstrom’s macroglobulinemia [10].
Monoclonal antibodies like trastuzumab, used in the treatment of HER2-positive breast cancer, also carry the risk of left ventriculfar dysfunction, which may be reversible or irreversible [11].
Imagine techniques and definitions of chemotherapy-induced cardiotoxicity
Transthoracic echocardiography is a non-invasive technique that can be performed at all stages of cancer to evaluate and detect coronary, myocardial, valvular, and pericardial disease, as well as complications secondary to therapy (chemotherapy/radiotherapy). Transthoracic echocardiography remains the first-line imaging modality in evaluation, diagnosis, and surveillance [12, 13].
Currently, validated methods for detecting cardiotoxicity include measurement of left ventricular ejection fraction (LVEF) and global longitudinal strain (GLS). Regarding LVEF, a reduction > 10% (10 absolute percentage points) from the baseline evaluation or an LVEF < 50% is considered significant, or a reduction > 15% with an LVEF > 50%. The European Society of Cardiovascular Imaging defines cardiotoxicity as a reduction of more than 10 absolute percentage points to a value less than 50% At the same time, degrees of cardiotoxicity have also been established (Table S1).
An LVEF between 50–54% is considered borderline and requires further investigation before labeling a patient with normal or abnormal systolic function [14–16].
There are different tools to evaluate cardiotoxicity which can help us with the limitations of performing only LVEF [17] (variability inter an intra-observer, geometric assumptions, lack of capability to show early systolic dysfunction), which includes advanced echocardiography techniques [three dimensional reconstruction (3D), two dimensional images (2D) Strain and Myocardial Work], Multigated Acquisition Scan (MUGA) and magnetic resonance imaging (MRI) are available. All these methods help assess the presence of cardiotoxicity (Figure 2).
If the possibility of evaluating LVEF by 3D reconstruction is available, it has better reproducibility than the conventional 2D method in patients undergoing cancer treatment [18]. It is recommended to use the same technique in patient follow-up.
The introduction of software in echocardiography machines capable of acquiring deformation of cardiac fibers (longitudinal) through speckle tracking (acoustic markers in the myocardium) can detect subclinical dysfunction.
A reduction > 15% from the baseline value establishes the diagnosis of cardiotoxicity, or a strain of –16% without prior evaluation. Its better reproducibility compared to 2D LVEF has been demonstrated [19–21].
The major limitation of GLS is that values are highly influenced by loading conditions. Patients receiving cancer treatment may experience alterations in blood pressure, vomiting, and diarrhea secondary to chemotherapy, hence the suggestion of using new parameters that can detect myocardial dysfunction early and do not have these limitations [22–24].
The non-invasive evaluation of strain curves and blood pressure correlates with myocardial oxygen consumption and metabolism. Global myocardial work index (GWI) combines strain deformation with hemodynamics (non-invasive left ventricular systolic pressure: systemic arterial pressure) [25]. Since 2012, the correlation of invasive and non-invasive pressure-strain curves has been demonstrated.
GWI represents the work performed by the myocardium from the closure of the mitral valve to the opening of the mitral valve, reflecting the metabolism and oxygen consumption of the left ventricle. Its advantage lies in the correction of afterload [26–29].
The parameters that have been established as normal for myocardial work are mentioned below in (Table S2).
This study aims to evaluate the role of myocardial work by echocardiography and determine its utility as an early diagnosis of cardiotoxicity.
Materials and methods
We conducted a cross-sectional diagnostic test study at a single center. Between January 2023 and March 2024, 200 cancer patients underwent echocardiography in our echocardiography department. A total of 180 patients were included in the analysis after meeting the selection criteria: aged 18 years or older, with a confirmed cancer diagnosis, having received at least one cycle of chemotherapy, and having agreed to participate in the study by signing informed consent. Patients with a history or echocardiographic evidence of ischemic or valvular heart disease, as well as those with a poor echocardiographic window, were excluded.
Transthoracic echocardiogram
Prior to the study, the patient’s blood pressure was measured following international standards for proper blood pressure recording. An E95 device from General Electric with a sectorial 5S transducer was used for echocardiographic measurements, adhering to the practice guidelines of the American Society of Echocardiography.
