Abstract
Biochemist Svetlana Mojsov, both as a graduate student at The Rockefeller University under the mentorship of Bruce Merrifield during the 1970s, and as an independent investigator at the Massachusetts General Hospital (MGH) during the 1980s, devised effective and robust methods for the chemical synthesis of the peptide hormones glucagon and glucagon-like peptide (GLP-1), along with numerous analogues of these key biomolecules. Working separately from MGH’s powerhouse endocrine research laboratory, Mojsov developed a tool-kit of reliable assays that were indispensable to later in vitro and in vivo collaborative studies that established profound insulinotropic effects of this peptide family, findings that were subsequently harnessed clinically with blockbuster drugs directed at often-serious endocrine conditions such as type 2 diabetes and obesity. Significantly, Mojsov was the first to recognize the critical cleavage sites in preproglucagon that give rise to the biologically active species, a truncated form known as GLP-1 (7–37), and carried out the key experiments that proved her hypothesis. Despite being the first author on critical formative publications for the field, and being acknowledged, along with Joel Habener, as one of two co-inventors on the controlling United States patents, Mojsov’s foundational contributions were initially overlooked when GLP-1 biochemistry began to be the subject of major scientific prizes. Fortunately, Mojsov’s work has, within the past year, been better appreciated and deservedly lauded.
Keywords
Glucagon-like peptide 1 (GLP-1), glucagon, type 2 diabetes, obesity, solid-phase peptide synthesis (SPPS), scientific discovery, credit and recognitionIntroduction
Since the isolation and clinical development of insulin in the 1920s, which turned diabetes mellitus from a devastating fatal illness to a manageable chronic condition, peptide hormones that modulate blood sugar levels have received considerable attention in biochemistry and medicine. Originally purified from animal sources, biomaterials such as insulin and glucagon were later synthesized chemically or produced with recombinant DNA methods, opening important new avenues for their investigation and application.
Starting in the 1980s, a class of appetite-connected molecules termed glucagon-like peptide (GLP-1) antagonists emerged as topics of interest, due to their implications for treating conditions such as type 2 diabetes and obesity. The pioneering work in the GLP-1 field was carried out in parallel in the United States and Denmark, the historical centers (along with Canada) of insulin pharmacology, and eventually spawned blockbuster drugs like Victoza, Ozempic, Wegovy, Rybelsus, Trulicity, Mounjaro, and Zepbound that have dominated recent public conversations in science, medicine, and popular culture [1, 2].1
In the historical context of the discovery and application of pharmacologically-relevant peptide hormones [3–5], the developing peptide synthetic chemistry during two critical decades (1970s and 1980s) [6–9], and the sociology of women’s under-recognition in science [10–13], this essay discusses the scientific career of biochemist Svetlana Mojsov (Figure 1), whose foundational findings—both conceptual and experimental—at the dawn of the GLP-1 era set the stage for everything that came thereafter, and have recently received overdue public recognition [14–17].2
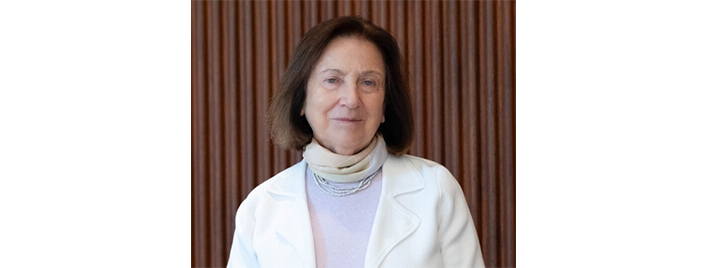
Svetlana Mojsov (September 2024). Credit: Lori Chertoff for The Rockefeller University. Used with permission from all relevant parties
Glucagon work at The Rockefeller University, 1975–1983
Svetlana Mojsov was born in Skopje, Yugoslavia (now Northern Macedonia) and majored in physical chemistry at the University of Belgrade. She arrived in 1972 for graduate studies at the prestigious and unconventional The Rockefeller University in New York City, a research institute founded in 1901 that began to admit graduate students only in 1955. Converting from institute to PhD-granting university, Rockefeller maintained its European-style system of autonomous laboratories rather than departments. Mojsov was accepted into the biochemistry laboratory of Professor Bruce Merrifield, inventor of solid-phase peptide synthesis (SPPS), for which he would receive a Nobel Prize a dozen years later [18, 19]. Through 1980, Mojsov and this perspective’s lead author shared an office, overlooking New York Hospital and the East River, adjacent to the wet chemistry labs within the 4th-floor Flexner Hall space assigned to Merrifield.
