Abstract
Aim:
This research utilizes the ethanolic extract from seeds of Monodora myristica and its biosynthesized selenium nanoparticles (SeNPs) for the treatment of diabetic nephropathy (DN) in male Wistar rats.
Methods:
Biosynthesis of crystalline, quasi-spherical shape 5.0 ± 0.25 nm SeNPs was achieved using M. myristica seed extract as a reducing agent, followed by surface plasmon resonance measurement by ultraviolet-visible (UV-Vis) spectrophotometer, Fourier transformed infrared spectroscopy (FTIR), high-resolution transmission electron microscopy (HR-TEM), and selected area electron diffraction (SAED) for confirmation of nanoparticle biosynthesis. Male Wistar rats were induced with diabetes using a 3-weeks high-fat diet and a single intraperitoneal injection of streptozotocin (STZ). Experimental groups included normal control group, untreated diabetic controls and those treated with either metformin (a standard drug), ethanolic extract or biosynthesized SeNPs of M. myristica seed extract. Biochemical analyses assessed renal function via serum creatinine and urea levels. Histological evaluations of kidney tissues were performed to assess structural changes.
Results:
Treatment with M. myristica seed extract and its biosynthesized SeNPs significantly improved renal function, evidenced by reduced serum creatinine and urea levels. Histopathological studies showed preserved renal architecture and reduced inflammatory damage, particularly in the combination therapy group, indicating a synergistic effect.
Conclusions:
This study highlights the potential of M. myristica and its biosynthesized SeNPs in mitigating DN through nephroprotective mechanisms. These findings advocate for the exploration of nanotechnology combined with bioactive plant compounds as effective strategies for managing DN.
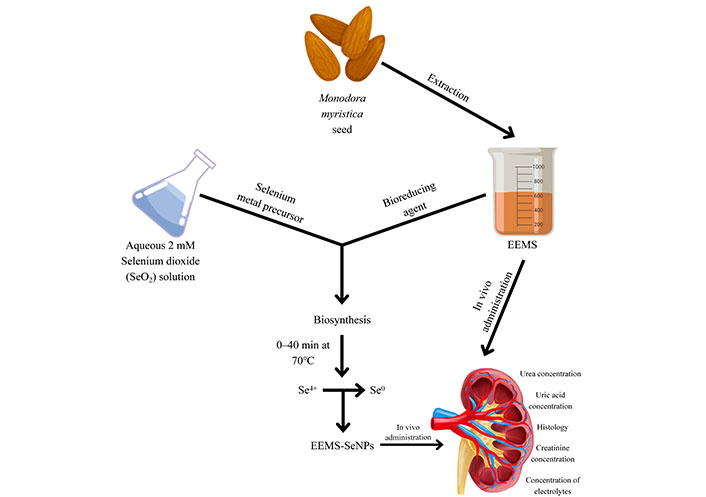
Keywords
Monodora myristica, selenium nanoparticles, diabetic nephropathy, nephroprotection, nanomedicine, streptozotocin, oxidative stressIntroduction
Diabetic nephropathy (DN), a major complication of diabetes, is characterized by persistent albuminuria and progressive kidney dysfunction, affecting 20–50% of diabetic patients [1]. Diabetes mellitus (DM) is a chronic metabolic disorder marked by hyperglycemia due to insulin resistance or insufficient insulin secretion [2]. As the prevalence of DM continues to rise globally, with projections estimating 55 million cases by 2045, effective strategies for managing DN and preventing associated complications like neuropathy and retinopathy remain critical [3].
The oxidative stress underlying DN pathogenesis necessitates exploring therapeutic options with antioxidative capabilities. Selenium (Se), an essential micronutrient and a critical component of seleno-proteins, plays a pivotal role in promoting health due to its antioxidative, and anti-inflammatory properties [4]. It is integral to the body’s antioxidant defense system and redox homeostasis, underscoring its therapeutic significance in conditions like DN, where oxidative stress is a major pathological driver [5]. Recent advancements in nanotechnology have spotlighted selenium nanoparticles (SeNPs) as innovative formulations that not only have low toxicity but also enhance selenium’s bioavailability [5]. The nanoscale dimensions of SeNPs increase its surface area and reactivity, facilitating improved cellular uptake and enabling the targeted delivery of bioactive compounds [6]. Also, the nano-dimensions of SeNPs could enable deep tissue penetration, enhanced permeability, and retention at inflamed nephrons [7], while biocompatibility and phytochemical capping from Monodora myristica seed oil optimize targeted delivery. Collectively, these attributes position SeNPs as promising candidates for addressing oxidative stress and its associated complications in DN.
Natural products, including medicinal plants, offer a complementary approach to conventional diabetes treatments. M. myristica, an African spice with documented antidiabetic, anti-inflammatory, and antioxidant properties, has shown potential in inhibiting key enzymes involved in glucose metabolism [8]. According to a study by Okonji et al. [8], ethanolic extract of M. myristica seeds has the potential to prevent diabetes through impeding the action of pancreatic α-amylase in the conversion of starch and glycogen to glucose, hence, lowering glucose release and absorption in the bloodstream. However, translating this in vitro efficacy into practical therapeutic agent requires optimization of its bioactivity and delivery in animal model. This study leverages the advantages of SeNPs by incorporating M. myristica ethanolic extract into a biosynthesized nanoparticle formulation. The combined antioxidant and anti-inflammatory effects of this nano-formulation target DN’s underlying mechanisms, offering a novel approach to managing the condition.
