Abstract
Throughout history, biopharmaceuticals have been essential in addressing health crises and enhancing human well-being. With advancements in genetic engineering, biotechnology, and bioinformatics, the development and approval rates of new biopharmaceuticals have surged, placing them at the forefront of the pharmaceutical field. Modern manufacturing processes and streamlined regulatory pathways have further expedited approval timelines, providing hope for previously untreatable conditions and significantly improving patients’ quality of life worldwide. However, despite these advancements, ambiguity persists regarding the definition of biopharmaceuticals due to the use of varied terms and a lack of standardized definitions. This article addresses this confusion by proposing a clear and comprehensive definition of biopharmaceuticals. Additionally, we present a reclassification of biopharmaceuticals into seven distinct verticals based on their functions. We assert that biopharmaceuticals not only offer superior therapeutic benefits for human health but also contribute to environmental sustainability, promoting a healthier planet. Thus, this article aims to clarify the scope of biopharmaceuticals and advocate for their broader adoption in the pursuit of global health and environmental integrity.
Keywords
Biopharmaceuticals, classification, chempharmaceuticals, environmental sustainabilityIntroduction
Biopharmaceuticals have been widely known for many years, with numerous medications being used daily and many products now being developed. Nevertheless, there is still significant uncertainty over the exact definition and extent of the phrase, as there is ambiguity regarding what exactly comes under the biopharmaceutical category. Some often-used words for biopharmaceuticals are biologicals, biologics, biological products, and biologic medicines, which can be confusing. Interestingly, despite their importance, there is no universally accepted definition of biopharmaceuticals [1]. While some definitions are focused exclusively on business models, perceptions, and public relations, others are based on the biological origin and nature of the medicines and their manufacturing procedures [2]. The term “biopharmaceuticals” has traditionally referred to medicinal products that are obtained from biological sources such as tissues, cells, and whole organisms [3]. The world acknowledges the United States Food and Drug Administration (US-FDA) framework as one of the standard systems for ensuring the safety and efficacy of biopharmaceuticals. However, the US-FDA does not give a straightforward definition of biopharmaceuticals. It acknowledges biopharmaceuticals or biologics as “complex mixtures that are not easily identified or characterized”. Moreover, the official term “biologics” refers to viruses, serums, vaccines, blood components, and allergenic items manufactured using live organisms or derived functional components for preventive, therapeutic, and in vivo diagnostic purposes [4]. While this definition clarifies their nature and origin, it falls short of describing the larger spectrum of products that are currently being used, such as recombinant therapeutic proteins, gene therapy, and engineered cells. Moreover, biologics is not an ideal term for common use because of its association with long-forgotten terminology and inconsistent application by the US-FDA [5].
Although most biopharmaceuticals are classified and regulated by the US-FDA as biologics, some are regulated as drugs or medical devices with different laws, definitions, and regulations. Some proteins, such as insulin and somatropin, are regulated as drugs by the Centre for Drug Evaluation and Research (CDER), which raises concerns about the undefined terminology [6]. According to the Public Health Service Act (42 U.S.C. § 262), biopharmaceuticals are referred to as “biological products” and defined as “a virus, therapeutic serum, toxin, antitoxin, vaccine, blood, blood component or derivative, allergenic product, protein, or analogous product, or arsphenamine or derivative of arsphenamine (or any other trivalent organic arsenic compound), applicable to the prevention, treatment, or cure of a disease or condition of human beings” [6]. Meanwhile, the European Union defines “biological products” as pharmacological compounds manufactured from proteins or nucleic acids, utilized for therapeutic or diagnostic purposes that are produced using biotechnological processes [5]. The phrase “biological medical products” applies to all biopharmaceuticals, including genetically modified and antibody therapeutics. As with “biologics”, these phrases are best used solely in their unique regulatory context [7]. Since there are so many definitions for biopharmaceuticals, Rasmussen et al. [1] defined it as “complex molecules derived from a biological source, with the purpose to diagnose, prevent, treat, or cure diseases or conditions of human beings”. However, this definition excludes several biopharmaceutical products, such as (synthetic) peptides and nucleic acid therapeutics. Undoubtedly, differences in perspective will persist regardless of how many definitions are proposed. Therefore, considering all the existing definitions and concepts, biopharmaceuticals can be defined as “any product derived from biological sources or designed to mimic biological molecules for therapeutic or diagnostic purposes”.
