Abstract
A high consumption of ultra-processed food (UPF) is a hallmark of Western diets that has been related to increased risk of non-communicable diseases. As an underlying mechanism, UPF may promote non-alcoholic fatty liver disease (NAFLD) which is a key driver of metabolic impairment with extra-hepatic manifestations like type 2 diabetes, cardiovascular disease, chronic kidney disease, and osteoporosis among others. The present review provides an overview of UPF properties that may promote NAFLD and are thus potential targets for reformulation of UPF. Such approaches should address improvements in the quality of carbohydrates and fat, changes in food texture that lower eating rate as well as ingredients that prevent excess caloric intake or avoid dysbiosis and leaky gut syndrome. Promising strategies are enrichment with fiber, prebiotics, phytochemicals, and protein with a concurrent reduction in glycemic load, energy density, saturated fatty acids (FA; SFA), emulsifiers, fructose, and non-caloric sweeteners. Future studies are needed to examine the interactive and protective effects of such modifications in the composition of UPF on prevention and treatment of NAFLD.
Keywords
Ultra-processed foods, non-alcoholic fatty liver disease, appetite control, energy balance, saturated fatty acids, glycemic loadIntroduction
The development of non-alcoholic fatty liver disease (NAFLD) is closely linked to lifestyle factors that promote a positive energy balance. The increasing adoption of ultra-processed food (UPF) according to the NOVA-classification [1] is coincidental to the rising global prevalence rate of NAFLD, e.g., in Asia over the past 15 years (from +25.3% between 1999–2005 to +33.9% between 2012–2017) [2]. Whereas increasing evidence shows that the consumption of UPF promotes excess energy intake and may thus contribute to the development of NAFLD, there are also other proposed mechanisms that may explain the simultaneous increase in NAFLD and the consumption of UPF. According to the NOVA-classification, food products can be classified depending on processing as (1) unprocessed or minimally processed foods, (2) processed culinary ingredients, (3) processed foods, and (4) UPF [1]. Potential mechanisms that link the consumption of UPF to the development of NAFLD are typical characteristics of “Western” type foods like hyper-palatability, soft texture, high energy density, high saturated fat, and high sugar content. The present review addresses these mechanisms as potential targets for reformulation of UPF mainly by analyzing observational studies but also considering evidence from human interventions as well as animal studies.
Consumption of UPF may lead to NAFLD by promoting overfeeding
NAFLD is a common comorbidity of obesity and the metabolic syndrome [3, 4]. Compared to persons with normal weight, individuals with obesity are at 3.5-fold higher risk for NAFLD [3]. Excess hepatic triglyceride accumulation originates from adipose tissue lipolysis (~60%), de novo lipogenesis in hepatocytes (~25%) and from diet (~15%) [5]. Since the majority of adipose tissue-derived free fatty acids (FA; FFA) passing through the liver originates from lipolysis of abdominal subcutaneous adipose tissue [6], central obesity with impaired lipid storage capacity rather than the total amount of fat mass is a major risk factor for accumulation of liver fat.
An increasing number of cross-sectional and prospective cohort trials have shown a positive association between UPF consumption according to NOVA classification and the risk for weight gain and obesity [7–12], for a meta-analysis see [13]. In prospective studies, a higher UPF consumption was associated with a greater accumulation of abdominal obesity or visceral fat [14, 15]. In a cross-sectional study in adults with or without NAFLD, a positive association between UPF consumption and metabolic syndrome has been observed [16]. Although no association between a higher UPF consumption and NAFLD was found, subjects with a higher UPF consumption had a higher total energy intake, a higher relative intake of carbohydrates and a lower relative protein intake. However, among subjects with NAFLD, a higher UPF consumption was associated with higher odds for presumed non-alcoholic steatohepatitis (NASH) [16]. In an analysis of the PREDIMED-Plus trial (5,867 subjects, one-year follow-up), a higher UPF consumption was associated with higher levels of the non-invasive fatty liver index and the hepatic steatosis index [17]. Similarly, in a prospective cohort of Chinese adults (16,168 subjects, 4.2 years follow-up) increasing UPF consumption by one standard deviation (62.7 g/1,000 kcal per day) increased the risk for NAFLD by 6%, even when adjusted for traditional risk factors as age, sex, body mass index (BMI), smoking, etc. [18].
