Abstract
Traditional medicines and their active ingredients and some natural products and derived analogs have been used for treating multiple diseases including cancer. Among these compounds cytotoxic agents such as bleomycin, paclitaxel and vincristine block essential pathways and genes required for cancer cell growth and these agents have diverse clinical applications. Dietary phenolics including flavonoids and related compounds are associated with multiple health benefits however most individual dietary compounds and other natural products that show promising anticancer activity in preclinical studies exhibit minimal clinical effectiveness and this is particularly true for cancer. Many of the compounds perform poorly in clinical trials due to pharmacokinetic consideration and limited uptake (e.g., curcumin) and these are issues that can be addressed. The clinical effectiveness of flavonoids and many other natural product-derived anticancer compounds can also be enhanced by a more targeted approach. This would include identifying a significant response/gene or target in a specific cancer and then identifying the optimal compound. In this review, I have discussed a limited number of targets including non-oncogene addiction genes such as Sp transcription factors, reactive oxygen species (ROS) or the orphan nuclear receptor 4A (NR4A) sub-family. Thus, the most active compound for these responses could be used only for treating patients that are ROS-inducible or highly express targets such as Sp1 or NR4A sub-family members. A mechanism-based precision medicine approach should enhance the clinical efficacy of dietary and related natural products as anticancer agents and decrease toxic side effects for some combination therapies.
Keywords
Phenolics, flavonoids, cancer, precision medicineIntroduction
Several anticancer drugs have been isolated from natural products and play an important role in cancer therapy. Many of these compounds such as paclitaxel and doxorubicin are plant or microbial-derived chemicals that inhibit cancer due to their mechanisms of cytotoxicity. There is also evidence that the diet can play a significant role in disease prevention and in some cases specific diets may exhibit chemotherapeutic activity. However, many dietary compounds such as polyphenolics, flavonoids and related phytochemicals exhibit minimal to modest effects in clinical trials despite their promising preclinical anticancer activities suggesting that studies on the mechanisms of action of these compounds may enhance their efficacy as precision medicines. This review discusses examples of this approach with dietary compounds and natural products that act through targeting specificity protein (Sp) transcription factors (TFs), inducing reactive oxygen species (ROS) and binding the orphan nuclear receptor 4A1 (NR4A1).
Phytochemicals and derived compounds: health benefits and anticancer activities
Background
Traditional medicines have been a major form of health care for several different societies, and they include Chinese medicines, Indian medicines (Ayurveda, Unani), Japanese (Kampo), traditional Korean (SCM, TKM), traditional aboriginal (Australia), African and Russian medicines. Most of these medicines are from herbal or plant extracts, and other natural product medicines and drugs are also derived from microbial, marine and other sources [1–9]. These medicines usually contain complex mixtures of individual compounds and in many cases, a single or only a few constituents have been associated with the activity of the mixture. The effects of traditional medicines on multiple diseases are being extensively investigated and a PubMed research (Feb, 2024) under “Traditional medicines” lists over 290,000 manuscripts including approximately 3,000 publications that appeared on-line during the January-February period of 2024. The focus of these articles is variable but includes evidence for the efficacy of traditional medicines in treating multiple diseases. A recent report summarized results of several studies on the treatment of gastric ulcers with traditional Chinese medicines (TCM) and other drugs [2]. Most of the referenced studies showed that both individual drugs and traditional medicines improve health conditions in rodent model studies. However, direct comparisons of the efficacy of TCM vs individual drugs were not clear; nevertheless, it was concluded that the multiple activities exhibited by the chemical ingredients of herbal medicines contributed to their effectiveness in treating gastric ulcers and comparison of “active” drugs vs mixtures present in traditional medicines need to be further investigated. It is evident that many natural products are highly effective drugs for clinical applications however, for both herbal medicines and health promoting diets, the individual compounds associated with the activity have had only limited clinical applications.