Distinguishing biopharmaceuticals from chempharmaceuticals
Biopharmaceuticals are large, complex molecules produced biologically from living cells (e.g., proteins, antibodies, etc.), whereas chempharmaceuticals are small, relatively simple, chemically synthesized molecules with well-defined structures that can be easily represented by chemical diagrams [5]. Chempharmaceuticals are manufactured with high consistency using standardized chemical processes, typically involving conditions and materials that eliminate most organisms and deactivate biological molecules. Biopharmaceuticals, on the other hand, have molecular masses two or three orders of magnitude greater than chempharmaceuticals and involve higher levels of structural complexity, forming polymeric chains with diverse structures and chemical modifications [8]. Unlike chempharmaceuticals, which offer relative simplicity and certainty, biopharmaceuticals necessitate an explanation of their biological source and processing in order to gain a comprehensive understanding. In contrast, the sources and manufacturing processes of chempharmaceuticals are less complex compared to the synthesis of biopharmaceuticals. Furthermore, biopharmaceuticals are typically administered systemically, such as through injection or infusion, rather than orally. This is due to their susceptibility to denaturation in the acidic environment of the gastrointestinal tract and their large size, which can hinder membrane transit and digestion. Additionally, differences between biopharmaceuticals and chempharmaceuticals extend to intellectual property, especially in terms of patents. Biopharmaceuticals generally rely on patents related to biological entities and information, such as vectors, genetic constructs, etc. In contrast, drugs are predominantly protected by patents covering chemical structures [9]. Moreover, biopharmaceuticals often have additional patent protection based on their expression system, process, and formulation. Both quality control measures and product specifications differ significantly. Unlike chempharmaceuticals, biopharmaceuticals generally necessitate manufacturing under sterile conditions, and the final products must be devoid of biological contaminants (or have acceptable levels), such as bacteria, viruses, prions, host or source DNA/RNA, proteins, and cellular debris [10].
Environmental sustainability
By the 1990s, it was increasingly recognized that pharmaceuticals were contaminating the environment and causing harmful effects on exposed organisms and ecosystems. This awareness led to the emergence of the term “pharmaceutical pollution”, which has since become a significant environmental issue associated with chempharmaceuticals [11]. The issue of pharmaceutical contaminants (PCs) arises from the production stage and persists even after the consumption of chempharmaceuticals. During the production of chempharmaceuticals, a significant portion of the reactants are converted into harmful residues and byproducts, which are often released into the environment, leading to ecological damage. In contrast, biopharmaceuticals are developed using biological systems, which enhances their biodegradability and reduces their environmental impact. As a result, biopharmaceuticals represent an eco-friendlier option, reflecting the global movement towards more sustainable healthcare practices [1, 12]. Moreover, a higher percentage of chemical pharmaceuticals (up to 90%) are excreted from the body unmetabolized and are subsequently released into the environment through sewage treatment facilities. These compounds, along with their toxic metabolites and degradation products, accumulate in soil and water, posing significant risks to ecosystems and disrupting biological systems across the planet [13]. The primary reason for the environmental harm caused by chempharmaceuticals is due to inadequate regulatory frameworks that fail to address pharmaceutical residues in water systems, resulting in ecological disruption. Regulatory gaps allow for the persistence of active pharmaceutical ingredients (APIs) in the environment, leading to bioaccumulation, antimicrobial resistance, and adverse effects on aquatic life [11, 14]. Given that the global consumption of therapeutics is rising dramatically due to the annual emergence of new diseases, biopharmaceuticals are said to have a minimally damaging environmental impact, or perhaps put another way, they represent a relatively better option for the health of our planet [1, 15].
Classification of biopharmaceutical
Numerous classification schemes have been developed for biopharmaceuticals, considering a wide range of criteria. Uncertainty still exists since biopharmaceuticals are classified under so many various categories. Therefore, for better understanding, we are categorizing the biopharmaceuticals, based on their biological characteristics into seven verticals (Figure 1).