Causality between UPF consumption and weight gain can, however, only be derived from intervention studies. In a highly regarded randomized controlled trial by Hall et al. [19] in 20 inpatient adults (10 women and 10 men, 31.2 years ± 1.6 years, weight-stable with BMI 27 kg/m2 ± 1.5 kg/m2, as mean ± SE), ad libitum energy intake was increased by ~500 kcal/day when UPF were provided compared to a largely unprocessed diet matched for energy content, macronutrients and fiber. During 14 days of each intervention, participants gained 0.9 kg body weight consuming the ultra-processed diet whereas they lost 0.9 kg body weight consuming the unprocessed diet. These findings suggest, that there is indeed a causal relationship between regular consumption of a diet high in UPF and an increased risk for weight gain and obesity.
High energy density of UPF may lead to overfeeding
There are several mechanisms by which UPF consumption can trigger excess calorie intake. First of all, UPF usually have a higher energy density (i.e., energy content divided by weight of food served; kcal/g) compared to low processed foods (LPF). More energy dense diets tend to be associated with a higher daily energy intake and weight gain [20]. In an elegantly conducted intervention trial with normal-weight women, food consumption during a meal was more consistent in terms of weight of food compared to energy content of the consumed food when palatability and fat content were kept constant [21]. This insensitivity to food energy density was not only observed in women but also in men and in adults of different weight status as well as in 3- to 5-year-old children [22]. This insensitivity facilitates “passive overconsumption” [23] when an energy dense diet is consumed [21] and may partly explain the excess caloric intake with a diet high in UPF. This effect has been confirmed by the study by Hall and colleagues [19] because the UPF diet had a higher non-beverage energy density compared to the unprocessed diet (1.95 kcal/g vs. 1.05 kcal/g). In line with this finding, a recent meta-analysis has shown that lowering the energy density of the diet (with or without macronutrient manipulation) was associated with a large decrease of daily energy intake. This effect was dose-dependent and only minimal compensation at the following meals was observed [24]. A recently published secondary analysis using controlled intervention data from Hall et al. [19] as well as free-living data from the UK National Diet and Nutrition Survey, however, suggests that passive overconsumption only occurs to some degree and that overcompensation for higher energy density of foods (e.g., by reducing portion size) can be seen [25].
High eating rate of UPF consumption may lead to overfeeding
Eating more slowly may prevent overconsumption as it allows to register satiation before too much food is consumed [26, 27]. It has been shown that eating rates and thus also energy intake rates [i.e., eating rate (g/min) multiplied by the energy density (kcal/g) of the specific food; kcal/min] differ according to food processing defined by NOVA classification. Thus, UPF consumption leads to higher average energy intake rates (69 kcal/min ± 3 kcal/min) compared to processed (54 kcal/min ± 4 kcal/min) and unprocessed foods (36 kcal/min ± 4 kcal/min) [28]. This would provide an additional mechanism by which UPF may lead to overconsumption and weight gain. In line with this supposition, in the randomized controlled intervention by Hall and colleagues [19], the energy intake rate was increased by > 50% with UPF compared to the unprocessed diet. Energy intake rates showed, however, a wide range within each NOVA group demonstrating that the degree of processing is not the exclusive criterium for inducing a higher energy intake rate [28].