For performing myocardial work 2D images of apical 4-chamber, 3-chamber, and 2-chamber views were obtained with a depth of around 15 cm to frame the left ventricle, observe the mitral annulus, and approximately one centimeter of the left atrium. These images were acquired with a frame rate of at least 85% of the patient’s heart rate at the time of the study and/or between 40 frame rates and 90 frame rates per second. Aortic valve opening and closing were obtained by marking the reference points on pulsed Doppler of the left ventricular outflow tract. Mitral valve opening and closing were obtained by marking with pulsed Doppler in an apical 4-chamber view at the tips of the mitral valve leaflets, marking the opening at the onset of the Doppler flow of the E-wave and the closing at the end of the Doppler flow of the A-wave. After obtaining the images, myocardial longitudinal deformation was processed using automated functional imaging (AFI) software on the equipment. Each view was labeled, and the region of interest (ROI) was adjusted to involve the entire myocardium, 1 mm above the mitral annulus, and not involve the epicardium. This provided the values of GLS. Subsequently, systolic blood pressure was input to analyze, the myocardial work index, and its derivatives.
Through advanced deformation analysis via transthoracic echocardiography, the following parameters are obtained:
Global myocardial work: This is the amount of energy the myocardium needs to contract and relax (mmHg%).
Global constructive myocardial work: This is the positive work done by the segments of the left ventricle contributing to ejection. Shortening during systole and lengthening during isovolumetric relaxation (mmHg%).
Global wasted myocardial work: This is the negative work done by segments of the left ventricle that do not contribute to ejection (mmHg%).
Global myocardial efficiency: This is the percentage of myocardial work. It represents the ratio between global work and the sum of constructive and wasted work (%).
Definition of cardiotoxicity
For this work, we defined cardiotoxicity as follows: a GLS value < 16% (absolute values) and or LVEF < 50%.
Statistical analysis
Qualitative variables were described in percentages and proportions, while the distribution of quantitative variables was explored using histograms and Kolmogorov-Smirnov test. Those with a normal distribution were reported as means with their respective standard deviations, and those not following a normal distribution were reported as medians with interquartile ranges.
For assessing statistical differences between categorical variables, we performed the χ2 test, and for quantitative variables, we made student T test.
Inferential statistics were performed using ROC curves to determine the cutoff point of myocardial work that best identifies the presence of cardiotoxicity. Additionally, other parameters derived from the analysis of myocardial work index were evaluated, such as constructive work, wasted work, and myocardial efficiency. For each value in the ROC curves, 2 × 2 tables were generated to calculate sensitivity, specificity, as well as positive and negative predictive values for identifying the presence of cardiotoxicity. Data analysis was performed using SPSS version 29 software.
Results
One hundred eighty patients were analyzed. We divided the population into two groups: those with echocardiographic data suggesting cardiotoxicity and those without. The Table 1 shows the differences on baseline characteristics between the groups.
Differences in baseline characteristics between patients with cardiotoxicity and those who don’t have cardiotoxicity
Variables | Patients with cardiotoxicityN = 34 | Patients without cardiotoxicityN = 146 | P |
---|---|---|---|
Age (years) | 55 | 54.4 | 0.442 |
Gender | |||
Male | 11.8% | 14.4% | 0.468 |
Female | 88.2% | 85.6% | |
Systemic arterial hypertension | 29.4% | 27.4% | 0.483 |
Diabetes | 14.7% | 19% | 0.490 |
Dyslipidemia | 8.8% | 9.6% | 0.595 |
Smoking | 5.9% | 15.1% | 0.124 |
Systolic blood pressure (mmHg) | 127.7 | 123.1 | 0.121 |
Diastolic blood pressure (mmHg) | 74.7 | 72.8 | 0.141 |
BMI (kg/m2) | 27.8 | 26 | 0.078 |
Cancer type | |||
Lymphoma | 6.1% | 6.2% | 0.547 |
Breast cancer | 74.5% | 72.6% | |
Leukemia | 2.7% | 3.4% | |
Others | 16.6% | 17.8% | |
Treatment | |||
Anthracyclines | 75% | 55.9% | 0.034 |
HER2 | 68.8% | 57.9% | 0.176 |
Fluoropyrimidines | 9.4% | 9.0% | 0.582 |
Multi-targeted kinase inhibitors BCR-ABL | 0% | 4.1% | 0.297 |
Androgen deprivation | 0% | 5.5% | 0.196 |
Endocrine therapy for breast cancer | 6% | 35% | 0.345 |
Epidermal growth factor receptor inhibitors | 0% | 0.7% | 0.824 |
Radiation therapy | 15.2% | 23.3% | 0.219 |
Others | 78.1% | 70.3% | 0.256 |
Combination chemotherapy and radiation therapy | 46.9% | 48.6% | 0.707 |
BMI: body mass index
The number of chemotherapy sessions was analyzed for the two therapeutic groups that involved the highest number of patients, which were anthracyclines and HER2-directed therapies. For anthracyclines, in the group that did develop cardiotoxicity, the range of cycles at the time of diagnosis was 1–6 vs. 1–10, P = 0.086, while for HER2-directed therapy, the range of sessions in the group that did develop cardiotoxicity were 1–22 vs. 1–40, P = 0.447.