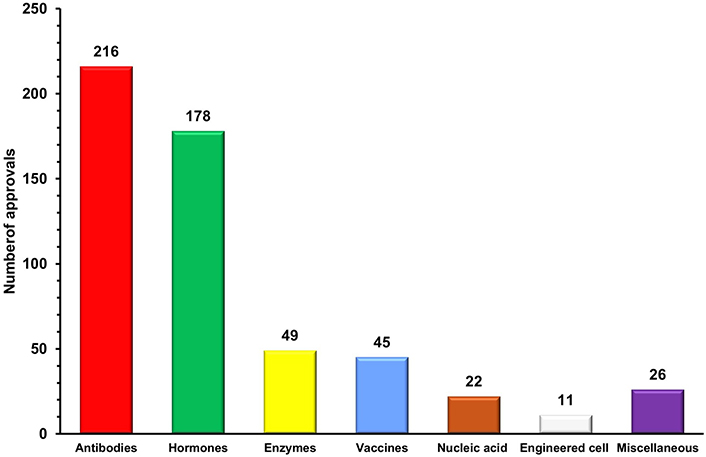
Antibodies
Antibodies are specialized protein molecules that bind with specific antigens and neutralize/eliminate them [16]. Since the first monoclonal antibody muromonab-CD3 was approved in 1986 to treat steroid-resistant acute allograft rejection in kidney transplant recipients, this vertical has undergone remarkable growth [17]. More than 200 therapeutic antibodies or antibody-based biopharmaceuticals have been approved and actively marketed as of 2023 for treatment in immunomodulatory, cancer, and other diverse disorders [18]. This vertical is experiencing significant growth, with 16 therapies receiving initial approval in 2023 and marketing applications for 26 product candidates currently under review in at least one country or region. Additionally, 23 investigational antibody therapies are projected to enter regulatory review by the end of 2024, based on company reports [19]. The approved candidates are primarily dominated by conventional antibodies, including full-length antibodies and fragments such as F(ab')2, fragment antigen-binding (Fab), single-chain variable fragment (scFv), and variable heavy domain of heavy chains (VHH). These are monospecific, first-generation antibodies that have long dominated the biopharmaceutical landscape [20]. Advances in protein engineering have given rise to next-generation polybodies, which are polyspecific and polyvalent antibodies capable of targeting multiple antigens simultaneously. With 11 bispecific antibodies already approved by the US-FDA, this category is expected to lead the antibody sector in the coming decades [19, 20]. Additionally, antibody-drug conjugates (ADCs) are contributing to the growth of this sector, evidenced by the approval of 13 ADCs and over 100 ADCs currently in various stages of clinical trials [21, 22]. The growing demand for targeted therapies and the increasing prevalence of chronic diseases worldwide have accelerated the adoption of antibodies as a preferred and effective treatment option. Advances in antibody engineering have further enhanced the commercial value of antibodies by enabling the development of next-generation monoclonal antibodies (mAbs) with improved therapeutic efficacy, reduced immunogenicity, and greater specificity [18]. This is reflected in the global mAb market, which reached United States dollar (USD) 234.37 billion in 2023 and is expected to double in the coming years [23, 24]. The importance of this sector was also highlighted during the COVID-19 pandemic, where mAbs played a significant role as a treatment option alongside vaccines in managing the disease and its complications. Notable examples include the FDA’s emergency approval of monoclonal anti-SARS-CoV-2 antibodies, such as sotrovimab in May 2021 and bebtelovimab in February 2022, for the treatment of mild to moderate COVID-19 [25]. With numerous candidates in the pipeline, it is likely that the number of antibody approvals will exceed current rates in the years ahead. As a result, antibody-based therapeutics are poised to dominate the biopharmaceutical market due to their increasing approvals, growing demand, and rising revenues.
Recombinant hormones
Recombinant hormones are protein molecules that interact with specific receptors and transduce signals to trigger targeted biological responses in a wide range of physiological processes. The approval of the first recombinant hormone therapeutic insulin in 1923 for the treatment of diabetes, paved the way for the broader field of recombinant hormone therapeutics [26]. Offering essential therapeutic options for a wide range of hormonal illnesses and conditions, the global market for recombinant hormone therapeutics has a substantial impact on the healthcare sector. Thus, this vertical currently ranks second in the biopharmaceuticals sector with over 170 approved therapeutics [18]. In addition, the growing global incidence of growth hormone disorders and menopausal disorders has led to the increasing popularity of hormone replacement therapy (HRT). Treating endocrine disorders by substituting missing or insufficient hormones has been made possible by HRT [27, 28]. Furthermore, children with growth hormone insufficiency can attain normal development growth with the help of recombinant growth hormone treatment, which opens the door to better health and confidence [29]. In the current scenario, recombinant hormones also hold the potential to treat reproductive problems, offering hope to infertile couples [30]. Moreover, it is reported that the geriatric population will be increased in the upcoming years which will increase the prevalence of hormonal imbalance conditions. The rising burden of diabetes and advancements in recombinant hormonal therapies are expected to drive growth in this vertical in the coming years. Additionally, ongoing research on the safety profile of recombinant hormones is expanding the use of these products, further contributing to the vertical’s growth. As a result, the recombinant hormone market, valued at USD 110 billion in 2024, is projected to grow significantly, reaching USD 208.83 billion by 2031 [31, 32].