Clinically active natural product-derived anticancer agents
Clinically used agents
Traditional medicines and some of their major active component(s) have had a highly significant impact on treatment of several diseases including cancer where natural products and their derivatives play an important role in cancer chemotherapy [10–17]. For example, several key plant-based anticancer agents that are used in the clinic are illustrated in Figure 1. These included paclitaxel (Taxus brevifolia bark), camptothecin (Camptotheca acuminata Decne), vinblastine and vincristine (Vinca Rosea L.) and podophyllotoxin (Podophyllum) [14]. These phytochemicals and their synthetic analogs have well defined mechanisms of action that are usually linked to inhibition of important pathways enabling cancer cell proliferation and viability. Paclitaxel targets microtubules which leads to mitotic arrest; camptothecin is a topoisomerase I inhibitor that enhances DNA damage and apoptosis in cancer cells; vinblastine acts as a mitosis inhibitor that binds microtubular proteins and podophyllotoxin also binds tubulin and inhibits topoisomerase II resulting in mitotic arrest. These phytochemicals and other synthetic analogs are highly cytotoxic and are extensively used alone and in combination therapies for treating multiple cancers.
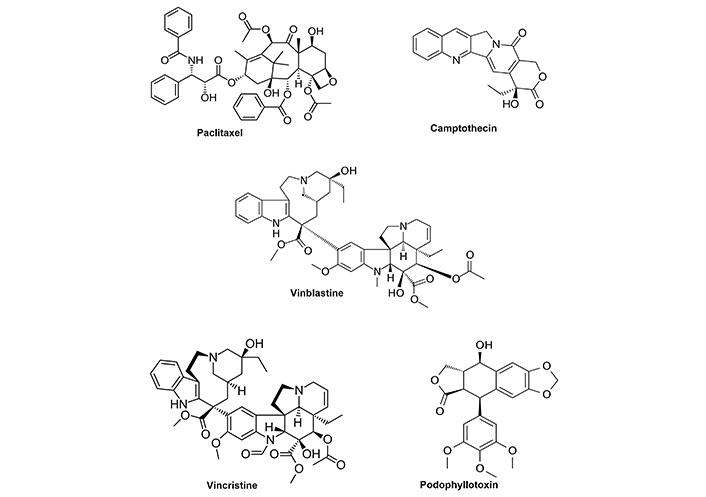
Clinically used anticancer agents derived from plant sources. Structures of 5 key plant derived compounds that are clinically used for cancer chemotherapy. Synthetic analogs of these compounds including other taxanes are also extensively needed
Several other anticancer agents are microbial products (Figure 2) including bleomycin, actinomycin D, mitomycin C, doxorubicin, pentostatin and daunorubicin (Streptomyces), carfilzomib (Actinomyces) [12] and drugs such as bleomycin bind DNA resulting in subsequent DNA damage. Actinomycin D intercalates DNA and inhibits cell proliferation; mitomycin C also alkylates DNA to inhibit cell growth; doxorubicin also intercalates DNA and induces cell death; pentostatin is an antimetabolite that binds and inhibits adenosine deaminase; daunorubicin also intercalates DNA and inhibits topoisomerase II activity and carfilzomib is a proteosome inhibitor. This group of microbial-derived anticancer agents and/or their derivatives are clinically proven drugs and there is an ever-expanding list of microbial metabolites that are currently being investigated for their potential clinical applications as cancer chemotherapeutics. It should be noted that activities of these compounds are related to their well defined effects on “critical/susceptible” pathways in cancer cells that include DNA damage/inhibition of repair, targeting microtubules and mitosis and inhibition of proteasomes. In addition, there is also evidence that some natural products from marine sources show promise as anticancer agents [12, 18]. It is anticipated that additional new and promising natural product-derived anticancer agents will also be approved for clinical applications in which they retain the efficacy of their precursors but with decreased toxic side-effects [12].