Table 2 summarizes the echocardiographic characteristics according to the presence or absence of cardiotoxicity.
Echocardiographic differences between the group with cardiotoxicity and the group without cardiotoxicity
Variables | Patients with cardiotoxicity | Patients without cardiotoxicity | P |
---|---|---|---|
Diastolic diameter (mm) | 43.3 ± 5.7 | 42.6 ± 5 | 0.254 |
Systolic diameter (mm) | 27.6 ± 6.3 | 27.0 ± 5.1 | 0.383 |
Septum (mm) | 8.2 ± 2.1 | 8.4 ± 1.4 | 0.362 |
Posterior wall (mm) | 8 ± 1.4 | 8.2 ± 1.4 | 0.275 |
Left ventricular mass (g/m2) | 71.1 ± 15.7 | 66 ± 16.7 | 0.127 |
RWT | 0.38 ± 0.11 | 0.38 ± 0.08 | 0.418 |
LVEF (%) | 58.6 ± 10.6 | 63.7 ± 6 | 0.005 |
GLS (%) | 13.9 ± 1.9 | 19.4 ± 4.2 | < 0.001 |
Postsystolic contractions | 74.5% | 16.5% | < 0.001 |
GWI (mmHg%) | 1,421.4 ± 396.7 | 1,925.4 ± 448 | < 0.001 |
GCW (mmHg%) | 1,669.6 ± 399 | 2,115.1 ± 484 | < 0.001 |
GWW (mmHg%) | 179.8 ± 106.7 | 135.3 ± 106 | 0.015 |
GWE (%) | 88.2 ± 5 | 104.6 ± 138 | 0.246 |
Mechanical dispersion (ms) | 76.7 ± 35 | 47.3 ± 15.9 | < 0.001 |
RWT: relative wall thickness; LVEF: left ventricular ejection fraction; GLS: global longitudinal strain; GWI: global myocardial work index; GCW: Global Constructive Work; GWW: Global Wasted Work; GWE: Global Work Efficiency
ROC curves were generated to determine the optimal cutoff points for the various myocardial work indices to differentiate between the presence and absence of cardiotoxicity (Figure 1).
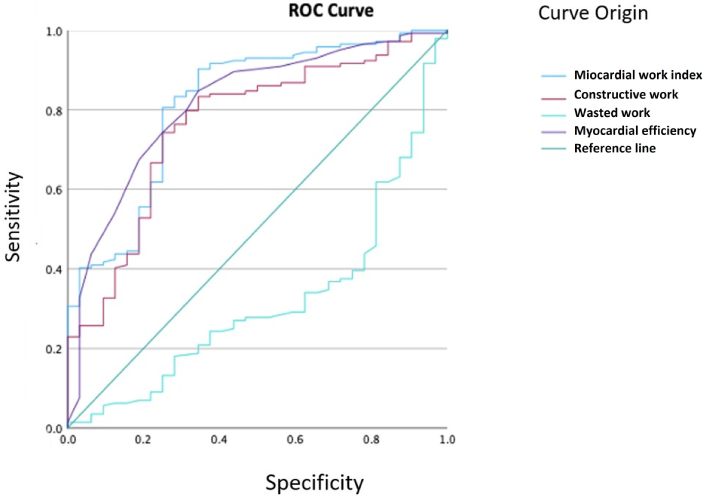
ROC curve shows the performance of each myocardial work index to differentiate cardiotoxicity
The values that best determined the presence of cardiotoxicity were GWI with a cut-off point of 1,381.5 mmHg%, area under the curve of 0.81 (CI 0.73–0.89), and for Global Work Efficiency (GWE) with a cut-off point of 88.5%, area under the cross of 0.81 (CI 0.73–0.89). For the overall Global Constructive Work (GCW), the cut-off point was 1,722 mmHg% with an area under the cross of 0.76 (CI 0.73–0.89). The Global Wasted Work (GWW) did not have values that could discriminate between the presence or absence of cardiotoxicity.
With the cutoff points described, the population was divided by each of them to create contingency tables and determine the sensitivity, specificity, positive predictive values, and negative predictive values of the GWI, GCW, and GWE (Tables 3, 4 and 5).
