Abstract
Sirtuins are a family of NAD+-dependent class III histone deacetylases that regulate histones and other proteins. The mammalian sirtuins comprise seven members that have a role in energy metabolism, DNA repair, inflammation, cell survival, apoptosis, cellular senescence, oxidative stress, and mitochondrial production. Sirtuin modulation may have beneficial effects on aging and age-related diseases; thus, attracting a growing interest in discovering small molecules modifying their activity. A class of compounds both natural and chemically synthesized has emerged as sirtuin activators. This review discusses mammalian sirtuins in aging, the small molecules that activate sirtuins, modulation of sirtuin activity, and its impact in alleviating the effects of aging.
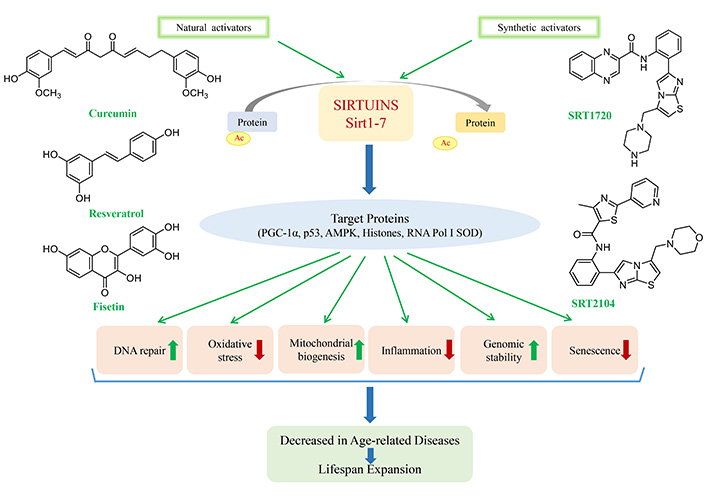
Keywords
Sirtuins, activators, aging, resveratrol, NAD+Introduction
The world population is expected to peak around 2084, rising to 10.3 billion [1]. However, the rate of population aging is faster than it ever was in the past. By the end of this decade, in 2030, one in six individuals will be 60 or older, increasing the population of people aged 60 to 22% by the year 2050 [2]. In the upcoming decades, this will lead to aging being one of the major socio-economic and psychological burdens for individuals and society globally [3]. As an individual’s age progresses, its organ function declines, increasing its susceptibility to many chronic diseases, and putting immense pressure on the monetary power of countries in healthcare and pension. The lifespan of humans has increased rapidly, with the advancement in medical technology and improved living conditions; but it has not led to an increase in the health span. This is the primary reason to study, investigate, and understand the various aspects of aging and devise interventions to potentially benefit individuals, and play a part in reducing the socio-economic burden of countries with high percentages of an aged population.
Aging is a time-dependent and irreversible pathophysiological phenomenon, accompanied by the development of numerous age-related diseases, such as type 2 diabetes, cardiovascular disease, cancer, Alzheimer’s disease, Parkinson’s disease, musculoskeletal problems, etc. [4]. Aging can also be referred to as an inclusive term for a number of molecular, biochemical, physiological, genetic, and epigenetic processes that have been described as hallmarks of aging [5, 6]. Interestingly, these hallmarks of aging are decentralized and interdependent on one another. Over the years, as our understanding of the biochemical, morphological, and functional decline of various processes related to aging has increased, efforts are underway to come up with strategies and interventions to ameliorate the effects of age-related diseases. Dietary interventions such as intermittent fasting, calorie restriction (CR), incorporation of CR mimetics, with physical exercise, etc., have been used in animal models and humans to slow down aging by alleviating the effects of the processes falling under the category of hallmarks of aging. This review focuses on one emerging field of research, i.e., sirtuin activators, a group of small molecules that activate sirtuin proteins, leading to sirtuins being considered therapeutic targets for anti-aging and age-related diseases.
Sirtuins in aging
Sirtuins are a family of nicotine adenine dinucleotide (NAD+)-dependent class III histone deacetylases [7], which catalyze post-translational modification of histones and other proteins and play a prominent role in aging. Sirtuins are dependent on NAD+ for their activity and, thus, are activated during conditions of low energy, like fasting or exercise. In 1999, it was reported that a sirtuin protein could extend the lifespan of Saccharomyces cerevisiae [8]. Sirtuins play a major role in energy metabolism, DNA repair, inflammation, cell survival, apoptosis, cellular senescence [9], oxidative stress, and mitochondrial production [10] and can be modulated by diet and exercise.