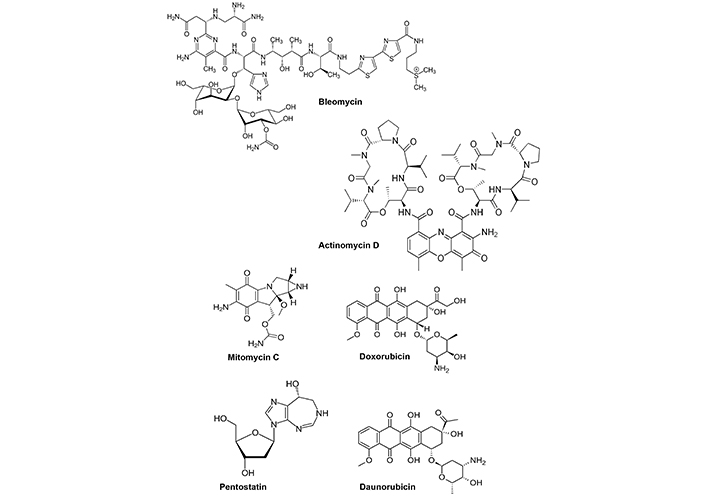
Microbial derived anticancer agents used for clinical application. Structures of microbial metabolites that are clinically used for cancer chemotherapy
Herbal/traditional medicine-derived natural products with limited activity
Yuan and coworkers [9] summarized a number of natural products derived from Chinese herbal medicines in which the individual compound and the herbal medicine are used for treating the same disease. These structurally-diverse herbal medicine-derived drugs and their targeted disease include; puerarin (diabetes), salvianolic acid B (cardiovascular and cerebrovascular diseases), rhynchophylline (antihypertensive), costunolide (anti-gastric ulcer, antispasmodic), gastrodin (anti-convulsion, analgesic), artemisinin (anti-malarial), tetrahydropalmatine (analgesic), tetramethylpyrazine (myocardial ischemia-reperfusion injury), paeoniflorin (analgesic), icariin (osteoporosis). However, even though their preclinical effects (in vitro/in vivo) look promising the clinical applications and effectiveness of these “active” of components of TCM is limited. For example, puerin is poorly absorbed with low bioavailability which “limits its application in the clinic” [19]; for salvianolic acid B the “low bioavailability is a serious obstacle” [20]; for gastrodin “more detailed clinical trials are still in need” [21]; costunolide exhibits activities but limited clinical data [22]; few clinically relevant studies have been determined for rhynchophylline [23]. For paeoniflorin the anti-depressive activity is promising but “more high quality pre-clinical and clinical studies are expected” [24]; tetramethylpyrazine is “a promising drug with great research potential” [25]. Thus, many of these compounds display promising preclinical activities for treating one or more diseases including cancer, but their clinical applications are limited or non-existent. They exhibit highly diverse structures and their mechanisms of action are not well defined. This does not necessarily mean that these compounds and other natural products will not be clinically useful however extensive studies are required to identify their mechanisms of action and specific disease targets as previously illustrated in the discovery of taxol derivatives [13].
Health benefits and anticancer activities of dietary-derived phytochemical mixtures
As indicated above, many important chemotherapeutic agents for single and combined treatments have been discovered from plant-derived traditional medicines, microbial metabolites and marine sources. Another significant source of human exposure to potential therapeutic agents are dietary compounds, which have been associated with health promoting activities (e.g., chemoprevention) and with their activities as therapeutic agents [26]. Population studies have identified specific diets and dietary components that have significant health impacts and the Mediterranean diet stands out as an example [27–36]. The enhanced health of individuals on this diet is similar to that observed for other diets that are high in fruits, vegetables and nuts, whole grains, olive oil, moderate amounts of yogurt, cheese, fish and eggs, smaller amounts of red and white meat and low amounts of alcohol [27]. For example, comparisons between individuals on the Mediterranean diet and on so-called “Western” diets show that the former diet is associated with increased longevity, decreased cardiovascular diseases, lower rates of some cancers and lower rates of neuronal dysfunction including cognitive decline, Alzheimer’s disease and other neurotoxicities [26–40]. Coffee is another dietary component and is the most widely consumed beverage; increased consumption of up to 5 cups of coffee per day correlated with many of the same health benefits observed for the Mediterranean diet [41]. Only a few studies have investigated interactions of a Mediterranean diet and coffee consumption: In a case control study, effects of coffee and diet on colorectal cancer gave equivocal results [42] whereas in a randomized trial moderate coffee consumption and a Mediterranean diet were associated with decreased body fat in 1,483 participants with metabolic syndrome and on a Mediterranean diet [43]. In addition to the health benefits associated with some diets and coffee consumption [44–51] these health effects have also been correlated with relative serum levels of individual classes of dietary components. For example, a study in a Spanish population showed an inverse association between dietary phenolics and cardiovascular disease [49]. This association was also observed for specific phenolic sub-groups including dihydroflavonols, flavonols, alkyl methoxyphenols, hydroxy-cinnamic acids, hydroxyphenyl acetic acid (Figure 3). A dose-response meta-analysis of several prospective cohort studies also showed an inverse association between polyphenolic subgroups and the risk of type 2 diabetes [52]. Inverse associations were observed for flavonoids, flavanols, flavan-3-ols, catechins, anthocyanidins, isoflavones, daidzein, genistein, and stilbenes. Analysis of two other prospective studies showed that higher consumption of phytoestrogens was also associated with decreased mortality from cardiovascular disease and lower risks also correlated with higher intakes of isoflavones, lignans and coumarins [53].