Despite evidence supporting the role of nanoparticles in treating various diseases, including cancer and microbial infections [9, 10], the use of nanoparticles in the management of DN, particularly in vivo, remains underexplored. This study addresses this gap by investigating the therapeutic potential of M. myristica-derived SeNPs in an in vivo DN model. By emphasizing the bioavailability and synergistic effects of selenium and the plant extract, this research provides insights into an innovative strategy for DN management. The study also underscores the importance of integrating traditional medicinal knowledge with advanced nanotechnology to develop cost-effective and efficient treatments for diabetes-related complications.
Materials and methods
Materials
Collection of plant material
Dried seeds of African Nutmeg (Monodora myristica) used for this study were purchased from Owode market in Offa, Kwara State, Nigeria on July 4, 2022, and authenticated at the Department of Plant Biology, Herbarium unit, University of Ilorin. A voucher number (UILH/001/1592/2023) was assigned and the specimen was deposited.
Drugs, chemicals, reagents and assay kit
Metformin was purchased from Impulse Pharma Pvt Ltd Tarapur, Boisar, India. All chemicals and reagents used for the study were of analytical grade. Selenium dioxide powder (99.99%), ethanol (99.99%) and n-hexane were supplied by BDH Chemicals Ltd. Poole England. Dimethyl sulfoxide (DMSO; 99%) was purchased from Loba Chemie Pvt. Ltd. (India). Assay kits for urea, uric acid, creatinine, sodium, potassium, chloride, and magnesium were products of Randox Laboratories Ltd., Co-Antrim, UK. Streptozotocin (STZ) obtained from Sigma Chemical Company, USA was administered to induce diabetes in rats.
Apparatus and instrument
Rotary evaporator and its components (Stuart model, Cole-Parmer, UK), Blender (ES-242, Eurosonic, UK), Centrifuge (CGOLDENWALL 80-2, USA), Freeze dryer (VaCo 10, Zirbus technology, Germany), ultraviolet-visible (UV-Vis) spectrophotometer (Systronics AU-2700, India), Glucometer (Accu-chek® Roche Diagnostic, Manheim, Germany), Light microscope (AM Scope MD500, USA), Weighing balance (PL303, Mettler Toledo, China) and Oven (TT-9023A, Techmel & Techmel, USA).
Experimental animals
From the University of Ilorin Animal House, Nigeria, forty (40) males healthy Wistar rats (Rattus norvegicus) with an average weight of 156.18 ± 0.05 g were collected. The rats were acclimated in clean plastic cages at room temperature, with enough ventilation, and given free access to eat rat pellets and drink clean tap water ad libitum.
Methods
Preparation of ethanolic extract of M. myristica seed (EEMS) and its biosynthesized nanoparticles (EEMS-SeNPs)
Seeds of M. myristica were de-shelled and oven-dried to obtain constant weight. The dried seeds were then pulverized and 100 g of the pulverized seeds were weighed into two different jars, 200 mL of n-hexane was added, vigorously shaken and left for 24 h for defatting. This procedure was repeated 3 times to ensure all the non-polar components were completely extracted. The mixture was filtered, 200 mL of ethanol was added to the residue contained in 2 jars for exhaustive extraction for another 24 h and filtered. This process was also performed three times. The ethanolic extract obtained was concentrated using a rotary evaporator and described as EEMS. The EEMS was reconstituted in a solution of 0.5% DMSO and distilled water to give desired doses [25, 50 and 100 mg/kg body weight (b.wt)].
Method for preparing nanoparticles
The method described by Akinfenwa et al. [11] was adopted with slight modifications. EEMS was used for the biosynthesis of green SeNPs via bio-reduction process. Briefly, drops of 10 mL (10 mg/mL) EEMS was added to freshly prepared 90 mL (2 mM) colorless solution of SeO2 on stirring at 70℃ and refluxed for 3 h. A color change from colorless to pink-red was visually observed during the process. For the control sample, 10 mL of deionized water (DW) was added to 90 mL of 2 mM SeO2 which showed no color change. Further, biosynthesized SeNPs were separated by centrifugation at 10,929.4 × g for 10 min, washed thoroughly in distilled water, freeze dried and stored for further usage. The surface plasmon resonance of synthesized EEMS-SeNPs was measured using UV-Vis spectrophotometer at wavelength of 200–800 nm.
Animal grouping and extract administration
A total of forty (40) male Wistar rats were used in this study. The animals were randomly assigned into eight groups (A–H) of five (5) animals each as shown in Table 1.
Animal group and extract administration
Groups | 14-days oral administration |
---|---|
A (NDR) | 0.5 mL of dH2O |
B (DR + dH2O) | 0.5 mL of dH2O |
C (DR + metformin) | 0.5 mL 500 mg/kg b.wt of metformin |
D (DR + 25 mg/kg EEMS) | 0.5 mL of 25 mg/kg b.wt of M. myristica seed extract |
E (DR + 50 mg/kg EEMS) | 0.5 mL of 50 mg/kg b.wt of M. myristica seed extract |
F (DR + 100 mg/kg EEMS) | 0.5 mL of 100 mg/kg b.wt of M. myristica seed extract |
G (DR + 10 mg/kg SeNP) | 0.5 mL of 10 mg/kg b.wt of selenium nanoparticle |
H (DR + 20 mg/kg SeNP) | 0.5 mL of 20 mg/kg b.wt of selenium nanoparticle |
NDR: non-diabetic rats; DR: diabetic rat; dH2O: distilled water; b.wt: body weight; EEMS: ethanolic extract of M. myristica seed; SeNPs: selenium nanoparticles
Ethics statement
The current study was performed following the guidelines provided by Cadmus and and Daramola [12] to ensure replacement, reduction, and refinement in the protocol used. Forty adult male healthy Wistar rats (Rattus norvegicus; average body mass 156.18 ± 0.05 g) from the University of Ilorin Animal House, Nigeria, were used in this experiment. Rats were housed, fed and handled in clean plastic cages (5 per cage) at room temperature and humidity (20–25℃, 40–50%) under a 12:12-h light/dark cycle with enough ventilation. Animals were given free access to normal rodent pellets diet and clean tap water ad libitum during the acclimatization. All the animals were treated in accordance with the “Guidelines for Care and Use of Experimental Animals” issued by Summit University, Offa Nigeria with approval number: SUERC/CONAS/2023/002.