In mammals, seven sirtuin family members have been detected, namely Sirt1–Sirt7. Although, the seven members have an identical highly conserved catalytic core domain of 275 amino acids, their localization within the cellular compartments varies, with Sirt1, 2, 6, and 7 localized in the nucleus, Sirt3, 4, and 5 in mitochondria, and Sirt1, 2 in the cytoplasm [11]. In addition to deacetylase, sirtuins also possess different enzymatic activity [12]. Some of the target proteins of sirtuins include NF-κB, phosphoglycerate mutase-1 (PGAM-1), sterol regulatory element-binding protein (SREBP), p53, peroxisome proliferator-activated receptor-γ co-activator 1α (PGC-1α), eNOS, mTORC2, and forkhead box protein O3a (FOXO3a).
Sirt1
Sirt1 is the most widely studied mammalian sirtuin as it is closely related to S. cerevisiae Sir2 and also for its role in aging, inflammation, and metabolic dysfunction. It is localized in the nucleus; however, it translocates to the cytoplasm, in response to certain stimuli [13]. It plays an important role in DNA damage recognition and response, and metabolism via deacetylation of target proteins. It deacetylates histones like H3, H4, and H1, along with modification of more than fifty non-histone proteins, including transcriptional factors p53, p65, PGC-1α, NF-κB, SREBP, and repair proteins. Sirt1, although its overexpression in transgenic mice leads to an increase in lifespan, the longevity aspect of the role of Sirt1 in aging, is implicated by the process of CR [14]. However, overexpression of Sirt1 specifically in the brain of both male and female mice, leads to an increase in lifespan [15]. This suggests that overexpression of Sirt1 in the brain will also have an impact on neurodegenerative diseases like Parkinson’s. For example, it has been observed that overexpression of Sirt1 causes a decline in amyloid plaques in several models of Alzheimer’s disease [16]. Although, most research for Sirt1 has been conducted in non-human model organisms, increased Sirt1 levels were found in muscle tissue of humans participating in CR [17].
Sirt2
Sirt2 is mainly localized in the cytoplasm, but it translocates to the nucleus. It moves to the nucleus during mitosis and plays a major role in the cell cycle. It regulates mitosis by controlling the activity of the anaphase-promoting complex/cyclosome. Sirt2 delays cell cycle progression, deacetylates p300 and tubulin, and also plays a role in reducing inflammation [18, 19]. Sirt2 promotes DNA repair and acts as a tumor suppressor by transcriptionally activating glycosylase OGG1 in an ATM/ATR-dependent manner [20]. It also contributes to the stabilization of p53 in the nucleus by regulating the acetylation state of p53 at lysine 382 and regulating the DNA damage response [21]. The kinetic and crystal structure study of Sirt2 also demonstrates a demyristoylase activity [22]. In kidneys, activated Sirt2 deacetylates FOXO3a during renal ischemia, promoting its nuclear shuffle and activating caspase-8 and caspase-3, thus inducing death [23].
Sirt3
Sirt3 is a mitochondrial sirtuin that promotes the transcription of superoxide dismutase 2 (SOD2) by directly deacetylating and activating SOD2, promoting the transcription of SOD2 and peroxisomes [24]. It induces PGC-1α and FOXO3a upregulation, leading to the restoration of manganese superoxide dismutase (MnSOD) activity and levels [25]. Sirt3 regulates glutamate dehydrogenase (GDH) [26] and acetyl-CoA synthetase 2 (ACS2), whose activity is regulated by reversible acetylation, as deacetylation activates the enzyme [27]. During prolonged fasting, Sirt3 deacetylates long-chain acyl-CoA dehydrogenase (LCAD), a protein involved in fatty acid oxidation, which activates fatty acid oxidation [28]. Sirt3, during CR, contributes to mitochondrial physiology by reprogramming the mitochondrial protein acetylome [29].