The association of individual and sub-classes of polyphenolic and other phytochemicals on risk for various cancers has been extensively investigated and potential mechanisms have been proposed [54–66]. In most of these studies the chemopreventive effects are linked to the antioxidant and anti-inflammatory activities of polyphenolics (Figure 3) and many other pathways may also be important. An extensive meta-analysis of case-control and prospective studies indicates that consumption of dietary flavonoids is inversely related to risks of lung and stomach cancers and decreased risk for breast and colorectal cancer were observed but not significant [64]. Studies on clinical applications of dietary-derived phenolics show that these mixtures and individual compounds can be used alone or with others and provide health benefits. For example, a combination of luteolin, quercetin and rutin decreased some behavioral symptoms in children autism spectrum disorder [67]; higher tea intake and some flavonoids were inversely related to hospitalization of elderly women at a high risk for fracture [68]. These studies confirm that both diets and sub-classes of dietary phytochemicals are associated with some health benefits. Many individual dietary-derived natural products and related compounds show promising preclinical activity whereas their clinical effects are primarily modest or not observed.
Dietary-derived natural products and related compounds as anticancer agents
While it is clear that the Mediterranean and other diets enriched in fruits, nuts, vegetables, grains and polyunsaturated fatty acids are chemopreventive in terms of both non-cancer and cancer endpoints, their cancer chemotherapeutic activities are much less impressive. For example, a comprehensive review of dietary interventions in the treatment of cancer patients concluded that “Evidence demonstrated little effects of dietary interventions on overall mortality and secondary cancers” [69]. A phase II study reported that soy isoflavones did not increased survival of patients with advanced pancreatic cancer being treated with gemcitabine and erlotinib [70]. Thus, evidence for dietary polyphenolics as enhancers of anti-tumor activity in patients being treated with standard-of-care anticancer agents is lacking, however, success might be increased using a more mechanism-based approach where the polyphenolics are targeted to specific pathways. Another study systematically reviewed phase I and phase II clinical trials of individual flavonoids as anticancer agents [60]. Most of the flavonoids exhibit promising preclinical activity in cell culture and animal models but exhibit minimal clinical activity although some synthetic flavonoids such as flavopiridol gave promising results for blood-derived but not solid tumors. The overall impacts of dietary flavonoids and other polyphenolics as cancer chemotherapeutics in clinical studies are minimal but can be enhanced with improved formulations and drug delivery. In contrast to the less than favorable clinical results obtained for flavonoids, there is extensive ongoing research on flavonoids and other natural products as anticancer agents and under the heading “Flavonoids as Anticancer Agents” in PubMed over 31,000 papers are listed and approximately 200 have been published in January–March, 2024. Quercetin alone (on PubMed) has over 3,300 papers listed. There is concern that continued preclinical testing with these compounds will not improve clinical outcomes until their mechanisms of action are determined.
Curcumin, a polyphenolic stilbene compound is an important traditional Indian medicine that is being developed for treating both non-cancer and cancer endpoints [71–73]. Under a search for “Curcumin an Anticancer Agent” over 16,000 papers are listed on PubMed for this compound alone. Problems associated with the therapeutic effects of curcumin include poor bioavailability and this is being addressed in ongoing studies by improving formulations and delivery systems for this agent [71]. A recent review paper on clinical trials of curcumin for cancer therapy concluded that “available clinic evidence is not strong enough to support the therapeutic use for curcumin in cancer” [73]. However, it is conceivable that with the discovery of more effective delivery of curcumin or a synthetic analog that activities may be sufficiently increased for clinical efficacy. It is also possible that the efficacy of curcumin and related natural products can be improved in cancer chemotherapy by more precision targeting and this will be discussed in the following section.