Anesthesia method
Inhalation anesthesia using isoflurane protocol previously reported by Hohlbaum et al. [13] was adopted with slight modifications. Briefly, the rats were placed in anesthetic chamber and anesthesia was induced with 4% isoflurane (Isoflurane CP®, CP-Pharma Handelsgesellschaft mbH, Burgdorf, Germany) in 100% oxygen in the anesthetic chamber (with sliding cover, Evo-nik Plexiglas, 240 mm × 140 mm × 120 mm), which was not prefilled in order to prevent distress. During induction, the number of rats showing excitations was recorded. Parameters such as breathing rate and activities were monitored until the animals went into complete loss of behavioral responsiveness (sleeping mode). Summarily, the study used inhalation anesthesia method to induce the animals before a painless sacrifice of the animals.
Induction of type 2 diabetes mellitus and treatment
The animals were fed with high fat diet (compounded using 60% animal feed, 38% lard, and 2% cholesterol) for 3 weeks. After an overnight fast of the animals, type 2 diabetes was induced by administering a single intraperitoneal injection of 35 mg/kg b.wt of STZ, following a method similar to that described by Ramadan et al. [14] with minor adjustments. The blood glucose levels of the rats were assessed 72 h after STZ injection. Blood samples were obtained from the tail vein, and these samples were placed on a test strip inserted into a glucometer. Rats with blood glucose levels equal to or exceeding 250 mg/dL were identified as diabetic and subsequently used in the ensuing experiments [15].
Diabetic animals used for this study were treated with various dosages of EEMS (25, 50, and 100 mg/kg b.wt of the rats) and EEMS-SeNP (10 and 20 mg/kg b.wt of the rats) as well as metformin for 14 days. Dosage selection for EEMS, SeNPs, and metformin were based on safe level reported in similar study in the literature [16, 17].
Preparation of serum and tissue supernatants
After an overnight fast of the rats, they were sacrificed on the fifteenth day. The rats were anaesthetized in an anesthetic chamber induced with isoflurane; the jugular veins were cut with a sharp, sterile blade. For serum analyses, blood was drawn into plain sample tubes. Blood samples were centrifuged at 658 × g for 10 min after standing at room temperature for 15 min. The serum-containing supernatant was decanted into sterile sample bottles following centrifugation. The resulting sera were appropriately labeled and kept in the freezer at 4℃ for no longer than 72 h prior to the biochemical assay preparation [15].
The kidney was harvested, and blotted with tissue paper. Portions of the kidney were preserved in 10% buffered formal saline solution respectively for histological analysis. Kidney homogenate was prepared by homogenizing 1 g of in 3 mL 0.25 M TRIS-buffer in an ice cold medium, and centrifuged at 1589 × g for 10 min. The supernatant was collected by decantation and stored frozen until required but used within 72 h of preparation [15].
Determination of biochemical parameters and histological examination
With slight modification, the method employed by Veniamin and Vakirtzi-Lemonias [18] was utilized for the measurement of urea concentration. Uric acid concentration was determined using the method described by Tietz [19], creatinine concentration as described by Bartels et al. [20], and serum electrolytes (Ca, Na, Cl, Mg and K) as described by Tietz [19]. Histology was done following the method described by Drury and Wallington [21].
Statistical analysis
The data were expressed as a mean ± standard deviation (SD) of five replicates. Data were statistically analyzed by one-way analysis of variance (ANOVA) followed by Turkey’s multiple comparisons. Statistically significance was set at 95% confidence interval (P < 0.05) and graph pad prism version 8.0.2 (263) for Windows, statistical analysis was performed using GraphPad Software, San Diego, California, USA. A significance level of P < 0.05 was considered.
Results
Biosynthesis of EEMS-SeNPs
Optical property and stability
The seed extract of M. myristica was utilized as a reducing and capping agent for the formation of EEMS-SeNPs. During synthesis, formation of EEMS-SeNPs was visually monitored by observing the color change due to the addition of 20 mg/mL EEMS to heated solution of selenium (Se4+) precursor on stirring. The reaction mixture’s color changed from colorless to pink red indicating possible formation of the SeNPs (Figure 1). This color change could be attributed to the excited oscillating surface electrons of SeNPs at a resonance wavelength, a phenomenon known as surface plasmon resonance. The surface plasmon resonance action was recorded by UV spectrophotometer at 294 nm reflecting a redshift; an increased in wavelength from initial 285 nm to 294 nm (Figure 2). The stability of SeNPs was evaluated by monitoring the UV absorbance spectrum at different time points (6 h intervals for 3 days). The SeNPs showed approximately the same wavelength equal to 294 nm (wavelength of the control sample), suggesting that the SeNPs were stable. Studies on UV absorbance of SeNPs derived from microbes have been reported to show higher values (450–600 nm) than those obtained from plant extracts (200–400 nm) due to bioavailability of biomolecules [22]. The result from this study agrees closely with the report of green synthesis of SeNPs from Ceropegia bulbosa Roxb. var. aqueous extract [23]. Similarly, phytochemical reports on M. myristica revealed that the seed extract is a rich source of phytochemicals, including alkaloids, flavonoids, tannins, phenolic compounds, and saponins [24]. During the biosynthesis process, the combined activities of these phytochemicals may have contributed to the reduction the selenium ions to zero-valent oxidation state and capping of the SeNPs formed.