Sirt4
Sirt4 is a mitochondrial protein, lacking in in vitro deacetylase activity. In pancreatic beta cells, Sirt4 has been found to oppose the effects of CR, by actively inhibiting GDH [30]. Sirt4 also regulates ATP homeostasis by improving the efficacy of ATP via the adenine nucleotide translocator 2 (ANT2) and maintains retrograde signaling. Sirt4 overexpression is associated with increased ATP levels, and loss of Sirt4 expression leads to decreased cellular ATP levels in vitro and in vivo [31]. Overexpression of Sirt4 protects the organism by reducing the expression of proteins, such as Bax and phosphorylated p38, and upregulated Bcl-2 expression. It causes a decline in inflammatory factors, such as tumor necrosis factor alpha (TNF-α), interleukin (IL)-1β, and IL-6 [32].
Sirt5
Sirt5 is a mitochondrial enzyme, involved in metabolism and stress response, regulated largely by two proteins PGC-1α and AMP-activated protein kinase (AMPK). Overexpression of PGC-1α elevates cellular Sirt5 levels, while activation of AMPK down-regulates Sirt5 levels, playing a role in mitochondrial energy metabolism [33]. Sirt5-deficient mice exhibit defective energy metabolism and a decline in ATP production [34]. Overexpression of Sirt5 enhances AMPK phosphorylation and attenuates mitochondrial dysfunction, by alleviation of mitochondrial structural damage and restoration of ATP molecules [35]. In mice, Sirt5 desuccinylates metabolic enzymes, maintaining energy homeostasis and protecting mitochondrial metabolism [36].
Sirt6
Sirt6 is a nuclear sirtuin, with three catalytic enzyme activities—ADP-ribosyltransferase, deacetylase, and deacylase activity. Mice deficient in Sirt6 protein depict genomic instability and premature aging [37]. Sirt6 maintains the chromatin structure at telomeres by deacetylation of histone H3 lysine 9, preventing telomeric DNA damage and cellular senescence [38]. Sirt6 and Sirt1 act synergistically, where Sirt1 deacetylates Sirt6 on residue K33, and deacetylated Sirt6 remodels the chromatin [39]. CR and nutrient availability have a positive impact on Sirt6, as it is upregulated during CR, resulting in the expression of genes involved in energy metabolism, oxidative stress, autophagy, and inflammation [40]. Sirt6 also deacetylates non-histone proteins in the nucleus and cytoplasm, including members of the FOXO family, p53, and nicotinamide phosphoribosyl transferase (NAMPT) [41]. Genetic overexpression of Sirt6, in mice partially prolongs lifespan by restoring energy homeostasis and blocking insulin-like growth factor-1 (IGF-1)/AKT signaling [42–44]. Sirt6 also represses LINE1 retrotransposons by mono-ADP ribosylating the nuclear corepressor protein KAP1; however, with the increase in age of the mice and DNA damage, Sirt6 levels decrease, and the silenced retroelements are activated [45]. In a study by Pan et al. [46], it was shown that Sirt6 also plays a protective role by reducing oxidative stress.
Sirt7
Sirt7 is a nuclear sirtuin, involved in the regulation of RNA polymerase I (Pol I) transcriptional machinery in mammalian cells [47]. It plays an active role in ribosome biogenesis, metabolic homeostasis, mitochondrial biogenesis, DNA repair, inflammation, and apoptosis [48]. Mice deficient in Sirt7 have a decrease in lifespan and develop inflammatory cardiomyopathy [49, 50]. Sirt7 mediates DNA damage repair by deacetylation of ATM protein and inhibiting it from activation [51]. Overexpression of Sirt7 in hypertensive mice promotes Krüppel-like factor 15/nuclear factor erythroid 2-related factor 2 (Nrf2) signaling, indicating that Sirt7 is a promising target for the treatment of injured kidneys [52]. It has been reported that overexpression of Sirt7 in the hippocampal region in aged mice improves neurogenesis and cognitive functions [53].
The cellular localization, a few examples of target proteins, and their functions are summarized in Table 1.