Merrifield conceived of SPPS in 1959 and took four years to demonstrate the synthesis of a simple tetrapeptide, in a sole-authored Journal of the American Chemical Society (JACS) paper [20] that became among the journal’s most-ever cited. This precipitated syntheses of an impressive series of increasingly challenging targets: bradykinin (1964; 9 amino acid residues [21]), insulin (1966; total of 51 residues in an A chain and a B chain linked by two interchain and one intrachain disulfide bridge [22]), and the enzyme ribonuclease A (1969; 124 residues with four disulfides [23, 24]), with such rapid progress deriving in part from SPPS’s amenability to automation [25].
Despite the notable successes of SPPS in the decade before Mojsov joined his laboratory, Merrifield still faced considerable skepticism from senior figures in the peptide field, and was looking for a dramatic example that would demonstrate the power of his method. He accordingly gave his enthusiastic encouragement when Mojsov asked whether she could pursue the 29-amino acid peptide hormone glucagon as a target for her doctoral research. In the glucagon primary structure, 58% of the residues are trifunctional, there are several problematic dipeptidyl sequences, and portions of the molecule as well as the molecule as a whole can be severely prone to aggregation in a range of environments (aqueous solution, mixed solvents, and even on-resin). Separately and in combination, each of these factors presented daunting challenges to successful synthesis.
The total synthesis of glucagon had been achieved in 1968 by a large research group headed by Erich Wünsch in Munich, Germany [26], using painstaking solution methodology to create and then stitch together smaller fragments to obtain the full molecule. The early stages of Mojsov’s work in the Merrifield laboratory overlapped with the announcement of similarly laborious syntheses by teams at the Shanghai Institute of Biochemistry in China, headed by Yucang Du, and at Kyoto University in Japan, headed by Haruaki Yajima (see [27]). It was common knowledge that several top research groups in both academia and industry had previously tried and failed to make glucagon by SPPS. There was a clear need for a method to synthesize glucagon by less-herculean means that could be scaled enough for clinical investigation.
To provide definitive proof of purity, and so to preempt the skepticism SPPS of glucagon might invite, Mojsov set out not just to synthesize but also to crystallize glucagon. After carefully analyzing available methodologies, Mojsov settled on a novel plan that used the very acid-labile Bpoc amino protecting group, side-chain protecting groups that were mostly (but not all) derivatives of tert-butyl alcohol, and a p-alkoxybenzyl ester anchor (Wang resin). Circumventing the then-standard Boc/Bzl protecting group paradigm, these choices made it possible to avoid using the strong acid anhydrous hydrogen fluoride (HF) for final cleavage of the peptide from the solid support. Through skillful and meticulous experimental work, Mojsov obtained and crystallized pure synthetic glucagon [27, 28].
Even before Mojsov completed her thesis work, her preliminary results motivated Merrifield to develop a research program around glucagon chemistry and biology. Mojsov led the preparation and writing of a proposal in response to a new National Institutes of Health (NIH) initiative in diabetes research, with Merrifield serving as Principal Investigator (PI) and Mojsov as co-PI. Funded in 1978, this grant (AM 24039) allowed Mojsov to continue in the Merrifield lab as a Research Associate, and to hire a technician. Merrifield ultimately maintained the grant at The Rockefeller University for nearly 30 years.
Mojsov, for her part, was thinking ahead from glucagon synthesis to structure-function studies. Influenced by Roger Unger’s bihormonal hypothesis of glucose metabolism regulation [29, 30], she had the insight that glucagon antagonists might affect the relevant pharmacology in a clinically useful way. The necessary studies would require robust and reproducible methodologies for creating multiple analogues in quantities sufficient for biological assays. Mojsov quickly recognized that her Bpoc/Wang resin approach, having achieved the seemingly impossible in enabling an SPPS synthesis at all, would not meet these further requirements.