Food texture and matrix of UPF may lead to overfeeding
Food texture and matrix are known to also play a role in eating rate because softer/thinner foods can be consumed more quickly compared to harder/more chewy foods [29]. The satiating value of foods (per kcal) that are eaten quickly is lower compared to foods usually ingested slower [30] leading to consumption of higher quantities of foods that can be ingested more rapidly [31]. In other words, harder foods (e.g., raw vegetables) reduce ad libitum energy intake compared to soft foods (e.g., boiled vegetables) [32]. The impact of food texture on satiety was also investigated in a recent meta-analysis confirming an enhanced effect of solid and higher viscous foods on reducing hunger and increasing fullness ratings compared to liquid and low viscous foods [33]. It can therefore be assumed that a softer food texture per se may accelerate eating rate with minor impact on satiation. The effect of food texture in combination with food processing level on eating rate was investigated in a cross-over intervention [34]. Participants with normal-weight consumed ad libitum lunch meals at four occasions consisting of “soft minimally processed”, “hard minimally processed”, “soft ultra-processed”, and “hard ultra-processed” components. The results revealed an effect of food texture on eating rate, as hard foods were consumed slower evoking a 26% reduction in energy intake while foods with a soft texture were consumed faster. On the other hand, also the food processing level had an impact on energy intake as the lowest intake was seen with the “hard minimally processed” meal and the most energy was consumed with the “soft ultra-processed” meal with a difference of ~300 kcal [34]. These findings show that UPF can lead to faster ingestion and thus facilitate overconsumption. Comparing the instrumental texture properties from textural profile analysis with eating behaviors of solid foods identified increased springiness and chewiness as the textural food characteristics that contribute to a reduced eating rate [35]. These texture properties could be used to design special “slow UPF” that prevent overconsumption.
The proposed mechanism behind the association between ad libitum energy intake and energy density, eating rate or food texture is the oro-sensory exposure (OSE) time during oral processing of food (i.e., mastication and lubrication of the food until swallowing [36]). Increasing the time a food spends in the mouth during oral processing as well as the number of chews required per bite (i.e., harder foods) directly slow energy intake rate and thus reduce ad libitum energy intake [31, 32, 37–39]. For example, adding peach gel particles to yoghurt and thus increasing the OSE due to chewing decreased the eating rate by up to 60% compared to homogenous yoghurt in young and elderly healthy adults without differences in palatability ratings [37]. In another intervention increasing OSE by food composition (chocolate custard with caramel fudge, high OSE instead of chocolate custard with caramel sauce, low OSE), reduced ad libitum food intake [38]. The underlying mechanism is the direct effect of OSE to food on satiation by signaling to the brainstem and further to higher cortical regions as described in detail in a recent review [40]. Hence, the sensory stimulation by a prolonged OSE mediates earlier satiation by brain signaling leading to earlier meal termination and thus resulting in a reduced ad libitum food intake. On the other hand, peripheral mechanisms like gastrointestinal hormone responses or gastric signaling were less affected by OSE [40].
High palatability of UPF may lead to overfeeding
In addition to the above-mentioned properties, UPF are also engineered to maximize palatability and therefore consumption [41]. Naturally occurring foods contain one primary nutrient inducing the palatability of the food. In contrast, UPF with maximized palatability, so called “hyper-palatable foods (HPF)”, contain several palatability-inducing nutrients in combinations that are not typically found in nature, e.g., simultaneous high amounts of fat and sugar [42]. HPF have been defined as rich in (i) fat and sodium, (ii) fat and simple sugars, or (iii) carbohydrates and sodium with specific contributions to the energy content of the food [43]. Applying this definition to the Food and Nutrient Database for Dietary Studies, revealed that 62% of foods in the US may be hyper-palatable. It was also shown that rather the food processing, than the food item category is crucial for hyper-palatability. For example, foods that would not intuitively be attributed as HPF, e.g., vegetables cooked in creams or sauces also fall within the definition of HPF [43]. What makes the consumption of HPF problematic is the ability to modulate feeding-related neural systems and food-seeking behavior by inducing reward and thus leading to overconsumption and ultimately to weight gain [44]. In a recent review, the reinforcing natures of HPF showed strong evidence that they may induce behaviors similar to that seen in drug abuse. The highly rewarding experience of HPF-consumption is suggested to be responsible for delayed satiety mechanism which in turn lead to excess energy intake with HPF [45]. HPF have been suggested as potential target substance of food addiction already a decade ago as they share several similarities with addictive drugs [46].
High sodium content of UPF not only effects hyper-palatability but may also contribute to NAFLD. This is supported by the Prevention of Renal and Vascular End-Stage Disease (PREVEND) study that found that a higher sodium intake assessed by 24h-urinary sodium excretion was associated with fatty liver index [47]. The author suggested that insulin resistance (IR) may represent a metabolic intermediate in explaining the relationship of higher sodium intake with NAFLD.