Summary of cellular localization, target proteins, and functions of sirtuins in aging
Sirtuin | Cellular localization | Molecular weight (kDa) | Enzymatic activity | Target proteins | Function | References |
---|---|---|---|---|---|---|
Sirt1 | NuclearCytoplasmic | 81.7 | DeacetylationDecrotonylationDelactylation | H1, H3, H4, LKB1, AMPK, MnSOD, NF-κB, FOXO, PGC-1α, mTOR | DNA repair, insulin secretion, glucose metabolism, neuroprotection | [7, 54, 55] |
Sirt2 | CytoplasmicNuclear | 43.2 | DeacetylationDemyristoylation | H4K16, α-tubulin, FOXO, p53 | Cell cycle regulation, DNA repair | [22, 56] |
Sirt3 | MitochondrialNuclearCytoplasmic | 43.6 | DeacetylationDecrotonylationDelactylation | H3, H4, FOXO, MnSOD, catalase, p53GDH | ATP production, regulation of mitochondrial metabolism | [26, 27, 54, 55] |
Sirt4 | Mitochondrial | 35.2 | ADP-ribosylationDeacylationDeacetylation | AMPK, Glutamate dehydrogenase malonyl-CoA decarboxylase | Insulin secretion, fatty acid oxidation, apoptosis | [30, 57, 58] |
Sirt5 | Mitochondrial | 33.9 | DeacetylationDemalonyationDesuccinylationDeglutarylation | SOD, Succinate dehydrogenase | Fatty acid oxidation, oxidative stress | [36, 59–61] |
Sirt6 | Nuclear | 39.1 | ADP-ribosylation DemyristoylationDeacetylationDepalmitoylation | H2B, H3, FOXO, PARP1, NF-κB, IGF-1/AKT | DNA repair, genome stability, telomere maintenance, stress response, energy homeostasis | [38, 45, 62, 63] |
Sirt7 | Nucleolar | 44.8 | DeacetylationDesuccinylationADP-ribosylation | H2A, H2B, H3, FOXO3a, RNA polymerase-1, PARP-1, p53, ELK-4 | Regulation of rRNA transcription, stress resistance, DNA repair | [64–66] |
AMPK: AMP-activated protein kinase; MnSOD: manganese superoxide dismutase; FOXO: forkhead box protein O; PGC-1α: peroxisome proliferator-activated receptor-γ co-activator-1α; GDH: glutamate dehydrogenase; SOD: superoxide dismutase; IGF-1: insulin-like growth factor-1
Sirtuin activating compounds
The mammalian sirtuins are involved in several biological processes and overexpression of sirtuins plays an important role in increasing lifespan in model organisms. Sirtuins possess the potential to become markers and therapeutic targets for the management of aging and other age-related diseases. Dietary restriction is the only intervention known to increase lifespan in model organisms [67] and primates to a certain extent. The beneficial effects of CR are mediated through the nutrient sensing proteins, and sirtuins being one of the proteins, its activators can play an enormous role in therapeutics. Other than targeting the core catalytic subunit, sirtuins can be modulated by targeting the N- and C-terminal of the proteins, to enhance their enzymatic activity. With the advancement in research in this field, various small molecules, both natural and chemically synthesized compounds, have emerged as activators of sirtuins that can alleviate the conditions associated with aging and age-related diseases.
Resveratrol
Resveratrol is one of the first compounds discovered to mimic CR and activate sirtuins. Resveratrol (3,5,4ʹ-trihydroxy-trans-stilbene) is a naturally occurring plant polyphenol and a phytoalexin, of the stilbene family. It is found in plants such as grapes, blueberries, apples, plums, peanuts, and grape products such as red wine. It interacts with a wide variety of proteins, including Nrf2 and sirtuins [68]. Resveratrol and related polyphenolic compounds have antioxidative properties, thus possessing health-enhancing effects [69]. In the year 2003, it was reported that resveratrol, as a direct activator of sirtuins, extended the lifespan of S. cerevisiae [70]. Subsequent studies were also performed in other model organisms like Drosophila melanogaster, mice, etc. Resveratrol also modulates Sirt3 and Sirt5’s deacetylase activity [71]. Resveratrol upregulates the expression of antioxidant encoding genes and neuroprotection factors, Sirt1 and Sirt3 in immortalized lymphocytes of Alzheimer’s patients [72]. In the hippocampal area, Sirt1 activation by resveratrol produces neural malleability [73]. In 2020, Ma et al. [74] showed that resveratrol significantly increased Sirt1 expression in a rat model of diabetes with concomitant Alzheimer’s disease. The study also demonstrated increased acetylcholinesterase, malondialdehyde, and reduced SOD and glutathione levels.