Fortunately, Mojsov was in very good company in the Merrifield laboratory, with colleagues such as Alexander R. Mitchell, James P. Tam, Bruce W. Erickson, Stephen Kent, Martin Engelhard, Richard DiMarchi, and William Heath (not to mention this perspective’s lead author) who were refining the scope of SPPS methodology. Laboratory innovations of particular value towards Mojsov’s goals included a new resin support called PAM, new side-chain protecting groups, and a new “low-high” HF cleavage procedure that, individually or in tandem, overcame identifiable limitations associated with the Boc paradigm and its reliance on HF under standard conditions. Integrating these advances allowed Mojsov to use improved Boc chemistry to synthesize glucagon and several of its analogues in the required yields and purities [27, 31]. Indeed, the initial purities of her synthetic materials were so high that a single pass through a preparative HPLC column was sufficient to isolate them for further application [31].
GLP-1 work at Massachusetts General Hospital (MGH), 1983–1990, and beyond
Svetlana Mojsov joined the Endocrine Unit of Massachusetts General Hospital (MGH) in the Spring of 1983. Mojsov’s position was Member, with her salary initially budgeted from the Endocrine Unit, and she had additional duties as an Instructor in Medicine and an Assistant in Biochemistry. In the Fall of 1983, George Cahill appointed Mojsov as an Associate of the Howard Hughes Medical Institute (HHMI) and as Director of the HHMI/MGH Peptide Synthesis Core Facility. This gave Mojsov an independent position with external funding from HHMI that was sufficient to cover her salary and that of a technician to help with peptide synthesis. With these resources and independence, Mojsov began a synthesis-driven research program that led to essential breakthroughs in the GLP-1 field.
Mojsov’s laboratory was situated within the Endocrine Unit, which would be led by Henry (Hank) Kronenberg from the Fall of 1983 until his retirement more than 35 years later. This unit was housed two stories above the Laboratory of Molecular Endocrinology, also part of the Department of Medicine, and headed by physician-endocrinologist Joel Habener. The proximity and later collaborations between the Endocrine Unit and the Laboratory of Molecular Endocrinology have been a source of significant misconceptions about the relationship between Mojsov’s research and the research conducted by individuals supervised by Habener. Due to his relative institutional prominence, and the success of his group’s collaborations with Mojsov, many people assumed erroneously that Mojsov’s breakthroughs at MGH were under Habener’s direction. This reflects a common pattern in scientific visibility and prestige, where recognition tends to accrue to established (overwhelmingly male) scientists in formal leadership roles at the expense of less-established (frequently female) scientists [10].
For clarity, it should be stated explicitly that Mojsov was never employed by, nor a member of, Habener’s group. Their collaboration began in 1984 after Mojsov had already successfully synthesized several different peptide analogues of GLP-1, both full length and the truncated active form GLP-1 (7–37), and had already raised antibodies to the various peptides. Mojsov had also successfully developed viable radioimmunoassays and a chromatographic method to separate the different GLP-1-related peptides, all prior to involving Habener’s group. Only Mojsov identified the critical amino acid sequences that would prove pivotal to the clinical development of GLP-1 biomedicine. In the early years of this research, Mojsov was the only researcher in a position to synthesize the needed peptides (see [32]).
Habener’s team had, shortly before Mojsov’s arrival at MGH, cloned and sequenced cDNA from fish encoding preproglucagon [33]. This celebrated result was followed in 1983 by the work of Graeme Bell and colleagues at the Chiron Corporation (California), who cloned hamster and human cDNAs encoding preproglucagon [34, 35]. Meanwhile, Gerhard Heinrich, a senior researcher in Habener’s laboratory at MGH, was working to clone the rat preproglucagon cDNA, eventually publishing this work [36] a year after Bell. Remarkably, the DNA from all three mammalian species (rat, hamster, human) encoded the identical GLP-1 sequence, which differed from the fish DNA.