Low protein content of UPF may lead to overfeeding
Another potential mechanism by which UPF may lead to excess energy intake is the lower protein content seen in UPF compared to less processed foods (9.5% vs. 25.3%) [48]. Calories from protein are more expensive than from fat or carbohydrates, thus the food industry has a financial incentive to replace calories from protein with cheaper alternatives and therefore dilute the protein content of UPF [49]. According to the protein-leverage hypothesis, individuals show a protein-specific appetite trying to maintain a fixed protein intake. If the protein density of the diet consumed is low, the amount of food ingested will be increased to maintain absolute protein intake regardless if this means to increase total energy intake above the needs due to higher intake of fat and carbohydrates [50]. Protein dilution in UPF could therefore lead to excess energy intake when consuming a high proportion of UPF in an attempt to maintain protein intake. In line with the protein-leverage hypothesis, analyzing data from the National Health and Nutrition Examination Survey 2009–2010, it has been shown that with a higher percentage of energy intake from UPF the percent of total energy intake from protein decreased and total energy intake increased while the absolute protein intake remained relatively constant [48]. In the study by Hall et al. [20], it was calculated that protein leverage could potentially have explained up to ~50% of the observed difference of ~500 kcal/day in energy intake between the ultra-processed and unprocessed diet.
In summary, UPF may lead to excess energy intake by a high energy density, a soft texture that promotes a higher eating rate, a low protein content and hyper-palatability [51]. This excess energy intake with UPF may lead to weight gain and ultimately to the development of overweight and obesity and thus also increases the risk for NAFLD. However, not only physiological mechanism may increase UPF consumption, but also the fact that UPF are easily accessible and highly convenient, mostly being ready-to-eat or ready-to-heat products that may promote snacking behavior. They also provide inexpensive calories compared to unprocessed foods and have a long shelf-life [52]. These factors contribute to a regular consumption and a higher proportion of UPF in the diet.
Consumption of UPF may lead to NAFLD by low quality of dietary fat (i.e., high intake of saturated FA and a low intake of polyunsaturated FA)
There is evidence that excessive intake of saturated FA (SFA) with pervasive UPF consumption contributes to the explanation of adverse health outcomes [53, 54]. In population based case-control and prospective studies, Western dietary patterns with a high SFA content are associated with higher risk and prevalence of liver fat and NAFLD (for review see [55]) whereas a plant-based dietary pattern rich in poly-unsaturated FA (PUFA) was associated with lower NAFLD risk [56]. The adverse effect of high dietary intakes of SFA on hepatic fat deposition was independent of energy balance because it was not only observed in hypercaloric [57–59] but also in isocaloric dietary intervention studies [60–62].
Nutritional survey data from different countries revealed that contemporary diets with relatively higher contribution of UPF are characterized by higher contents of SFA [53, 63]. This relationship is not supported by a prospective study in British children in which UPF consumption was associated with lower calculated intake of fat, and particularly saturated fat [64]. UPF are, however, often characterized by a high quantity of fat and, by definition, also by a large proportion of highly processed dietary fats and oil [65, 66]. SFA and mono-unsaturated FA (MUFA) were predominant in fast food and ready-to-eat food items, while PUFA were abundant primarily in seafood UPF meals [65]. SFA was the second major content of FA of all UPF categories with 37.9%, just behind the MUFA (38.6%). SFA content increases stability and thus extends shelf life as compared to unsaturated FA [63].
Palm oil accounts for more than half of all vegetable fats and oils consumed [67]. It has technological and chemical properties that allow a broad use in food processes and it guarantees neutral taste, fragrance and defined texture in the food products [68–70]. Refined palm oil can be fractionated into a liquid olein fraction with better cold stability and a solid stearin fraction with better melting properties [71]. Palm stearin is preferentially utilized as a baking fat because of its stable β’-polymorphic form [72]. The use of palm oil by the food industry has markedly increased in the last few decades [73]. Palm oil and palm kernel oil contain 50% and 85% SFA, respectively [68]. In healthy persons, a single oral dosage of 80–92 g palm oil during a hyperinsulinemic-euglycemic clamp led to a rapid increase in liver fat (+35% 4 h after ingestion of the palm oil) as well as increases in hepatic and peripheral IR compared to vehicle (still water) [74].