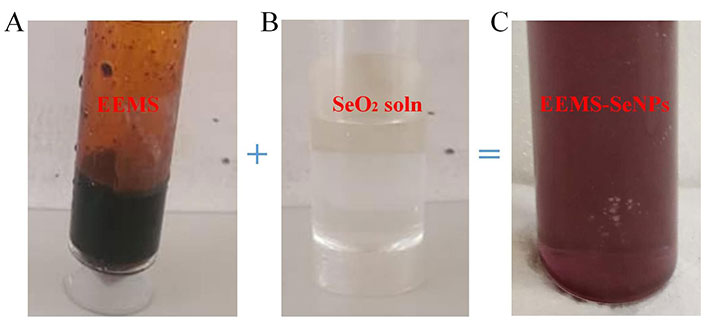
Color changes of the reaction medium. (A) EEMS; (B) SeO2 solution (before synthesis); (C) added EEMS to SeO2 solution (after synthesis)
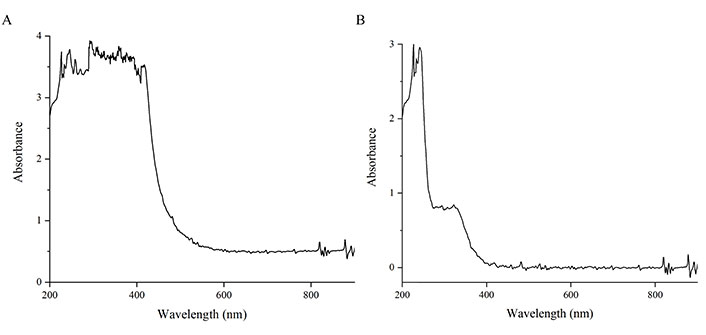
UV spectra of 2 mmol Se solution and EEMS-SeNPs. (A) UV spectra of 2 mmol Se solution; (B) UV spectra of EEMS-SeNPs
FTIR spectra of EEMS-SeNPs
The involvement of certain functional groups from EEMS in the synthesis of SeNPs was recorded on FTIR. Figure 3 represents the FTIR of EEMS and EEMS-SeNPs respectively. The strong band at 3,305.47 cm–1 corresponded to the O–H stretch vibration of phenols and alcohols in EEMS was shifted to 3,307.39 cm–1 in EEMS-SeNPs. Similarly, the band at 1,638.22 cm–1 assignable to carbonyl stretch (C=O) of flavonoids in EEMS became 1,631.5 cm–1 after synthesis of EEMS-SeNPs. Furthermore, the disappearance of the C–O stretching band of alcohol at 1,085.04 cm–1, C–H stretching vibrations at 2,981.15 cm–1 (possibly asymmetric –CH3 groups), and the 2,905.30 cm–1 (possibly symmetric stretching –CH2 groups) respectively in EEMS-SeNPS which where were initially present in EEMS also suggest their involvement in the bio-reduction and stability of biosynthesized nanoparticles. Summarily, the hydroxyl (OH), carbonyl (C=O), stretching C–O, and the C–H stretching vibration groups could be the interaction points between the EEMS agents and the SeNPs. This result is similar with report on synthesis of SeNPs from citric acid [17].
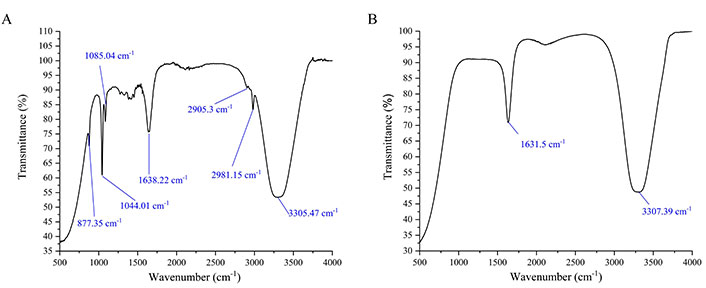
FTIR spectra of EEMS and EEMS-SeNPs. (A) FTIR spectra of EEMS; (B) FTIR spectra of EEMS-SeNPs
High-resolution transmission electron microscopy and selected area electron diffraction analysis
The morphology of EEMS-SeNPs recorded on high-resolution transmission electron microscopy (HR-TEM) as shown in Figure 4 reveals a non-aggregated, few pentagonal and mostly quasi-spherical shapes of the nanoparticles with size range of 5–5.5 nm which is similar to report in existing literature [25–27]. The crystallinity of the nanoparticles was further supported by bright circular rings observed in the micrograph of the selected area electron diffraction (SAED).
Effect of M. myristica seed extract and selenium nanoparticle on fasting blood glucose level of diabetic Wistar rats
This study evaluated the impact of M. myristica seed extract (EEMS) and its biosynthesized selenium nanoparticles (EEMS-SeNPs) on fasting blood glucose (FBS) levels in diabetes-induced Wistar rats across a duration of fourteen days (Table 2). On Day 0, FBS levels showed no significant variation across all groups, establishing a uniform baseline. By Day 1, 72 h after induction, all diabetic groups exhibited a significant (P < 0.05) increase in FBS levels compared to the non-diabetic control, reflecting the hyperglycemic effect of diabetes induction.