On the basis of the sequences published by Bell, and without knowledge of Heinrich’s rat DNA work that had not yet been published, Svetlana Mojsov identified the critical cleavage site in the preproglucagon polyprotein (Figures 2 and 3). Her deduction depended on the unique understanding she had developed in over a decade of investigating the specific biochemistry of glucagon and its analogues. Mojsov’s conjectured cleavage site, whose chemical significance had eluded researchers taking a predominantly biomedical and genetic perspective on glucagon, releases the biologically active form of the peptide, namely GLP-1 (7–37)—a hypothesis that Mojsov was later able to prove experimentally.
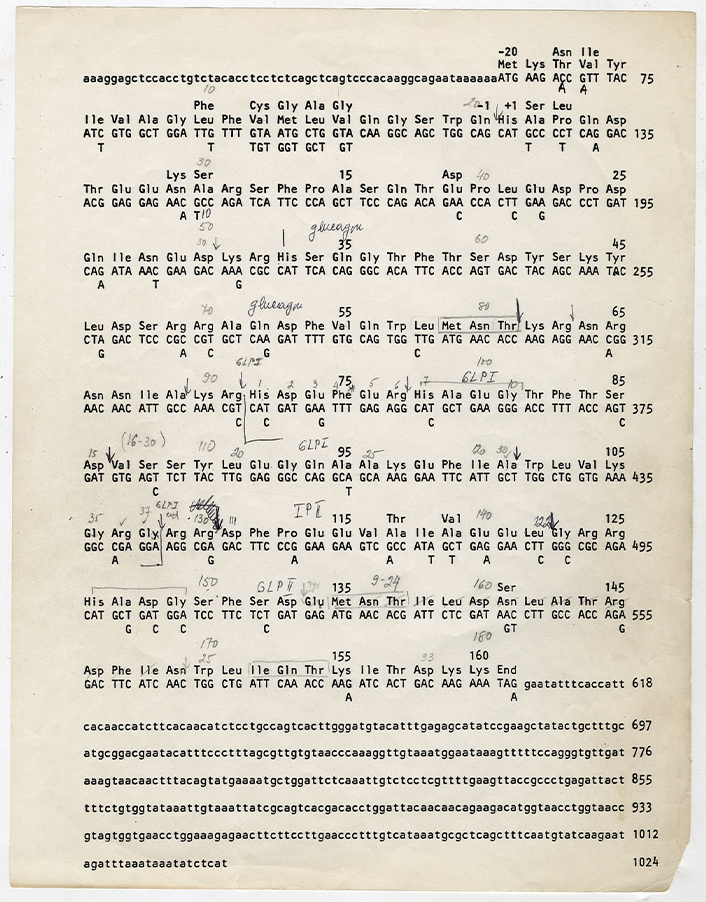
Nucleotide sequence of the cloned human preproglucagon gene, along with the relevant deduced amino acid sequences, as hand-annotated by Mojsov during the early days of her time at MGH. The original sequence data were worked out by Bell and deposited into a database, concurrent with his important Nature article [35]; further computer analysis was carried out with in-house software at MGH. Image courtesy of Svetlana Mojsov, taken from her personal lab notebooks. Used with permission from all relevant parties
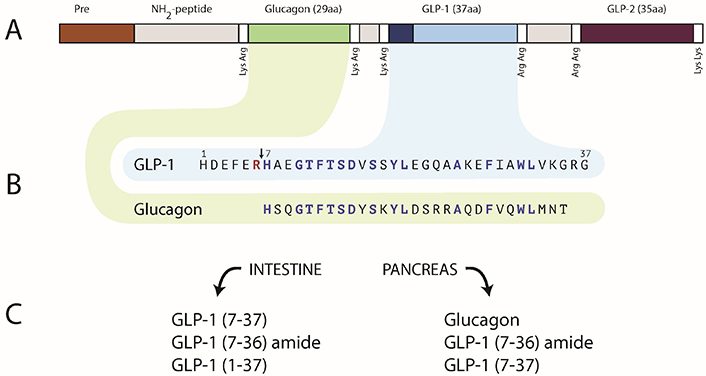
Processing of preproglucagon. (A) Schematic of full polyprotein, showing sites with adjacent basic residues, i.e., Lys and/or Arg. (B) Glucagon (green, 29 residues) and GLP-1 (blue) form after cleavage at the aforementioned sites. Note a critical Arg6–His7 secondary cleavage site in GLP-1, and how it leads to GLP-1 (7–37). (C) Final products of the processing, in the intestine and in the pancreas. Note how the N-terminus of GLP-1 (7–37), now known to be the insulinotropic species due to Mojsov’s research, is analogous to the N-terminus of glucagon and includes residues known to be important for the latter’s function. Artwork by Joseph Alexander (The Rockefeller University). Used with permission from all relevant parties
Working with a technician between Fall 1983 and Winter 1984, Mojsov synthesized the various peptides released from preproglucagon. Then, in Spring 1984, she produced specific antibodies against each peptide, developed a specific radioimmunoassay, and devised an HPLC chromatographic method to detect GLP-1 (7–37) and separate it from all of the other relevant peptides. These newly developed tools were used to prove that GLP-1 (7–37) exists in the gut and, to a small extent, in the pancreas [37]. Having become aware of relevant work being conducted in parallel by Gerhard Heinrich and Ira Wilson in Habener’s group, Mojsov generously invited them to join as authors on the landmark paper that she mostly wrote and that was based principally on her experiments (of eleven figures in [37], all but figure 10 come from Mojsov’s hands-on research).