A close interaction occurs between the energy sensors, AMPK, and Sirt1 [75]. It regulates AMPK and Sirt1 in a dose-dependent and reciprocal manner. Resveratrol, in low doses, activates Sirt1, leading to deacetylation of liver kinase B1, which acts as an upstream kinase of AMPK, thus activating it. An analysis of gene expression in streptozotocin-induced diabetic rats showed that resveratrol supplementation balanced the hippocampal gene expression, involved in the neuronal origin and synaptic malleability (Hdac4, Hat1, Wnt7a, ApoE) and decline in expression of Jak-Stat pro-inflammatory signaling (IL-15, IL-22) [76]. The effect of resveratrol pretreatment in rats on NLRP3 inflammasome and ROS may also be Sirt1 dependent [77].
However, the role of resveratrol in activating Sirt1 is ambiguous and needs further investigation [66]. In initial studies, activation of Sirt1 by resveratrol is dependent on the presence of a fluorophore, Fluor-de-Lys peptide substrate, as removal of the fluorophore failed to deacetylate the same peptide [78, 79].
Curcumin
Curcumin is a bright yellow compound, derived from the rhizome of the turmeric plant. It is a hydrophobic polyphenol with low bioavailability in humans [80]. Its supplementation in lower concentrations shows anti-aging effects, however, higher concentrations can trigger senescence [81]. It also acts as an activator of Sirt1 and is known to stimulate sirtuins [82, 83]. The down-regulation of Sirt1 after myocardial infarction was attenuated by curcumin pretreatment, which indicated that the activation of Sirt1 might be activated by curcumin [84]. Curcumin pretreatment also protects against a range of neurological disorders like stroke by activation of Sirt1 [85, 86]. Increased level/activity of AMPK and Sirt1 was observed in muscles of mice and rats, post curcumin supplementation [87, 88] enhancing the effect of exercise. Activation of Sirt1 by curcumin blocks the neurotoxicity of amyloid‐β25–35 in rat cortical neurons [85]. In a rat model of chronic obstructive pulmonary disease, curcumin supplementation upregulated Sirt3 expression and attenuated inflammatory markers and oxidative stress [89]. Curcumin reduced oxidative stress and protected the kidneys by activating Sirt1, Sirt3, and Sirt4 in cisplatin-induced toxicity in male rats [90]. The protective role of curcumin in aging and age-related pathologies is mediated by activated sirtuins, along with its anti-inflammatory and antioxidant effects.
Pterostilbene
Pterostilbene is a dimethyl ether resveratrol analogue, safe for human consumption with a high bioavailability [91]. It has been reported to increase lifespan in model organisms due to its anti-oxidative and anti-inflammatory properties [92, 93]. In recent studies, pterostilbene was found to activate sirtuins, AMPK and Nrf2 [94, 95]. In an obesity mice model, pterostilbene enhances thermogenesis and mitochondrial biogenesis by activating the Sirt1/PGC-1α/Sirt3 pathway [96]. It has also been reported that pterostilbene activates the Sirt1-FOXO1/p53 signaling pathway and decreases the apoptotic ratio in skeletal muscle cells induced by ischemia-reperfusion injury [97].
Other natural compounds activating sirtuins
There are some other natural compounds that are known to activate sirtuins. These include flavonols like fisetin, quercetin, apigenin, epigallocatechin gallate (EGCG), etc., along with chalconesbutein and isoliquiritigenin, the stilbene piceatannol. The chemical structures of some of the natural compounds activating sirtuins are shown in Figure 1. These compounds increase the lifespan of yeast by activating sirtuins [98]. Fisetin also activates sirtuin deacetylase activity in Drosophila melanogaster S2 cells, and a 23% extension of the lifespan was observed when the Drosophila melanogaster was fed on a fisetin-rich diet [99]. In mice, honokiol was found to reverse cardiac hypertrophy by activating Sirt3 [100]. Quercetin and its derivatives at high concentrations activate Sirt6 by binding to the Sirt6-selective acyl binding channel [101]. Polyphenols like cyanidin and luteolin also activate Sirt6 [102]. Fisetin and luteolin suppresses oxidative stress by activating sirtuins like Sirt1, Sirt3, and Sirt6 and reducing expression of FOXO3a [103].