Effect of oral administration of ethanolic extract of M. myristica seed and selenium nanoparticle on fasting blood glucose levels in streptozotocin-induced diabetic rats
Parameters/Days | NDR | DR + dH2O | DR + metformin | DR + 25 mg/kg b.wt of EEMS | DR + 50 mg/kg b.wt of EEMS | DR +100 mg/kg b.wt of EEMS | DR + 10 mg/kg b.wt of SeNP | DR + 20 mg/kg b.wt of SeNP |
---|---|---|---|---|---|---|---|---|
Day 0 | 67.20 ± 7.50 a | 63.40 ± 13.43 a | 67.60 ± 8.26 a | 66.80 ± 11.65 a | 66.80 ± 10.45 a | 66.00 ± 10.93 a | 62.40 ± 11.13 a | 64.20 ± 10.33 a |
Day 1 | 71.20 ± 15.27 α | 418.40 ± 44.59 b | 347.40 ± 44.46 b | 426.80 ± 62.29 b | 354.80 ± 53.05 b | 440.00 ± 83.56 b | 432.00 ± 30.06 b | 458.00 ± 52.96 b |
Day 4 | 61.60 ± 8.71 a | 256.80 ± 23.53 b | 166.80 ± 20.25 c | 233.00 ± 53.32 b, c | 75.00 ± 14.98 a | 291.00 ± 37.16 b | 263.20 ± 57.10 b | 157.60 ± 44.40 c |
Day 7 | 50.00 ± 10.65 a | 276.00 ± 21.22 b | 141.00 ± 34.29 c | 130.60 ± 50.25 c | 51.20 ± 18.36 a | 273.80 ± 30.72 b | 153.00 ± 42.76 b, c | 194.40 ± 4.93 c |
Day 10 | 56.20 ± 6.65 a | 266.80 ± 26.50 c | 94.60 ± 27.51 a, b | 114.60 ± 57.57 a | 61.00 ± 14.30 a | 242.60 ± 68.23 c | 115.60 ± 6.66 a, b | 94.80 ± 19.84 a, b |
Day 13 | 60.00 ± 9.41 a | 284.80 ± 31.59 c | 79.20 ± 24.17 a | 127.60 ± 49.39 a, b | 64.80 ± 7.79 a | 227.20 ± 33.55 d | 70.80 ± 23.53 a | 80.00 ± 15.81 a |
Day 14 | 62.40 ± 11.52 a | 267.80 ± 25.77 b | 66.40 ± 13.61 a | 62.60 ± 8.05 a | 69.20 ± 11.17 a | 194.00 ± 43.15 c | 63.20 ± 8.04 a | 70.00 ± 21.89 a |
Values are means of five replicates ± STD. Values with different superscript are significantly different (P < 0.05) from others. Statistical significance was determined using superscripts (e.g., a, b, c and d) to indicate differences among groups at a significance level of P < 0.05 (5%). NDR: non-diabetic rats; DR: diabetic rats; dH2O: distilled water; SeNPs: selenium nanoparticles; b.wt: body weight; EEMS: ethanolic extract of M. myristica seed; Day 0: FBS level pre-induction
However, from Day 4 and onwards, there were changes in treatment-dependent differences. Diabetic rats administered 50 mg/kg b.wt of EEMS displayed a significant (P < 0.05) lower FBS levels which were almost similar to that of the non-diabetic control group. This particular pattern of behavior was observed during Day 10 and Day 14 as well, distinguishing the 50 mg/kg b.wt dose as particularly effective. In contrast, 25 mg/kg and 100 mg/kg b.wt of EEMS and 10 mg/kg b.wt EEMS-SeNPs groups were comparatively less efficient. The standard drug metformin consistently regulated glucose levels effectively across the treatment period.
By Day 14, FBS levels in groups treated with 50 mg/kg b.wt EEMS, metformin, and EEMS-SeNPs at 10 and 20 mg/kg b.wt were significantly (P > 0.05) comparable to the non-diabetic controls, whereas levels remained significantly elevated in groups receiving distilled water and 100 mg/kg b.wt EEMS, with FBS values significantly (P < 0.05) higher in the diabetic rats administered distilled water than in the 100 mg/kg b.wt EEMS group.
Effect of oral administration of ethanolic extract of M. myristica seed and selenium nanoparticle on urea level of diabetic male Wistar rats
Urea concentration was significantly decreased (P < 0.05) in all treatment groups when compared with the diabetic distilled water treated control group (negative control). This decrease in urea concentration across all treatment groups was however more significant in the rats administered 25 mg/kg b.wt of the extract compared with other treatment groups (Figure 5).
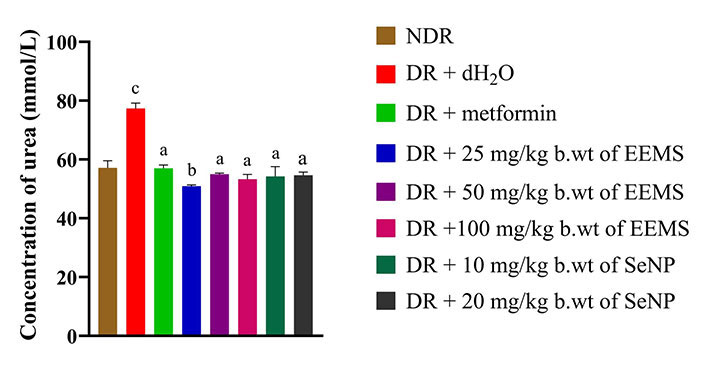
Urea level of diabetic Wistar rats administered ethanolic extract of M. myristica seed and selenium nanoparticle. Statistical significance was determined using superscripts (e.g., a, b and c) to indicate differences among groups at a significance level of P < 0.05 (5%). a: represents groups not significantly different from the control group; b: represents treated group significantly different from the control group; c: represents groups significantly different from both the control and other treatment groups. NDR: non-diabetic rats; DR: diabetic rats; dH2O: distilled water; b.wt: body weight; EEMS: ethanolic extract of M. myristica seed; SeNP: selenium nanoparticle
Effect of oral administration of ethanolic extract of M. myristica seed and selenium nanoparticle on uric acid level of diabetic male Wistar rats
The concentration of uric acid was significantly increased (P < 0.05) in distilled water administered diabetic rats when compared to the distilled water administered non-diabetic rats (Figure 6). Also, uric acid concentration was not significantly different (P > 0.05) in all treatment groups when compared to the distilled water administered non-diabetic rats (Figure 6).