Enzymes
Enzymes are highly specific protein molecules that catalyze the conversion of substrates into products, playing a crucial role in driving the biochemical reactions essential for life [33]. Enzymes were originally derived from natural sources; however, the discovery of recombinant DNA technology and protein engineering has replaced this process, ushering in an entirely new age in enzyme synthesis [34, 35]. Enzymes are being used to treat a variety of disorders, including genetic conditions, cardiovascular disorders, and cancer [36]. Since the approval of the plasminogen activator alteplase in 1987 for the treatment of acute ischemic stroke, the enzyme therapeutics vertical has grown to include over 45 approved therapies, positioning it as the third-largest vertical in the biopharmaceutical sector [18, 37]. Despite its slower growth, the enzyme therapeutics sector made notable progress in 2023 with the approval of three enzyme replacement therapies (ERT). Velmanase (Lamzede™) became the first therapy for non-central nervous system manifestations of α-mannosidosis. Pegunigalsidase alfa (Elfabrio™), a pegylated, chemically modified α-galactosidase A, was approved for treating Fabry disease. Additionally, cipaglucosidase alfa-atga (Pombiliti™) was approved as an exogenous source of α-glucosidase for Pompe disease [37]. Additionally, asparaginase (ASNase), an important amino acid-depleting drug, has been used for nearly 50 years in the treatment of acute lymphoblastic leukemia (ALL), highlighting the significant role of this vertical in medicine. Remarkably, ASNase alone accounts for 30% of the oncolytic enzyme market, highlighting its significant impact [38]. Following its advancement, numerous other oncolytic enzymes have been developed, and clinical studies have demonstrated their efficacy in treating various cancers [39]. According to research conducted by Rare Disease International in 2022, 6% of the global population is affected by rare diseases, making it a key driver of growth in enzyme therapeutics. This vertical is also expanding due to ongoing research advancements and the development of next-generation ERT that offer improved efficacy and safety profiles [40]. Although ERTs have been shown to be beneficial in treating rare genetic conditions and enzyme deficiency disorders, their high price is a barrier to growth and demand in this vertical. The high cost of ERTs is a hindrance for many patients with rare genetic conditions, causing inequities in therapy availability. The high cost also placed a significant economic burden on insurers and government-funded healthcare systems. Moreover, gene therapies are emerging as a more promising approach, with the potential to reduce or completely eliminate the requirement for ERT. Even though this vertical is growing at a very slow rate annually, the market was valued at USD 10 billion in 2023 and is expected to grow in the coming years [41].
Vaccines
Vaccines are prophylactic preparations that boost the immune system by modulating its components and training the body to recognize and defend against specific pathogens, thereby providing immunity [42]. This vertical is the fourth leading player in the biopharmaceutical sector, with 45 approved vaccines as of now [18, 42]. Despite the relatively low approval rate, approved immunization vaccines remained the primary drivers of this vertical’s growth. Additionally, the high cost and low success rate of vaccine development make it difficult for manufacturers to raise capital to cover their operational expenses [43]. But the COVID-19 pandemic has shown how vaccines remained the primary strategy of choice to fight infectious diseases and how they will continue to be an essential therapeutic. The World Health Organization (WHO) projects the global distribution of over 16 billion doses of over 40 different vaccinations in 2021, with a total projected value of USD 141 billion. The launch of COVID-19 vaccines was primarily responsible for this significant growth in vaccine market value, which increased 3.5 times when compared to 2019. Additionally, COVID-19 vaccines continue to account for a sizeable portion (60%) of the worldwide vaccine market volume, even with the reduction in COVID-19 vaccine sales in 2022 [44, 45]. The FDA has approved and granted emergency use authorization for updated COVID-19 vaccines (2024–2025 formulation) to enhance protection against COVID-19 caused by currently circulating variants. These new vaccines are designed to offer a more effective defense against evolving strains of the virus [42]. This demonstrates that the demand for vaccines is primarily driven by the rising occurrence of infectious diseases around the world. Furthermore, next-generation vaccine development, including mRNA and recombinant vaccines, provides the potential for market expansion and innovation [46, 47]. Nevertheless, it is projected that the global vaccine market value will increase gradually from USD 74.8 billion in 2023 to USD 85.2 billion by 2028, reflecting the vertical’s expected long-term growth [45].