Phytochemicals and natural products as anticancer agents—examples of mechanism-based precision targeting of Sp transcription factors, reactive oxygen species and orphan nuclear receptor 4A1
Background
While it is clear that some natural products such as the taxanes and microbial metabolites are mainstays as cancer chemotherapeutics (Figures 1 and 2) many other natural products that exhibit promising preclinical anticancer activity in cell culture and in vivo are not effective in human clinical studies. Most of the clinically used natural products target critical genes and pathways required for DNA integrity and tumor growth and this includes effects on microtubules, DNA damage and DNA repair enzymes. In contrast, many other natural products such as dietary and other polyphenolics tend to target cell context-dependent multiple proteins/pathways in cancer cells but do not exhibit therapeutic efficacy in human clinical trials. This problem will be difficult to resolve for natural products, but one possible solution is to uncover additional critical targets in cancer cells and focus on patients that highly express these targetable genes and pathways. This will be discussed with examples in the following section.
Natural product anticancer agents that target Sp transcription factors
Sp TFs Sp1, Sp2, Sp3 and Sp4 are members of the Sp—Kruppel-like factor family which exhibit many common structural features [74–79]. These TFs are particularly important in early development and there is some evidence for a decrease in Sp1 with age [80, 81]. In contrast, Sp1, Sp3 and Sp4 (and possibly Sp2) are highly expressed in many tumors and cancer cells. Most reports on Sp TFs have focused primarily on Sp1 and it is possible that Sp3 and Sp4 may have similar effects but have not been as extensively investigated and there are even fewer studies on expression of Sp2 [81]. A focus on Sp TFs as an important druggable target in cancer is due to several factors including evidence that cellular transformation of a normal cell to a cancer cell is accompanied by increased expression of one or more Sp TFs [81–89]. For example, carcinogen or oncogene-induced transformation of human fibroblasts into fibrosarcoma cells was accompanied by an 8- to 18-fold increase in Sp1 protein expression. Moreover, knockdown of Sp1 in the fibrosarcoma cells abrogated their ability to form tumors in an athymic nude mouse model [82, 83]. Sp1 (primarily) or Sp3 and Sp4 are overexpressed in many tumors and are the negative prognostic factor for patients with multiple cancers including; liver, ovarian, lung, breast, colon, gastric, pancreatic, head and neck, bladder, prostate and esophageal cancers, glioblastoma and astrocytoma [75]. Sp TFs are associated with many other proteins, long non-coding RNAs and microRNAs that often play pro-oncogenic roles in cancers [78]. Moreover, based on results of knockdown studies in pancreatic cancer cells Sp1, Sp3 and Sp4 regulate genes/pathways that are responsible for cancer cell growth, survival and migration/invasion and Sp TFs have been referred to as non-oncogene addiction genes [90].
These properties suggest that drugs targeting Sp TFs in tumors would potentially be effective. Figure 4 illustrates structures of several compounds that inhibit tumor growth and exhibit functional characteristics similar to those observed after Sp knockdown. Interestingly some of these compounds such as celecoxib, panobinostat, metformin and bortezomib [91–94] already have other clinical applications and it can be assumed that their downregulation of Sp TFs contributes to the effectiveness of these compounds as anticancer agents. Moreover, many other natural products including betulinic acid, honokiol, quercetin and phenethyl isothiocyanate [95–103] which downregulate Sp TFs exhibit promising preclinical activity in cancer cells and rodent models but in clinical trials their effects are modest. The differences in lack of potency of natural product-derived compounds vs clinically used drugs (Figure 4A and B) could be due to several factors including pharmacokinetics and different mechanisms of drug-induced Sp-downregulation (Figure 4C). A summary of these mechanisms has previously been reported and discussed and this includes examples of where the phytochemical (e.g., murrayafoline [104] and quercetin [105]) directly bind Sp1 and decrease its activity. However, it is possible that the efficacy of these compounds may be enhanced by treating only patients that overexpress Sp1, Sp3 and Sp4. The clinical challenge for these natural products will be to identify optimal compounds for each tumor type and target the susceptible patient group overexpressing Sp TFs.