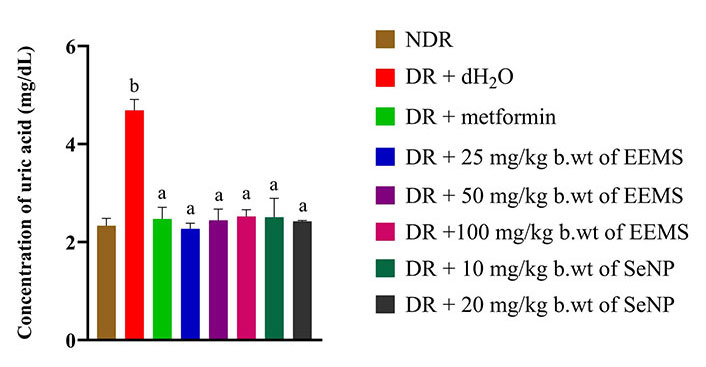
Uric acid concentration of diabetic rats administered ethanolic extract of M. myristica seed and selenium nanoparticle. Statistical significance was determined using superscripts (e.g., a and b) to indicate differences among groups at a significance level of P < 0.05 (5%). a: represents groups not significantly different from the control group; b: represents group significantly different from both the control and other treatment groups. NDR: non-diabetic rats; DR: diabetic rats; dH2O: distilled water; b.wt: body weight; EEMS: ethanolic extract of M. myristica seed; SeNP: selenium nanoparticle
Effect of oral administration of ethanolic extract of M. myristica seed and selenium nanoparticle on creatinine level of diabetic male Wistar rats
The level of creatinine was significantly (P < 0.05) increased in diabetic rats administered distilled water (negative control) when compared to non-diabetic rats. Whereas, a significant decrease (P < 0.05) in creatinine level was produced in all diabetic rats treated with 50, and 100 mg/kg b.wt of EEMS as well as 10 and 20 mg/kg b.wt SeNPs which produced no significant difference (P > 0.05) when compared the non-diabetic rats. Administration of 25 mg/kg b.wt of EEMS produced a significantly (P < 0.05) increased level of creatinine (Figure 7).
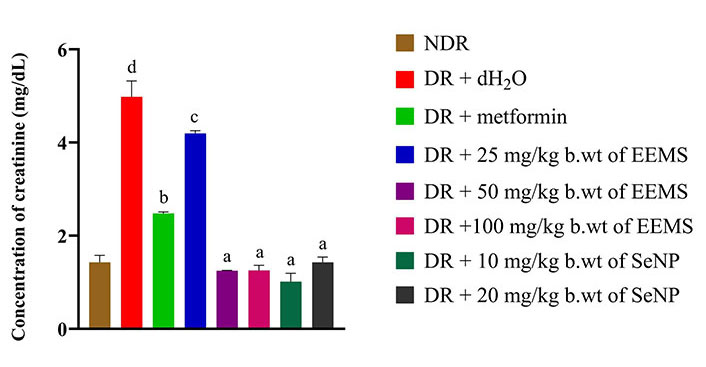
Creatinine concentration of diabetic rats administered ethanolic extract of M. myristica seed and selenium nanoparticle. Statistical significance was determined using superscripts (e.g., a, b, c, d and e) to indicate differences among groups at a significance level of P < 0.05 (5%). a: represents groups not significantly different from the control group; b: represents group significantly different from control group; c: represents group significantly different from both the control group and group treated with metformin; d: represents group significantly different from both the control and other treatment groups. NDR: non-diabetic rats; DR: diabetic rats; dH2O: distilled water; b.wt: body weight; EEMS: ethanolic extract of M. myristica seed; SeNP: selenium nanoparticle
Effect of ethanolic extract of M. myristica seed and selenium nanoparticle administration on the level of electrolytes of diabetic male Wistar rats
The concentration of calcium was significantly increased (P < 0.05) in diabetic rats administered distilled water (negative control) when compared to non-diabetic rats. Across all treated groups, only animals administered 25 mg/kg b.wt of M. myristica produced a significant decrease (P < 0.05) in calcium level when compared to dietetic rats administered distilled water (negative control) and no significant difference (P > 0.05) was produced when compared to the non-diabetic control rats (Figure 8). The oral administration of EEMS and SeNPs produced no significant alteration (P > 0.05) in sodium (Figure 9) and chloride (Figure 10) concentration in all treatment groups when compared to both distilled-water administered diabetic and distilled water administered non-diabetic control groups.