Mojsov’s outreach to Habener’s group also brought her into contact with Daniel J. Drucker, a physician who joined the Habener laboratory as a postdoctoral fellow in the Summer of 1984. Drucker’s initial project was to artificially transfect cell lines in vitro to learn whether different GLP peptides, i.e., GLP-1 and GLP-2, could be released from preproglucagon. Lacking laboratory experience (see [15]) and so lacking tools to assay which peptides could actually be produced, Drucker collaborated with Mojsov for the difficult and intricate work of making these chemical determinations. Mojsov’s position as second author in [38] reflects the relative status of her and Habener’s laboratories in MGH’s research hierarchy, Mojsov’s deference to Drucker’s earlier career stage and instrumental need for first-author publications (see [39]), and also perhaps the relative value placed on biological versus chemical methods in their clinically-oriented setting.
The next scientific breakthrough in the GLP-1 field came about after Habener brokered a collaboration between Mojsov and Gordon Weir, a physician and renowned diabetologist at the Joslin Diabetes Center in Boston, Massachusetts, that (like MGH) is associated with Harvard Medical School. With all the needed peptide standards in hand, Mojsov, Weir, and Habener convincingly proved that the insulinotropic form of the molecule is indeed GLP-1 (7–37) [40]. To ensure the success of the difficult biochemical methods involved, Mojsov worked side-by-side with Weir’s technician in Weir’s laboratory to carry out all experimental work for the study.
Mojsov’s collaboration with the Habener laboratory continued in a Proceedings of the National Academy of Sciences paper on which Drucker was the first author [41]. Using peptides synthesized by Mojsov, this paper described an in vitro study of gene expression in a cell line, and reported that micromolar concentrations of GLP-1 (1–37) and GLP-1 (7–37) have similar biological effects.3
During the period of Mojsov’s extraordinary findings at MGH, a group at the University of Copenhagen in Denmark directed by physician-physiologist Jens Juul Holst was also studying GLP-1 and related compounds and their physiological effects. Though Holst himself has tended to omit the MGH in reviews of this formative period for GLP-1 science (e.g., [42]), his laboratory’s work has typically been linked with work from the MGH in reviews and recognitions in this area. A few months after the MGH paper [37] appeared, Holst’s group reported on the tissue specific release of full-length GLP-1 and GLP-2 in pigs (not recognizing the truncated active species) [43]. In a follow-up publication the next year, the Copenhagen group evaluated their isolated GLP-1 (7–36), along with synthetic GLP-1 (7–36) amide4 from a commercial source, and reported modest (two to four-fold) increases in insulin production in the perfused pig pancreas when exposed to nanomolar concentrations of these peptides [45], a significant but less dramatic effect than the earlier MGH findings in rats [40].
For her part, Mojsov’s success in driving major research findings with the relatively limited resources of the HHMI Peptide Synthesis Core Facility funding convinced the NIH to award her an independent 5-year grant (DK 40083) beginning in 1988. This set the stage for Mojsov’s return in 1990 to The Rockefeller University at the rank of Assistant Professor. There, she associated herself with the laboratory of Ralph Steinman, who would later be awarded a share of the 2011 Nobel Prize in Physiology or Medicine.5 Mojsov continued to carry out her independent work in GLP-1 biology, supported by the NIH, while also forging new collaborations with other Steinman group members in dendritic cell biology. She was promoted to her present rank of Research Associate Professor in 2002.