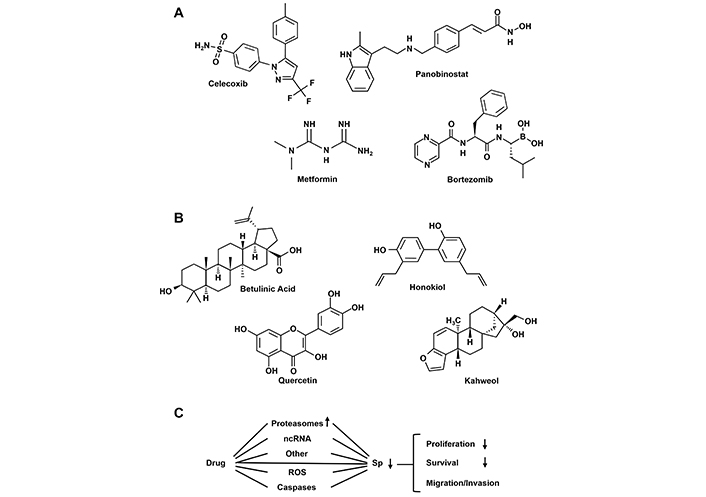
Drugs that target downregulation of Sp TFs. Structures of clinically used anticancer agents (A) and phytochemicals (B) that induce Sp downregulation and pathway associated with this response (C) [75]
Natural product anticancer agents that activate reactive oxygen species
The chemopreventive activities of dietary polyphenolics have been associated, in part, with their activity as antioxidants in normal cells that maintain low levels of ROS. In contrast, many of these some molecules that exhibit antioxidant activity in normal cells induce ROS in a cancer cell context and this contributes to their anticancer activity [60–62, 106–109]. The inverse activities of ROS and many natural phenolics as chemopreventive and chemotherapeutic agents also reflect the difference between normal tissue vs tumors where the high requirements for energy by rapidly proliferating tumors invariably are accompanied by higher levels of ROS. The flavonoids are examples of major dietary chemicals that may protect cells during growth as normal cells by their activities as antioxidants however many studies show that flavonoids such as phloretin, kaempferol luteolin, 3,5,7-trihydroxyflavone, quercetin, apigenin, acacetin, genistein, and isorhamnetin induce ROS in cancer cells [110–122]. Other studies do not report induction of ROS by flavonoids, and this may be due to lack of measurement of this response. However, it was reported that inhibition of melanoma cell growth by luteolin via effects on extracellular matrix and not by induction of ROS [123] whereas luteolin induced ROS and decreased mitochondrial membrane potential in rat liver tumor hepatocytes [112]. Moreover, some cytotoxic anticancer agents such as bleomycin and daunomycin induce ROS in non-tumor tissue as a toxic side-effect and this is in contrast to the effects of flavonoids. It is possible that the activities of flavonoids as ROS inducers may be both structure-dependent and tumor specific and these relationships have not been determined. Thus, precision targeting of tumors by ROS-inducing natural products must take into account several factors required for selection of optimal agents for a specific tumor.
A report by O’Hagan and coworkers [124] showed that after treatment of SW480 colon cancer cells with hydrogen peroxide, there was a chromatin-wide relocation of DNA methyltransferases from non-GC rich to GC-rich areas. This resulted in significant changes in gene expression and the cMyc gene which contains a GC-rich promoter was one of the genes downregulated by hydrogen peroxide. The oncogenic activity of cMyc has been well described and studies in this laboratory showed that a number of ROS inducing natural products including benzyl and phenethyl isothiocyanate also decrease cMyc expression in cancer cells [124, 125]. Figure 5 illustrates the critical role of cMyc in the ROS-induced response where downregulation of cMyc resulted in decreased expression of microRNA-27a and other microRNAs in the miR-17-92 complex. Decreased miRs resulted in the induction of at least 3 Sp-repressor proteins ZBTB4, ZBTB10 and ZBTB39 [103, 125, 126]. The ZBTB repressors competitively displace Sp TFs from GC-rich sites in Sp-regulated pro-oncogenic genes (including Sp TFs) and this is accompanied by Sp protein downregulation. Mechanistic studies show that in cancer cells knockdown of cMyc or cMyc-regulated miRs or overexpression of ZBTBs also results in downregulation of Sp TFs in the absence of ROS. These results suggest that the effectiveness of ROS-inducing natural products that trigger the cMyc-Sp TF pathway (Figure 5) could be enhanced in tumors overexpressing Sp1, Sp3 or Sp4. This drug-induced ROS-dependent downregulation of Myc by natural products has been observed for many compounds [126], however methods for selecting optimal ROS-inducing agents for targeting this response have not been determined and based on mechanism studies these compounds may also have increased clinical efficacy for treating patients that overexpress Sp TFs.