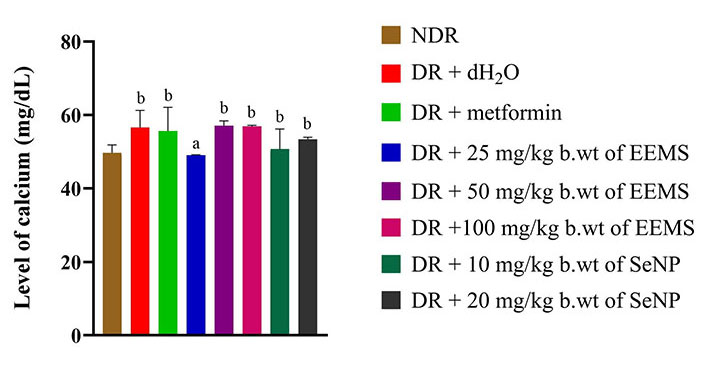
Calcium level of diabetic male Wistar rats administered ethanolic extract of M. myristica seed and selenium nanoparticle. Statistical significance was determined using superscripts (e.g., a and b) to indicate differences among groups at a significance level of P < 0.05 (5%). a: represents group not significantly different from the control group; b: represents groups significantly different from the control group. NDR: non-diabetic rats; DR: diabetic rats; dH2O: distilled water; b.wt: body weight; EEMS: ethanolic extract of M. myristica seed; SeNP: selenium nanoparticle
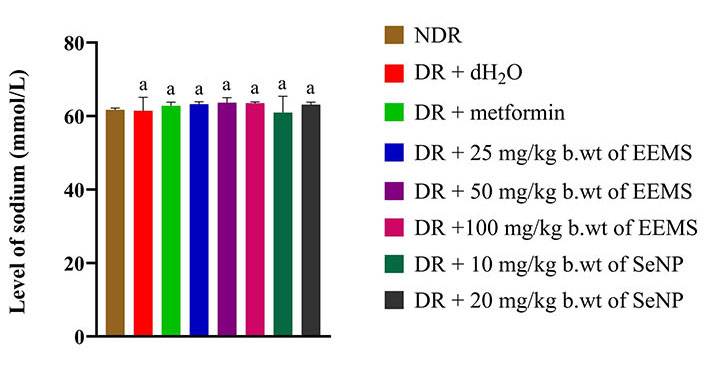
Sodium level of diabetic male Wistar rats administered ethanolic extract of M. myristica seed and selenium nanoparticle. Statistical significance was determined using superscripts to indicate differences among groups at a significance level of P < 0.05 (5%). a: represents groups not significantly different from the control group. NDR: non-diabetic rats; DR: diabetic rats; dH2O: distilled water; b.wt: body weight; EEMS: ethanolic extract of M. myristica seed; SeNP: selenium nanoparticle
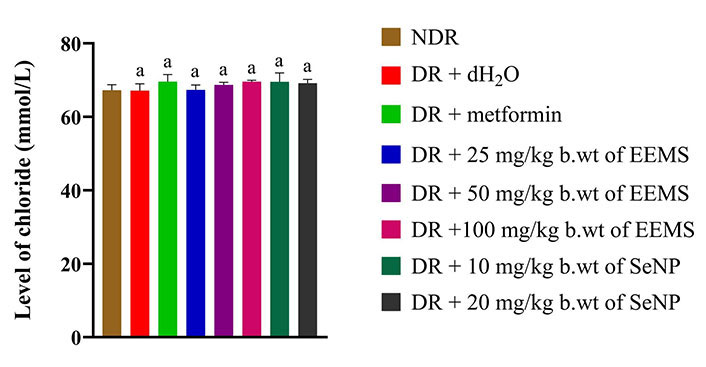
Chloride level of diabetic male Wistar rats administered ethanolic extract of M. myristica seed and selenium nanoparticle. Statistical significance was determined using superscripts to indicate differences among groups at a significance level of P < 0.05 (5%). a: represents groups not significantly different from the control group. NDR: non-diabetic rats; DR: diabetic rats; dH2O: distilled water; b.wt: body weight; EEMS: ethanolic extract of M. myristica seed; SeNP: selenium nanoparticle
There was a slight significant (P < 0.05) decrease in the level of magnesium in diabetic rats administered distilled water (negative control) when compared with the normal control and other treated diabetic rats (Figure 11). There was a significant (P < 0.05) increase in the level of potassium in the serum of diabetic rats treated with metformin when compared to the normal control group, diabetic rats administered distilled water (negative control) and other treated groups (Figure 12).