Mojsov was already at Rockefeller when the first clinical paper reporting the beneficial effects of GLP-1 (7–37) on patients with diabetes was published by a team from MGH, including Mojsov as a co-author under her former affiliation [47]. This study capitalized on the results of Mojsov’s collaboration with Weir, and used peptide materials that she had personally synthesized.
Credit and recognition for GLP-1 science
Without informing Svetlana Mojsov, MGH filed two patents in June 1990 for protection of GLP-1 intellectual property, listing only Joel Habener as the inventor [48, 49]. The patents were granted two years later, and it took Mojsov a dozen years of determined legal effort after that to reassert herself as a rightful and equal co-inventor, reflected in certificates of correction issued by the United States Patent and Trade Office (USPTO). The story repeated with a 1997 patent, filed in 1993 by MGH on Habener’s behalf, based on Mojsov’s GLP-1 (7–36) amide findings, corrected in 2004 by the USPTO [50], and once more with a patent filed in 2000, granted in 2005, and corrected in 2006 [51].
The GLP-1 patents that were eventually licensed by MGH to Novo Nordisk resulted in several therapeutic agents, first for type 2 diabetes and later for weight loss. Their trade names are Victoza, Ozempic, and (more recently) Rybelsus. Victoza, under the trade name of Saxenda, and Ozempic, under the trade name of Wegovy, are now used for weight loss (Eli Lilly has their own suite of GLP-1 based blockbuster drugs, under the trade names Trulicity, Mounjaro and Zepbound). Once the USPTO issued their correction, Mojsov was able to get some royalties from sales of Victoza, but only from 2010 through the 2012 expiration of the original GLP-1 patents.
With the recent commercial success of therapies based on GLP-1, and the corresponding awareness this has created of the underlying scientific breakthroughs of the 1980s, major prize committees have begun allocating recognition for the foundational work in this field. Among the first major prizes for GLP-1 biomedicine was the 2017 Harrington Award Prize for Innovation in Medicine, which went to Habener, Drucker, and Holst. A jointly written review explaining the honored work included the above-discussed misconception that Mosjov worked “in the Habener laboratory” [52].
The 2021 Canada Gairdner International Prize, covering biomedical/global health research, likewise went to Habener, Drucker, and Holst. The journal Cell commissioned an article explaining the awarded work, which was later corrected after Mojsov alerted Cell’s editors to errors that downplayed her role [53]. The 2023 Wolf Prize in Medicine, sponsored by the state of Israel, went only to Drucker, citing a career of work on endocrine hormones.
The growing sense of momentum for major prizes related to GLP-1 science and medicine lent urgency to efforts to correct the record of these innovations’ origins. A September 2023 profile in Science [15], followed in short order by stories in STAT [16, 17], identified Mojsov to a wide scientific readership as someone whose foundational contributions were being overlooked. A 2023 Nature report at the beginning of the year, extolling ‘breakthrough’ obesity drugs, focused just on Habener and Drucker; within a week, it was corrected to include Holst’s contributions, and a second clarification more than 8 months later (shortly after the Science article came on-line) finally acknowledged Mojsov [54]. By the end of the year, Nature had not only named Mojsov to its annual list of the top ten scientific contributors of 2023, but also published a profile centering her pivotal role in the discovery of GLP-1 [55].
These developments in reporting had a dramatic impact on the major prizes that followed. In May of 2023, for example, nominations closed for the VinFuture Prize, recently launched by the state of Vietnam. The selection committee convened in September on the heels of the Science reporting on Mojsov’s missing credit, and decided to include Mojsov alongside the trio of Habener, Drucker, and Holst that had been proposed in May [56, 57].6
April 2024 brought both the ceremony on The Rockefeller University campus anointing Mojsov as the recipient of the Pearl Meister Greengard Prize, given annually to an outstanding woman scientist [58], and Mojsov’s selection (together with Habener and Drucker) to the TIME magazine list of the Top 100 Influential People of 2024 (Pioneers section) [59, 60].7 MGH subsequently announced that its 2025 Warren Triennial Prize would be given to Habener, Drucker, Holst, and Mojsov [61]. The top scientific honor in Spain, the 2024 Princess of Asturias Award [62], will be presented in mid-October to Drucker, Jeffrey Friedman (The Rockefeller University, discoverer of the satiety hormone leptin), Habener, Holst, and Mojsov.