Synthetic sirtuin activators
The natural activators of sirtuins like resveratrol, although they gained popularity initially, did not show promising results in clinical trials as they accompanied a significant amount of side effects [104]. This led researchers to explore effective and alternative compounds as sirtuin activators. A number of compounds were established as the first generation of synthetic Sirt1 activators, including SRT1720, SRT2183, and SRT1460, and are derived from the imidazo[1,2-b]thiazole core structure. However, further studies based on these molecules led to a diversion from the original idea [105].
The second generation of synthetic Sirt1 activators was represented by two molecules SRT2104 and SRT3025. Out of the two, SRT2104 was identified via screening of 29,000 compounds. On the basis of its biochemical activity and pharmacokinetic characteristics, SRT2104 was selected as a potential drug candidate. SRT2104 was picked up by GlaxoSmithKline undergoing clinical trials. The results of the trials and its role as a potential agonist is described in the paper reviewed by Chang et al. [106]. The structures of synthetic Sirt1 activators are shown in Figure 2.
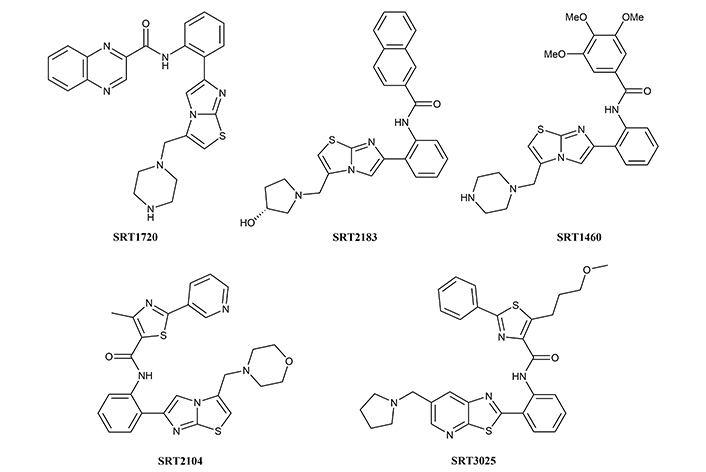
Chemical structures of first and second generation of synthetic activators of Sirt1
Synthetic activators of Sirt1 are widely studied, however, activation of the other mammalian sirtuins is not much reported. Interestingly, with the development in research, studies are being performed to find compounds that can activate and modulate other sirtuins. A prominent example of this is MDL-800, which has been found to activate Sirt6 by binding to an allosteric site and promoting histones H3K9 and H3K56 deacetylation in human hepatocellular carcinoma cells [107]. MDL-800 is reported to counteract age-related decline in DNA repair in murine-derived cells [108]. A series of novel pyrrolo[1,2-a]quinoxaline-based derivatives has been identified as potent selective activators of Sirt6 by repressing LPS-induced proinflammatory cytokine/chemokine secretion [109].
NAD+ boosting molecules
Sirtuins can be activated by regulating the cellular level of NAD+. Two approaches can be followed to modulate NAD+ levels; direct restoration of NAD+ levels by NAD+ precursor supplementation, and overexpression of enzymes related to NAD+ synthesis, NAMPT, and nicotinamide mononucleotide adenyltransferase (NMNAT), to enhance the rate of NAD+ synthesis or by inhibiting CD38 NAD+ hydrolase [110]. The NAD+ precursor supplements include nicotinamide mononucleotide (NMN) and nicotinamide riboside (NR) [111, 112]. A recent class of sirtuin activators called NAD+ boosting molecules is gaining popularity as a technique to restore NAD+ levels in aged individuals and potentially activate all seven sirtuins by a single molecule. NMN supplementation for a long time mitigates physiological age-associated decline in mice by improving energy metabolism and physical activity [113]. NMN supplementation has partially restored skeletal muscle loss and, also protects against β-amyloid oligomer-induced cognitive impairment and neuronal death in an Alzheimer’s model of mice [114, 115].