Impaired insulin signaling or IR is discussed to be central in the pathogenesis of NAFLD and there is evidence that SFA adversely affect this process [75, 76]. In a cross-over dietary intervention in 16 males with overweight [47.9 years ± 1.1 years, BMI 27.7 kg/m2 ± 0.4 kg/m2, as mean ± standard error of the mean (SEM)] that compared a high SFA vs. high sugar diet for four weeks each, postprandial glucose and insulin responses were exaggerated in the SFA as compared to the sugar diet indicating impaired insulin metabolism [61]. This was paralleled by an increase in hepatic fat storage with the SFA but not with the sugar diet. Another dietary intervention study in 67 subjects with abdominal obesity (66% women, 50–64 years, 15% with diabetes, 31% using antihypertensive drugs, 16% using lipid-lowering drugs) comparing high SFA vs. high PUFA intakes found increased fasting insulin concentrations with the SFA diet indicating impaired insulin sensitivity and liver fat enrichment as compared with a diet rich in PUFA [60]. Three-week overfeeding with a SFA rich diet in 38 individuals with overweight (21 women and 17 men, 48 years ± 2 years, BMI 31 kg/m2 ± 1 kg/m2, liver fat 4.7% ± 0.9%) increased hepatic fat content by stimulating adipose tissue lipolysis and inducing IR which may be mediated by plasma ceramides that were also increased [57]. Proposed mechanisms of ceramides increasing lipid accumulation are stimulation of hepatic fat uptake as well as de novo lipogenesis [59] but also stimulation of adipose tissue lipolysis and induction of IR [57].
Other pathways contributing to liver fat accumulation with a diet high in SFA and low in unsaturated FA involve an imbalance between hepatic lipid uptake, de-novo lipogenesis and removal [77]. Interestingly, a diet high in SFA led to increased liver fat without increasing hepatic de-novo lipogenesis [61]. This was confirmed in a two-week cross-over dietary intervention with constant macronutrient relation that showed an increase in hepatic fat content with a high SFA and high glycemic index diet without affecting de-novo lipogenesis or FA oxidation [62]. By contrast, eight weeks of an isoenergetic high MUFA diet (40% carbohydrate, 42% fat whereof 28% MUFA) led to reduced liver fat in a controlled randomized study in type 2 diabetic patients [78] presumably due to high postprandial hepatic FA oxidation [79]. Similarly, eight week supplementation of omega-3 FA was shown to decrease liver fat by increasing dietary FA oxidation and downregulating hepatic de-novo lipogenesis [80]. Enrichment of food products such as bread, milk products, margarine and eggs with long-chain n-3 PUFA has, however, become less popular due to the largely disappointing results from studies investigating the use of n-3 FA for the primary and secondary prevention of cardiovascular disease [81].
Consumption of UPF may lead to NAFLD by low quality of carbohydrates (i.e., high glycemic load and high fructose content)
Since insulin is known to stimulate sterol regulatory element binding protein 1c (SREBP1c) and thus lipogenesis [77], an insulinogenic diet can promote development of NAFLD. Minimally processed foods led to a lower postprandial glycemia and thus a lower insulin demand compared to UPF and an increased level of food processing was associated with higher glycemic responses [82, 83]. The underlying mechanism includes that food processing with high pressures and temperatures or intense refining leads to deconstruction of the food matrix structure, and thus to a higher degree of starch gelatinization that increases postprandial glycemia. In contrast, reformulation of UPF in a low-carbohydrate, high-protein version can produce a significantly lower glycemic response reducing the risk to develop NAFLD whilst maintaining the high palatability valued by consumers [84].
In line with this, in a cross-over study including 13 adults with overweight and obesity (67 years ± 6 years, BMI 29.5 kg/m2 ± 1.9 kg/m2), simultaneous improvements in carbohydrate and fat quality by reducing glycemic index (60 vs. 36) and replacing SFA with PUFA (15% SFA vs. 5% SFA) for just two weeks led to drastic improvements on postprandial glycemia and liver fat content (–28%) even at unchanged energy content and percentage of total fat and carbohydrate [62].