Contingency table for global work index
Diagnostic performance | Percentages | 95% CI | |
---|---|---|---|
Lower limit | Upper limit | ||
Disease prevalence | 18.89% | 13.60% | 25.54% |
Diagnostic accuracy | 85.56% | 79.37% | 90.18% |
Sensitivity | 58.82% | 40.83% | 74.87% |
Specificity | 91.78% | 85.77% | 95.49% |
Positive predictive value | 62.50% | 43.75% | 78.34% |
Negative predictive value | 90.54% | 84.35% | 94.54% |
CI: confidence interval
Contingency table for global constructive work
Diagnostic performance | Percentages | 95% CI | |
---|---|---|---|
Lower limit | Upper limit | ||
Disease prevalence | 17.98% | 12.79% | 24.58% |
Diagnostic accuracy | 79.21% | 72.36% | 84.77% |
Sensitivity | 65.63% | 46.77% | 80.83% |
Specificity | 82.19% | 74.81% | 87.84% |
Positive predictive value | 44.68% | 30.46% | 59.76% |
Negative predictive value | 91.60% | 85.13% | 95.53% |
CI: confidence interval
Contingency table for global myocardial efficiency
Diagnostic performance | Percentages | 95% CI | |
---|---|---|---|
Lower limit | Upper limit | ||
Disease prevalence | 19.10% | 13.76% | 25.81% |
Diagnostic accuracy | 82.58% | 76.03% | 87.69% |
Sensitivity | 52.94% | 35.40% | 69.84% |
Specificity | 89.58% | 83.12% | 93.85% |
Positive predictive value | 54.55% | 36.60% | 71.47% |
Negative predictive value | 88.97% | 82.43% | 93.36% |
CI: confidence interval
Discussion
Due to the increasing number of cancer patients receiving chemotherapy and/or radiotherapy, the use of non-invasive diagnostic tests such as echocardiography becomes relevant in determining the presence of cardiotoxicity. Advanced echocardiography techniques as myocardial work allow for a more detailed analysis of cardiac function and myocardial fiber deformation.
Our work shows several important findings. Firstly, the parameters of myocardial work decreased in patients identified with cardiotoxicity by validated methods (LVEF and GLS). However, these methods have a couple of disadvantages. LVEF is suggested not to be used in early (subclinical) stages of cardiotoxicity as the sole marker because, at these stages, a normal ejection fraction does not exclude myocardial dysfunction. A significant reduction in LVEF reflects manifest cardiac damage. As for myocardial deformation by two-dimensional speckle tracking, its value is influenced by loading conditions, so if there are changes in blood pressure between medical visits, it can change the value of this method and confuse the diagnosis of treatment-related cardiac damage. Hence, the use of new parameters that can detect myocardial dysfunction early and do not have these limitations is suggested.
In different pathologies such as ischemic heart disease, systemic arterial hypertension, heart failure, aortic stenosis, etc., the importance of using advanced myocardial deformation by echocardiography to establish early subclinical myocardial damage in order to initiate medical management or invasive treatment.
In our study, the cutoff points for GWI to demonstrate cardiotoxicity were values lower than 1,381.5 mmHg%, GCW values lower than 1,722 mmHg%, and for GWE values of 88.5%. The best sensitivity and specificity were for GWI and GWE. Comparing these cutoff points in relation to those established in the EACVI NORRE (European Association of Cardiovascular Imaging Normal Reference Ranges for Echocardiography) study, they were lower (or close to the lower limit) for normality [29].
It is worth emphasizing that GWI had a specificity greater than 90%, which identifies the absence of cardiotoxicity in oncology patients, allowing continuation of management for their underlying disease without the limitations mentioned with the already validated methods. As for wasted work, no significant results were found for this marker.
The performance of advanced ventricular mechanics obtaining GWI, GCW, GWW and GWE are based on myocardial deformation by two-dimensional speckle tracking, adding the patient’s measured blood pressure at the time of the study to its calculation. Therefore, it promises to be a method that helps us assess patients in subclinical stages without the limitation of changes in loading conditions between medical visits, which constitutes a valuable tool for early prediction of cardiotoxicity (Figure 2).