Molecular docking as a tool for studying sirtuin activators
Much insight into the mechanism of action and drug discovery today comes about due to the use of computational methods. Molecular docking and dynamics simulations are increasingly used to predict how molecules interact with their targets and predict ligand-target interactions [116, 117]. These techniques reduce the need for extensive laboratory testing, accelerating the drug development process. Docking studies have highlighted the potential of bioactive compounds from Curcuma zanthorrhiza, such as curcumin, as promising candidates for reducing age-related diseases through the Sirt1/NF-κB signaling pathway [118]. Additionally, research on resveratrol has demonstrated its role in stabilizing Sirt1/peptide interactions, offering insights for designing new substrate-specific Sirt1 modulators [119]. In silico approaches to discover bioactive compounds such as Sirt1 activators and NF-κB inhibitors, evaluating their toxicity and potential therapeutic benefits, have also been shown for metformin an important anti-diabetic drug. Analysis of metformin’s interaction with Sirt1 through molecular docking and dynamic simulation provides insights into its binding patterns and potential as a Sirt1 agonist [120].
Conclusions
With the advancement in studies related to sirtuin activators, the field of biogerontology is stepping into a new world of possibilities. It has great potential for future pharmacological interventions to slow aging. The family of mammalian sirtuins has risen to prominence in the field of aging research since the discovery of the yeast Sir2. Studies accumulating over the years have revealed that sirtuins exert protective functions against aging and age-related pathologies. Although the localization of sirtuins varies at the cellular level, their unique functions lead them to a common goal of promoting and preventing aging, marking them as promising targets for developing interventions for several age-related diseases. Since the discovery of resveratrol as an activator of sirtuins, efforts have been made by researchers to put forward certain molecules, which can activate all seven members of the sirtuin family and alleviate the features associated with aging. A small number of natural compounds like curcumin, fisetin, etc., have proved to be beneficial only up to a limited extent. Chemically synthesized molecules like SRT2104 showed promising results initially, and are undergoing clinical trials for various age-related diseases like diabetes, neurodegeneration, Parkinson’s, etc. However, an area of concern arises in the form of safety for humans. There are conflicting data in vitro, leading to assumptions that Sirt1 may act as an oncogene, despite the other health benefits. Also, a thorough understanding of the role of all isoforms of sirtuins is necessary before designing activators that target them, as a lapse in understanding the role of sirtuins may have a negative impact on other related molecules and processes.
Abbreviations
AMPK: | AMP-activated protein kinase |
CR: | calorie restriction |
FOXO3a: | forkhead box protein O3a |
GDH: | glutamate dehydrogenase |
IGF-1: | insulin-like growth factor-1 |
IL: | interleukin |
NAD+: | nicotine adenine dinucleotide |
NAMPT: | nicotinamide phosphoribosyl transferase |
NMN: | nicotinamide mononucleotide |
Nrf2: | nuclear factor erythroid 2-related factor 2 |
PGC-1α: | peroxisome proliferator-activated receptor-γ co-activator 1α |
SOD2: | superoxide dismutase 2 |
SREBP: | sterol regulatory element-binding protein |
Declarations
Acknowledgments
The authors thank the Department of Biochemistry, North-Eastern Hill University, Shillong, for support of research facilities under UGC-SAP, DBT, and DST-FIST, New Delhi.
Author contributions
PS: Conceptualization, Investigation, Writing—original draft, Writing—review & editing, Visualization. AKR: Investigation, Writing—original draft, Writing—review & editing. DS: Conceptualization, Validation, Writing—review & editing, Supervision. The authors read and approved the submitted version.
Conflicts of interest
The authors declare that they have no conflicts of interest.
Ethical approval
Not applicable.
Consent to participate
Not applicable.
Consent to publication
Not applicable.
Availability of data and materials
Not applicable.
Funding
The study was supported by the Department of Biochemistry, North-Eastern Hill University, Shillong, under UGC-SAP, DBT, and DST-FIST, New Delhi. The funders had no role in study design, data collection and analysis, decision to publish, or preparation of the manuscript.
Copyright
© The Author(s) 2025.
Publisher’s note
Open Exploration maintains a neutral stance on jurisdictional claims in published institutional affiliations and maps. All opinions expressed in this article are the personal views of the author(s) and do not represent the stance of the editorial team or the publisher.