Although low in glycemic index, excessive intake of fructose by sugar sweetened beverages (SSB) and possibly also by other processed foods has been linked to increased risk of developing NAFLD [85]. In a systematic review and meta-analysis of controlled trials on the effect of fructose-containing sugars from different food sources on NAFLD markers it was shown that total fructose-containing sugars from SSB but not from other food sources increased liver fat in addition trials and decreased aspartate transaminase (AST) in subtraction trials with no effect on any outcome in substitution or ad libitum trials [86]. When consumed in positive energy balance, fructose-sweetened beverages promote hepatic de novo lipogenesis, liver fat accumulation, dyslipidemia, and IR compared to glucose-sweetened beverages [87, 88].
Consumption of UPF may lead to NAFLD by dietary components promoting dysbiosis and leaky gut
Dysfunction of the gut-liver axis is a generally accepted mechanism for the progression of NAFLD [89] that is substantially influenced by the diet. Intestinal factors of increased portal endotoxin levels include impaired barrier function (leaky gut syndrome) and dysbiosis. Exposure of the liver to endotoxins from intestinal bacteria, antigens, pathogens and other proinflammatory substances leads to an increase of inflammatory cytokines as observed in animal models and humans which are expected to aggravate the progression of NAFLD early on [89].
Food processing and formulation may contribute to dysbiosis and impaired barrier function through various mechanisms. Non-caloric sweeteners (e.g., saccharin, sucralose, aspartame) that improve the taste and texture of food as well as advanced glycation endproducts (AGE) generated by thermal processes from amino acids and sugars can alter gut microbial metabolism [90–93]. Emulsifiers (i.e., lecithin, mono-and diglycerides of FA, guar gum, xanthan gum, carrageenan, celluloses, and polysorbates) that are common in bakery, dairy, beverages, ice cream, sauces, chocolate, and other foods are suspected to impair the diversity of gut microbiota (decrease in anti-inflammatory genera like Akkermansia and promotion of proinflammatory genera like Escherichia, Roseburia, Bradyrhizobium, and Turicibacter) [94]. In addition, emulsifiers may stimulate inflammatory pathways [95], impair the function of the mucus layer and the tight junctions [96]. High consumption of UPF is therefore suspected to play a causal role in several diseases associated with dysbiosis and impaired intestinal permeability, such as cardiovascular and microvascular diseases like chronic kidney disease, obesity, type 2 diabetes, and cancer [97–99].
Promising strategies to prevent or treat dysbiosis and leaky gut syndrome are enrichment of processed foods with phytochemicals like polyphenols and prebiotics/dietary fibers, like inulin, fructo-oligosaccharides, galacto-oligosaccharides, isomalto-oligosaccharides, xylo-oligosaccharides, arabino-xylo-oligosaccharides, pectic-oligosaccharides, or resistant starch. Per definition, prebiotics are “selective fermented ingredients that allow specific changes, both in the composition and/or activity in the gastrointestinal microflora that confer benefits upon host well-being and health” [100]. It is expected that prebiotics promote the abundance of beneficial bacteria, like Bifidobacterium, Lactobacillus, and Faecalibacterium prausnitzii [101–103] that improve barrier function and gut health by increasing the production of short-chain FAs and decreasing endotoxins levels [104].
UPF are generally low in phytochemicals and enrichment of these food products with polyphenols like quercetin, epigallocatechin gallate, curcumin as well as carotenoids, phytosterols, and others may help to increase the abundance of beneficial bacteria and reduce intestinal permeability or exhibit antioxidant and anti-inflammatory properties (for a review see [105]) that contribute to the prevention and treatment of NAFLD.
In addition, enrichment of UPF with so called plant lipotropes like choline, betaine, myo-inositol, methionine and carnitine could facilitate the removal of fat from the liver [i.e., by promoting phospholipid synthesis for very low density lipoprotein (VLDL) secretion], reduce hepatic lipid synthesis and increase FA oxidation [106, 107]. However, evidence from human intervention studies that confirm the capacity of these components to prevent or treat individuals with NAFLD is needed.