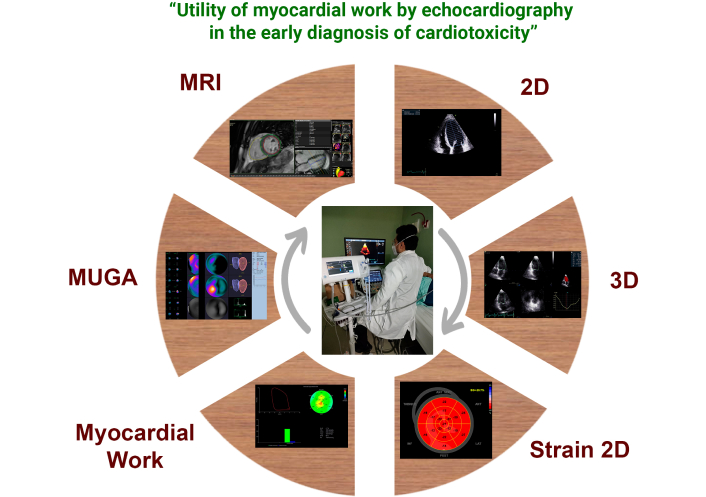
Myocardial work as a current diagnostic tool for evaluating cardiotoxicity. Myocardial work forming part of current diagnostic methods for evaluating cardiotoxicity currently include echocardiography in its 2D, 3D, 2D strain, and myocardial work modalities (for which blood pressure measurement at the time of the study is required). MUGA and MRI are available. All these methods help assess ventricular systolic function and thus the presence of cardiotoxicity. MUGA: Multigated Acquisition Scan (nuclear ventriculography); MRI: magnetic resonance imaging; 2D: two dimensional images; 3D: three dimensional reconstruction
Regarding the type of oncologic treatment, the groups most related to cardiotoxicity were, firstly, anthracyclines and subsequently HER2-directed therapies.
With respect to the classical cardiovascular risk factors, which have been previously associated with the risk of cardiotoxicity in multiple studies, no significant difference was found between patients with or without cardiotoxicity. Furthermore, no statistical significance was found in any of the other evaluated echocardiographic parameters.
Limitations
Myocardial work indexes are only available on General Electric echo machines, which not all healthcare services have access to. Other limitation in our study is that we used as a golden standard for the diagnostic test echocardiograms parameters that can be less accurate than myocardial work, instead of ventricular ejection fraction through magnetic resonance. That’s because we don’t have the MRI available for every patient.
Conclusions
- GWI having good specificity and negative predictive value, serves as a tool for early diagnosis of cardiotoxicity in individuals receiving chemotherapy.
- Similarly, GCW and GWE are parameters that support the GWI as confirmatory tests for cardiotoxicity due to their good specificity, diagnostic accuracy, and negative predictive value.
- We propose the measurement of myocardial work as an echocardiographic tool for the early diagnosis of cardiotoxicity (without waiting for a decrease in LVEF) and without being a marker that may be influenced by loading conditions, in patients undergoing antineoplastic treatment.
Abbreviations
GCW: | Global Constructive Work |
GLS: | global longitudinal strain |
GWE: | Global Work Efficiency |
GWI: | global myocardial work index |
GWW: | Global Wasted Work |
LVEF: | left ventricular ejection fraction |
MRI: | magnetic resonance imaging |
MUGA: | Multigated Acquisition Scan |
Supplementary materials
The supplementary material for this article is available at: https://www.explorationpub.com/uploads/Article/file/101254_sup_1.pdf.
Declarations
Acknowledgments
We would like to express our gratitude to the entire echocardiography service at the Cabinet Department of the National Medical Center 21st century as they were instrumental in-patient recruitment during this time. We also want to thank Dr. Daniel Calderón Rodríguez from the Nuclear Medicine service at the Cardiology Hospital, National Medical Center 21st century, and Dr. Rubén Fernández Galera from Vall d’Hebron Hospital for their contribution with nuclear medicine and cardiac magnetic resonance images.
Author contributions
DCP: Investigation, Writing—original draft. DRZ: Formal analysis, Methodology. GRG: Validation, Investigation. ICG: Investigation, Validation. CMH: Conceptualization, Project administration.
Conflicts of interest
The authors declare that they have no conflicts of interest.
Ethical approval
The study was approved by the local health research committee 3604, Cardiology Hospital National Medical Center 21st century, Registration number R-2023-3604-056.
Consent to participate
Informed consent to participate in the study was obtained from all participants.
Consent to publication
Not applicable.
Availability of data and materials
We have the database of this study electronically, if you need this or revision of any data, we will gladly send it to you, contact dnicrck@hotmali.com.
Funding
Not applicable.
Copyright
© The Author(s) 2025.
Publisher’s note
Open Exploration maintains a neutral stance on jurisdictional claims in published institutional affiliations and maps. All opinions expressed in this article are the personal views of the author(s) and do not represent the stance of the editorial team or the publisher.