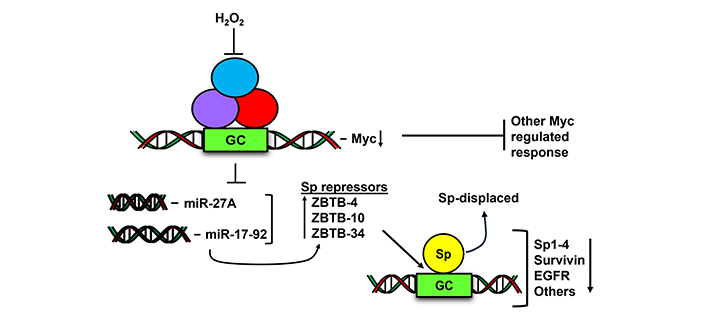
Hydrogen peroxide and ROS decrease Myc and Myc-regulate miRNA and this results in the induction of Sp repressors (ZBTB-10, 4, 34) which in turn competitively displace Sp transcription factors from GC-rich gene promoter sites
ROS induction of other “death” pathways in cancer
Natural products that induce ROS are cytotoxic to cancer cells and tumors however, this may be accompanied by several independent and overlapping pathways [106–109]. This may include induction of intrinsic and extrinsic apoptosis, DNA damage, endoplasmic reticulum (ER) stress, necroptosis, autophagy and ferroptosis. Ferroptosis is an iron-dependent form of cell death that is emerging as a therapeutic pathway for treating various cancers [127–131]. Ferroptosis-induced cell damage is characterized by enhanced lipoperoxidation and the subsequent cytotoxicity mediated by intracellular lipid hydroperoxides. Several genes are important for induction/repression of ferroptosis and these include: (I) glutathione peroxidase 4 (GPX4) which helps to maintain cellular glutathione levels and inhibits ferroptosis; (II) stearoyl coenzyme A (CoA) desaturase 1 (SCD1) which catalyzes formation of monounsaturated fatty acids that inhibit ferroptosis; (III) transferrin and the transferrin receptor (TFR) which facilitate iron import into the cell and enhance ferroptosis. There is evidence that structurally diverse natural products may be significant players in development of ferroptosis-based anticancer agents. Compounds that induce ferroptosis include curcumin, glycyrrhetinic acid, gallic acid, quercetin and other flavonoids, artesunate, piperlongumine, ursolic acid, capsaicin, honokiol, withaferin A and many other compounds and plant extracts [108, 132–135]. The mechanisms of action of these compounds are variable and include ROS induction and inhibition or activation of key ferroptosis-related gene products including TFR, SCD1 and GPX4. However, development of clinically effective ferroptosis inducers will require cancer type specific optimization of individual natural products and their preferred targets.
Phytochemicals that target nuclear receptor 4A
The NR superfamily of receptors play a major role in maintaining cellular homeostasis and in pathophysiology and these receptors are a major drug target for treating both cancer and non-cancer endpoints. The orphan NR4A family includes NR4A1 (Nur77, TRB), NR4A2 (Nurr1) and NR4A3 (Nor1) and endogenous ligands for these receptors have not yet been identified. NR4A play an important role for multiple functions including innate and adaptive immunity, immune cell differentiation, steroidogenesis, response to inflammation and stress, atherogenesis and central nervous system functions [136–138]. NR4A1 and NR4A2 exhibit pro-oncogenic activities in solid tumors and their derived cell lines and results of knockdown studies show that NR4A1 and NR4A2 regulate cell proliferation survival, migration and invasion (Figure 6). Studies in our laboratory have identified synthetic bis-indole derived compounds (CDIMs) that bind NR4A1, NR4A2 or both receptors and inhibit cancer cell proliferation, survival, migration and invasion [139, 140] (Figure 6). The effects of these compounds are comparable to effects observed after knockdown of NR4A1 or NR4A2 and they exhibit inverse NR4A1/NR4A2 agonist activity based on their functional effects. There are multiple reports on phytochemical anti-cancer agents that exhibit activities similar to that observed for the bis-indole derived NR4A1/NR4A2 ligands and this is particularly true for flavonoids. Based on their functional similarities it was hypothesized that the anticancer activities of the flavonoids quercetin and kaempferol might be due to interactions with NR4A1 and NR4A2 to block receptor-mediated pro-oncogenic activities. It was recently reported that both quercetin and kaempferol directly bound NR4A1 and acted as inverse NR4A1 agonists in rhabdomyosarcoma cells [141]. A subsequent study also showed that like the CDIM inverse NR4A1 agonists, both quercetin and kaempferol inhibited pro-endometriotic genes/pathways including fibrosis [142]. Subsequent studies show that over 20 structurally diverse hydroxyflavones also bind NR4A1 and other natural products including, broussochalcone, fangchinoline, tetrandrine, resveratrol, and piperlongumine are also NR4A1 ligands [143–146] that exhibit anticancer activities as illustrated in Figure 6. Previous clinical studies on flavonoids and other phytochemical NR4A1 ligands showed minimal effectiveness as anticancer agents. However, using a precision medicine approach, future studies on NR4A1-active flavonoids and related phytochemicals should first identify the optimal compound for a specific tumor type and then target cancer patients with tumors that overexpress NR4A1. This approach would also be relevant for phytochemicals that interact with other NRs. Moreover, several apoptosis inducing agents such as retinoids and phorbol esters induce nuclear export of NR4A1 which in turn activates pro-apoptotic pathways that also kill cancer cells [139].