Proposed molecular mechanisms
UPF are often high in sugar, low in dietary fiber, natural antioxidants, plant bioactives and micronutrients. At the same time UPF may contain substantial amounts of saturated fats (Figure 1). Collectively this may result in chronic low-grade metabolic inflammation [108] as observed by an increased nuclear factor k-light-chain-enhancer of activated B cells (NFκB) activity in laboratory rodents [109]. The transcription factor NFkB regulates the production of proinflammatory cytokines such as tumor necrosis factor alpha (TNF-α), interleukin 1 beta (IL1β), interleukin 6 (IL6) and chemoattractants including monocyte chemoattractant protein 1 (MCP1) amongst others (Figure 2). Similarly, a positive association between UPF consumption and C-reactive protein levels (as a proxy of chronic inflammation) as well as other biomarkers of inflammation (e.g., IL1β, IL6, MCP1) has been observed in several studies in children and adolescents [110–112].
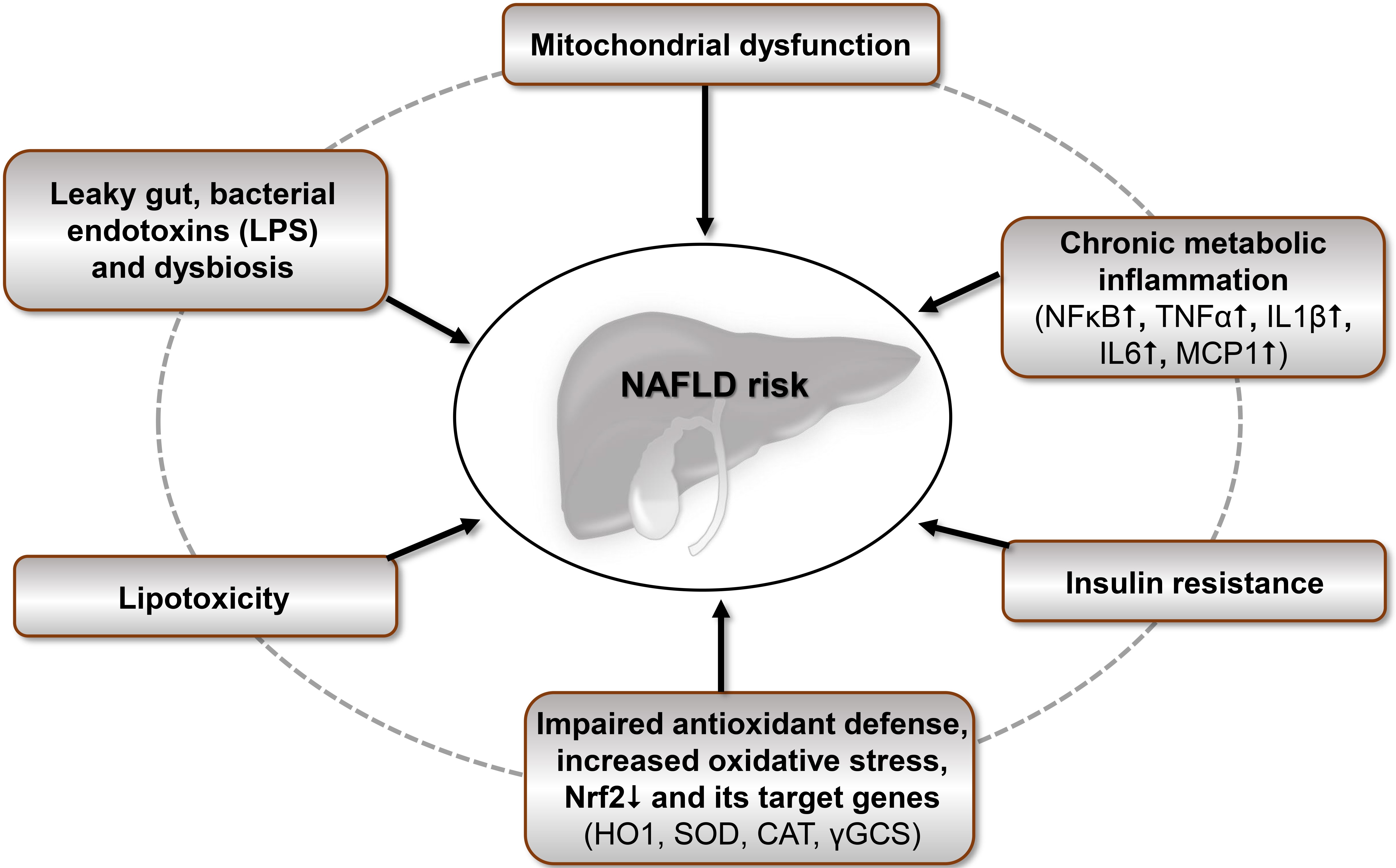
Underlying mechanisms, by which UPF increase NAFLD risk. CAT: catalase; HO1: heme oxygenase 1; LPS: lipopolysaccharide; Nrf2: nuclear factor erythroid 2-related factor 2; SOD: superoxide dismutase; γGCS: γ-glutamylcysteine-synthetase. ↑: High/increase; ↓: low/decrease
Furthermore, fiber-poor UPF as part of the so-called Western type diet can result in dysbiosis and impaired gut integrity. A leaky gut increases the concentration of bacterial endotoxins (e.g., LPS) known to trigger the above mentioned proinflammatory signal transduction pathways [113] of the gut-liver-axis partly via toll like receptor signaling [114].