Nucleic acid therapeutics
Nucleic acid therapeutics, also known as therapeutic nucleic acids (TNAs), are molecules derived from nucleotides that exert their therapeutic effects by regulating specific target genes. This vertical encompasses DNA-based therapeutics (including antisense oligonucleotides, DNA aptamers, and gene therapy), as well as RNA-based therapeutics (such as microRNAs, short interfering RNAs, ribozymes, RNA decoys, and circular RNAs) [48]. Despite being in its early stages, this vertical, which currently comprises 22 approved therapeutics, including 6 RNAse-H-activating antisense oligonucleotides (ASOs), 5 splice-switching ASOs (SSOs), 1 LNP-formulated siRNA, 5 GalNAc-conjugated fully modified small interfering RNAs (siRNAs), 2 aptamers, and 1 clustered regularly interspaced short palindromic repeats (CRISPR)-based therapeutics, is projected to experience significant growth in the coming years [18, 49]. As TNAs were successfully utilized for developing COVID-19 disease medications, demand for them increased during the previous few years. Notably, such as micro RNAs (miRNAs), ASOs, and siRNAs were reported to be very effective in combating the COVID-19 virus. These treatments also provide tremendous development potential since they can inhibit the expression of viral genes during and after transcription [50]. Moreover, approximately 400 RNA-targeting drug development projects have been carried out worldwide, with two-thirds in the pre-investigational new drug application stage, one-third in Phase I or II early clinical trials, approximately three percent in Phase III, and some awaiting regulatory approval for COVID-19 treatment [51]. In 2023, the RNA-based therapeutics dominated the market, capturing the largest revenue share of 65.75% [52].
Gene therapy, a crucial element within this field, was first proposed over fifty years ago [53]. Significant biological and technological advancements since then have resulted in the development of several safe and effective platform technologies [54]. Today, gene therapy is primarily used to treat various genetic disorders, including adenosine deaminase deficiency, α-1-antitrypsin deficiency, cystic fibrosis, familial hypercholesterolemia, Gaucher’s disease, and haemophilia B [51]. Additionally, gene therapy offers treatments for several rare disorders that currently have limited or no available options. This creates new opportunities for innovative therapies targeting specific genetic mutations, which can address debilitating genetic conditions. As a result, gene therapy holds significant potential to provide cures for genetic diseases affecting millions of patients [55]. Thus, the growing prevalence of genetic disorders, increasing healthcare expenditures, and the pharmaceutical industry’s strong interest in and shift toward novel biologics are expected to drive the expansion of this vertical. These positive trends underscore the potential of nucleic acid therapeutics to enhance disease treatment and improve patient outcomes in the coming years. Consequently, the overall market for this vertical is projected to grow from USD 5.59 billion in 2024 to USD 10.90 billion by 2029 [55].
Engineered cell therapeutics
Engineered cell therapeutics employ whole cells as therapeutic agents for treating diseases. Although it is in its earlier stage, this vertical has cleared regulatory obstacles and entered the market with 11 approved treatments, which has increased curiosity and excitement [18, 56]. Currently, engineered cell therapeutics represent a diverse arsenal of therapeutic tools, with distinct capabilities, including precise cellular treatments that can function as live assassins and thereby providing hope for diseases that were previously incurable [57]. Utilization of differentiation capability of pluripotent stem cells for treating illnesses such as Parkinson’s disease [58], spinal cord injuries [59], and heart failure, as well as for rebuilding damaged tissues [60] are some of the exciting potentials of engineered cell therapies. Additionally, advancements in gene editing tools like CRISPR-Cas9 are enabling higher precision of cell engineering, leading to more effective personalized medications [61]. The development of cell banking facilities and the subsequent growth in cell production, storage, and have expanded opportunities and fuelled the growth of this vertical. Additionally, the rising prevalence of chronic diseases, growing interest in personalized medicine, and the increase in clinical trials involving cell-based therapeutics are all anticipated to drive the expansion of this biopharmaceutical vertical [62]. Thus, the global market of this vertical was estimated at USD 4.74 billion in 2023 and is anticipated to grow substantially in upcoming years [63].