The two most recently announced awards, promulgated just as this article was submitted in September 2024, are perhaps the most significant because they are statutorily limited to three laureates, in deliberate reference to the Nobel Prize criteria. First, the Tang Prize in Biopharmaceutical Sciences, sponsored by Taiwan, is going to Habener, Mojsov, and Holst [63]. Next, the Lasker Foundation offers a different reading of the case [64], presenting its 2024 Lasker-DeBakey Clinical Medical Award to Mojsov, Habener, and Lotte Bjerre Knudsen (Novo Nordisk, leader of the translational work that turned GLP-1 into clinically viable products).
Timed to coincide with the Lasker Award announcement, Mojsov summarized the significance of her work in her own words in an invited two-page perspective for the Journal of the American Medical Association (JAMA) entitled “Chemistry Matters—From a Putative Peptide to Effective Treatments for Diabetes and Obesity” [65].
Conclusions
This perspective joins a burgeoning literature recognizing the importance of Svetlana Mojsov’s foundational contributions to the understanding and application of GLP-1 peptides in basic science and clinical medicine. The fact that awareness of Mojsov’s role has materialized so decisively over such a short period of time, after such a long period of relative obscurity, testifies to the striking significance of her work and to the strength of evidence of her contributions. It also testifies to the difficulty of attributing individual credit for specific findings in the accretive and collaborative settings of science, and to the continued relevance of barriers—especially those historically facing women scientists—to recognition in a highly inequitable world of research.
Some themes stand out in Mojsov’s story. One is the importance of collaboration and generosity in her approach to science. Even when this cost her potential glory, Mojsov embraced opportunities to team up with other researchers and to join her unique expertise and skills to theirs. The result was collaborative science that more fully realized the potential of Mojsov’s own work. The collaborative culture of the Merrifield laboratory supported Mojsov’s success in synthesis where many had failed, and the multifaceted biomedical research environment at MGH brought out the world-changing implications of the chemical techniques and insights that Mojsov brought from New York.
Another theme is the value of autonomy and its sometimes misleading relationship to credit in science. Creative scientific research benefits from a degree of independence—to forge new ideas—as well as a certain self-effacement to put those ideas ahead of one’s personal distinction and to link them with the ideas of others. Institutional mechanisms of funding, careers, and credit, by contrast, reflect the interdependence of scientists (including dependence on colleagues’ biases and prejudices) and the advantages of certain forms of selfishness and self-promotion. Mojsov’s scientific triumphs reflect a career built around the former values of creative science. She cultivated timely support to maintain her career, but did so in a way that put her independence and potential for collaboration ahead of spotlight-seeking or positions of institutional authority, such as leading a large research group. The benefits of working in the dynamic environments of Rockefeller and MGH, in particular, doubtless came with the costs of working there as a woman who prioritized creative research over self-advocacy, and who faced engrained prejudices that may have undermined advancement to higher-profile institutional roles.
Finally, Mojsov’s research and career trajectory from Rockefeller to MGH and back speaks to the powerful dynamic between basic biochemistry and clinically-oriented biomedical research. This perspective has made clear the pivotal importance of Mojsov’s understanding of chemical synthesis for the decisive breakthroughs in GLP-1 science that made way for their current medical applications. Her experience and capability producing difficult peptides made her indispensable in the research ecosystems where GLP-1 science flourished. At the same time, the clinical orientation of MGH’s research communities appears to have diminished the recognition of Mojsov’s essential contributions during the period she was there. Medical researchers have perhaps sometimes taken peptides (and other biomaterials) for granted, neglecting the value of what skilled peptide scientists bring to the table—including far more than just the peptides they synthesize.