The low dietary supply of natural antioxidants (e.g., vitamin C, vitamin E, carotenoids), plant bioactives (e.g., polyphenols, isothiocyanate derived glucosinolates), micronutrients (e.g., zinc, selenium) via UPF affects the cellular oxidant/antioxidant homeostasis. This in turn may induce cellular redox stress which interferes with Nrf2 signaling. The transcription factor Nrf2 orchestrates the expression of genes encoding important antioxidant and stress proteins [115–117] such as HO1, SOD, CAT and γGCS—the latter is the rate regulating enzyme of endogenous glutathione (GSH) synthesis a potent cytosolic antioxidant.
Redox stress as well as an overflow of FFA due to excessive UPF consumption leads to hepatic lipotoxicity, mitochondrial dysfunction, beta cell death and ultimately to IR [118] which contribute to the pathogenesis of hepatic injury and NAFLD as summarized in Figure 2. Furthermore, it is plausible to assume that UPF affects autophagy, proteasomal activity and endoplasmatic reticulum stress which warrants further systematic investigations.
Conclusion
Overall, literature data clearly indicate that UPF increase NAFLD risk. The nutritional quality of processed food could be improved due to enrichment with fiber, prebiotics, phytochemicals and protein with a concurrent reduction in glycemic load, energy density, SFA, fructose and food additives. Future studies are needed to examine the interactive and protective effects of such modifications in the composition of UPF on prevention and treatment of NAFLD. Furthermore, complimentary studies in cultured cells and laboratory rodents are needed to systematically elucidate the underlying molecular, cellular and physiological mechanisms by which UPF and its constituents affect NAFLD risk. In addition, prospective as well as sufficiently powered long-term human intervention studies addressing the role of UPF in the context of NAFLD and other chronic diseases should be conducted in the future. Finally, the identification of robust biomarkers which are indicative for UPF consumption in humans is crucial to better pinpoint UPF consumption in the population.
Abbreviations
BMI: | body mass index |
FA: | fatty acids |
HPF: | hyper-palatable foods |
IL1β: | interleukin 1 beta |
IL6: | interleukin 6 |
IR: | insulin resistance |
MCP1: | monocyte chemoattractant protein 1 |
MUFA: | mono-unsaturated fatty acid |
NAFLD: | non-alcoholic fatty liver disease |
Nrf2: | nuclear factor erythroid 2-related factor 2 |
OSE: | oro-sensory exposure |
PUFA: | poly-unsaturated fatty acids |
SFA: | saturated fatty acids |
UPF: | ultra-processed food |
Declarations
Author contributions
FAH and JE: Writing—original draft, Writing—review & editing. GR: Writing—original draft, Writing—review & editing, Visualization. ABW: Writing—original draft, Writing—review & editing, Conceptualization, Supervision. All authors read and approved the submitted version.
Conflicts of interest
The authors declare that they have no conflicts of interest.
Ethical approval
Not applicable.
Consent to participate
Not applicable.
Consent to publication
Not applicable.
Availability of data and materials
Not applicable.
Funding
Not applicable.
Copyright
© The Author(s) 2023.