Miscellaneous
This vertical encompasses the biopharmaceuticals which are not falling into the six categories mentioned above and includes non-enzymatic clotting factors and small peptides (less than 10 kDa). While most clotting factors are enzymatic, there are approximately 20 approved therapeutics derived from factor VIII that are non-enzymatic in nature [18]. Moreover, the global market for recombinant non-enzymatic coagulation factors is dominated by factor VIII-derived clotting therapeutics. The main causes of this include the rising prevalence of bleeding disorders such as haemophilia A, the growing demand from consumers for recombinant coagulation factors, and the rising quantity of coagulation factor-related R&D [64]. Meanwhile, peptides, which are small chains of amino acids, have the potential to treat many diseases due to their ability to easily cross cell membranes, and currently around 6 peptides have been approved for treating various conditions [18].
Conclusions
Biopharmaceuticals and chempharmaceuticals differ significantly in terms of manufacturing processes, structural composition, mechanisms of action, and regulatory frameworks. For decades, chemical pharmaceuticals have dominated the sector, but they have faced growing criticism due to the adverse effects originate from their synthetic nature. In contrast, biopharmaceuticals offer a promising alternative, leveraging their biological origins for improved efficacy and safety. Their rapid growth and increasing dominance in therapeutic applications have demonstrated their immense potential. Despite the growing prevalence of biopharmaceuticals, there remains confusion regarding what exactly falls under this category. To address this, a unified definition that encompasses the entire scope of biopharmaceuticals, aiming to bring clarity to this evolving field, is proposed. Additionally, a categorization system that organizes biopharmaceuticals into seven distinct verticals based on their biological function is also introduced. This framework not only facilitates a better understanding of the sector but also aims to streamline regulatory processes, reducing confusion among regulatory authorities across different countries. The COVID-19 pandemic has highlighted the value of biopharmaceuticals, drawing significant public interest. Due to the high demand for these therapeutics, the biopharmaceutical market has grown considerably in recent years, outpacing the overall pharmaceutical market. Among the various biopharmaceuticals being developed, antibodies have emerged as the dominant vertical due to their specificity and effectiveness. They have consistently led the biopharmaceutical sector in both the development pipeline and approvals, despite the fact that antibody discovery has been done far later than other verticals. Antibodies, with their wide range of applications, have become a significant source of revenue growth for the biopharmaceutical sector, accounting for more than half of the total revenue. As research progresses deeper into areas like nanobodies, ADCs, and polyspecific antibodies, it is clear that antibodies will continue to be at the forefront of biopharmaceuticals, with a promising future ahead.
Abbreviations
ADCs: | antibody-drug conjugates |
ASNase: | asparaginase |
ASOs: | antisense oligonucleotides |
CRISPR: | clustered regularly interspaced short palindromic repeats |
ERT: | enzyme replacement therapies |
HRT: | hormone replacement therapy |
mAbs: | monoclonal antibodies |
TNAs: | therapeutic nucleic acids |
US-FDA: | United States Food and Drug Administration |
Declarations
Author contributions
AHP: Conceptualization, Writing—original draft, Writing—review & editing. YRP and JA: Writing—original draft. All authors read and approved the submitted version.
Conflicts of interest
The authors declare that they have no conflicts of interest.
Ethical approval
Not applicable.
Consent to participate
Not applicable.
Consent to publication
Not applicable.
Availability of data and materials
Not applicable.
Funding
The authors thank the funding support provided by the Department of Biotechnology (DBT), Government of India, under grant number [BT/PR42965/PBD/26/821/2021]. The funders had no role in study design, data collection and analysis, decision to publish, or preparation of the manuscript.
Copyright
© The Author(s) 2025.
Publisher’s note
Open Exploration maintains a neutral stance on jurisdictional claims in published institutional affiliations and maps. All opinions expressed in this article are the personal views of the author(s) and do not represent the stance of the editorial team or the publisher.