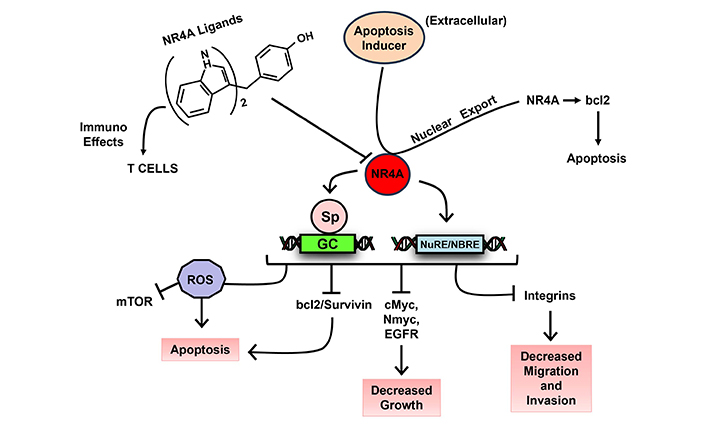
Mechanism of action of NR4A1/2 ligands. Prototypical NR4A1/2 ligands (e.g., CDIM, flavonoids and other polyphenolics) inhibit NR4A1/2-dependent pro-oncogenic pathways in tumor cells and reverse T cell exhaustion; apoptosis inducers activate nuclear export of NR4A1 to induce apoptosis [139]
Conclusions
Natural products and their derivatives have been an important source of several clinically effective agents for treating multiple cancers. However, many polyphenolics and other natural products, including phytochemicals in the diet, that exhibit promising preclinical anticancer activities are minimally effective in human clinical trials. These compounds need to be re-examined to determine their major mechanisms of action that can be used in a mechanism-based targeted approach that will more effectively inhibit tumorigenesis. In this review 3 major targetable pathways by phytochemicals have been discussed, namely downregulation of Sp TFs, induction of ROS and related death pathways, and binding to NR4A1. Other druggable targets also need to be determined. Having identified the most effective tumor cell type-specific target, compound screening should be carried out to identify the optimal compound and clinical studies should select a patient group that overexpresses or is responsive to the target gene/pathway. In the case of NR4A1 this would include patients with tumors overexpressing the receptor. A precision medicine approach using these natural products should significantly enhance the clinical outcomes of a more defined group of cancer patients and identify specific natural products suited for stand-alone or combination cancer therapies. The increased incorporation of natural products into cancer chemotherapies will also facilitate decreased toxic side-effects for some drug combinations.
Abbreviations
NR4A: | nuclear receptor 4A |
ROS: | reactive oxygen species |
Sp: | specificity protein |
TCM: | traditional Chinese medicines |
TFs: | transcription factors |
Declarations
Acknowledgments
I would like to acknowledge Amber Meyer for her assistance.
Author contributions
SS: Conceptualization, Investigation, Writing—original draft, Writing—review & editing.
Conflicts of interest
The author declares no conflicts of interest.
Ethical approval
Not applicable.
Consent to participate
Not applicable.
Consent to publication
Not applicable.
Availability of data and materials
The data that support the findings of this study are available from the corresponding author upon reasonable request.
Funding
This review was supported by the Syd Kyle Chair endowment, Texas A&M-AgriLife, and the National Institutes of Health [P30-ES029067]. The funders had no role in study design, data collection and analysis, decision to publish, or preparation of the manuscript.
Copyright
© The Author(s) 2024.