The mismatch between doing good science and recognizing good science runs deep in the history of GLP-1 research, as well as many other fields. Mojsov’s belated appreciation in the wider scientific community and beyond speaks to the value that many place on getting the recognition right, so as to encourage the very best of science and scientists.
Abbreviations
GLP: | glucagon-like peptide |
HF: | hydrogen fluoride |
HHMI: | Howard Hughes Medical Institute |
MGH: | Massachusetts General Hospital |
NIH: | National Institutes of Health |
PI: | Principal Investigator |
SPPS: | solid-phase peptide synthesis |
USPTO: | United States Patent and Trade Office |
Footnote
1 E.g., Jimmy Kimmel’s opening monologue in 2023 from the 95th annual Academy Awards ceremonies, (https://www.youtube.com/watch?v=GoM-64EkchU, at 2:27; refer to https://www.tiktok.com/@mariawatcheseverything/video/7210154537942928682, 19-second loop); https://youtu.be/oV8nd03p3Dk?si=q_NTL115ej9HT1aG (by rapper Ari Dayan); and People Magazine’s year-end cover story about media celebrity Oprah Winfrey, People (December 25, 2023): Oprah Winfrey reveals she uses weight-loss medication as a ‘maintenance tool’: ‘I’m absolutely done with the shaming’ (exclusive) (https://people.com/oprah-winfrey-reveals-weight-loss-medication-exclusive-8414552 and https://youtu.be/5GBthHX8XMs?si=ZrpmCqqWL2llEtr5).
2 This invited perspective article has been part of the recent turns of events, with earlier drafts having circulated since September 2023 to colleagues as well as to journalists investigating Mojsov’s role in the discovery of GLP-1. The rapidly turning tide in Mojsov’s recognition has necessitated significant revisions to the manuscript.
3 It remains an open question of how relevant an in vitro model is to what happens in vivo, where production of insulin is observed at six orders of magnitude lower concentrations (i.e., picomolar level), and where only the truncated GLP-1 is active (see [40], the Mojsov-Weir collaboration, the conclusions of which were subsequently corroborated in numerous research laboratories).
4 The MGH and Copenhagen researchers did not quite agree on the chemical structure of the active species: GLP-1 (7–37) with a carboxyl terminus versus GLP-1 (7–36) with an amide terminus, but this discrepancy was reconciled over the next few years by the discovery of further in vivo processing [44].
5 Steinman was cited for “his discovery of the dendritic cell and its role in adaptive immunity.” In a poignant coda, Steinman succumbed to a 4-year battle with pancreatic cancer, that he had tried to treat with his own discoveries, just before the announcement from Stockholm could reach him. Steinman’s protégé Michel Nussenzweig, now a full Professor at The Rockefeller University and a Howard Hughes Investigator (and since 1980, the husband of Svetlana Mojsov) had the honor of delivering the traditional Nobel lecture about this research [46].
6 It would not be surprising to see a repeat of the VinFuture scenario, where prize committees take the initiative to expand the list of laureates out of awareness of past oversights.
7 A nationally televised primetime presentation of TIME’s honorees caught glimpses of Mojsov seated next to entrepreneur Brittany Mahomes, Super Bowl winning quarterback Patrick Mahomes, and rapper 21 Savage.
Declarations
Acknowledgments
We thank Barbara Barany, Francis Barany, Richard DiMarchi, Jed Fisher, Robert Hammer, Svetlana Mojsov, Michel Nussenzweig, Marjorie Russel, Jeff Seeman, and Gary Taubes for illuminating discussions and background information. We would also like to acknowledge the journalistic work of Elaine Chen, Jennifer Couzin-Frankel, Elie Dolgin, and Megan Molteni that contributed to Mojsov’s belated public and scientific recognition. Meghan Lafferty and Chuck Tomlinson provided library services and administrative support, respectively.
Author contributions
GB: Conceptualization, Investigation, Writing—original draft, Writing—review & editing. MJB: Writing—review & editing. Both authors read and approved the submitted version.
Conflicts of interest
Both authors declare that they have no conflicts of interest.
Ethical approval
Not applicable.
Consent to participate
Not applicable.
Consent to publication
Not applicable.
Availability of data and materials
Not applicable.
Funding
Not applicable.
Copyright
© The Author(s) 2024.