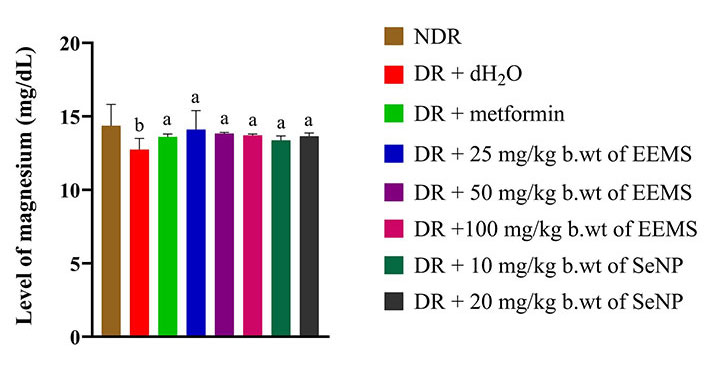
Magnesium level of diabetic male Wistar rats administered ethanolic extract of M. myristica seed and selenium nanoparticle. Statistical significance was determined using superscripts (e.g., a and b) to indicate differences among groups at a significance level of P < 0.05 (5%). a: represents group not significantly different from the control group; b: represents untreated diabetic group different from both the control group and other treatment groups. NDR: non-diabetic rats; DR: diabetic rats; dH2O: distilled water; b.wt: body weight; EEMS: ethanolic extract of M. myristica seed; SeNP: selenium nanoparticle
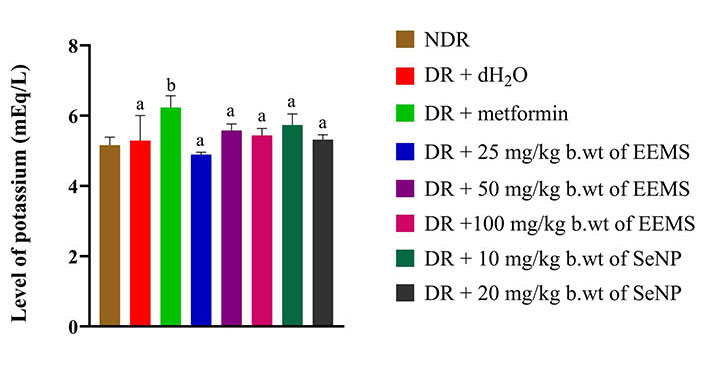
Potassium level of diabetic male Wistar rats administered ethanolic extract of M. myristica seed and selenium nanoparticle. Statistical significance was determined using superscripts (e.g., a and b) to indicate differences among groups at a significance level of P < 0.05 (5%). a: represents groups not significantly different from the control group; b: represents group significantly different from the control group. NDR: non-diabetic rats; DR: diabetic rats; dH2O: distilled water; b.wt: body weight; EEMS: ethanolic extract of M. myristica seed; SeNP: selenium nanoparticle
Kidney histology of streptozotocin-induced diabetic rats orally administered ethanolic extract of M. myristica seed and selenium nanoparticle
The non-diabetic control group (Figure 13A) showed no alteration in the normal kidney architecture of the non-diabetic control group and cut sections of glomeruli were intact. On the other hand, diabetic rats administered distilled water (negative control, Figure 13B) manifested distorted histo-architecture including broadening of capsular space, infiltration of inflammatory cells, reduced thickness of cortex and loss of glomeruli. Milder changes of the renal cells from mild to moderate were also noted in the metformin treated group (Figure 13C), EEMS at the doses of 25, 50 and 100 mg/kg b.wt. (Figure 13D–F) and EEMS-SeNPs at doses of 10 and 20 mg/kg b.wt. (Figure 13G and H).
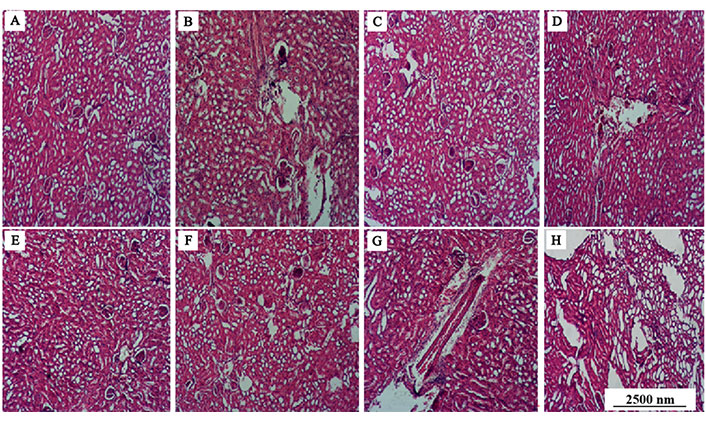
Cross section of the kidney of the rats administered ethanolic extracts of M. myristica seed and selenium nanoparticle (magnification: ×10; H&E). (A) 0.5 mL of dH2O; (B) 20 mg/kg b.wt of SeNPs; (C) 100 mg/kg b.wt of EEMS; (D) 10 mg/kg b.wt of SeNPs; (E) 50 mg/kg b.wt of EEMS; (F) diabetic rats orally administered 0.5 mL of dH2O; (G) 25 mg/kg b.wt of EEMS; (H) metformin
Discussion
Diabetes is one of the most prevalent metabolic diseases, marked by increased blood sugar levels causing deteriorative effects on the kidneys and other organs [28]. The World Health Organization (WHO) reported that DM is the leading cause of kidney failure globally [29, 30]. The bean-shaped kidneys are two retroperitoneal organs with a reddish-brown appearance. The kidney has a special capacity for gluconeogenesis, or the production of glucose from carbon sources that are not carbohydrates, much like the liver does. The kidneys process plasma continuously through filtration, reabsorption, and secretion of the chemicals, regulating the volume and composition of extracellular and intracellular fluid to maintain the body’s homeostasis [31]. When affected by DN, the kidneys become characterized by increased urinary albumin excretion, decreased glomerular filtration rate (GFR), or both. This is a significant complication that develops in as many as 40% of diabetic individuals [30].
FBS serves as a significant marker for diagnosing diabetes. The rise in FBS levels observed in the diabetic rats treated with distilled water in this study can be attributed to a decrease or absence of insulin production by the pancreatic beta cells, which may have been caused by the destruction of the beta cells in the pancreas [32] and/or the inability of the body to utilize the insulin produced well (insulin resistance) [33]. The decrease in FBS levels observed in diabetic rats after the administration of EEMS indicates its ability to ameliorate diabetic nephrotic conditions. These findings are consistent with previous studies that have reported reduced FBS levels in diabetic animals treated with different oral anti-diabetic agents [24, 34] as well as formulated biosynthesized nanoparticles [35–37]. Interestingly, the observed dose-response effect in this study revealed that lower doses (i.e. 25 and 50 mg/kg b.wt) of EEMS were more effective in reducing FBS levels compared to the higher dose of 100 mg/kg. This phenomenon may be attributed to a biphasic or bell-shaped dose-response curve often reported with phytochemicals and bioactive compounds [38, 39]. At higher concentrations, these compounds can exert opposing effects, such as inducing oxidative stress or overwhelming cellular antioxidant defenses, thereby diminishing their therapeutic efficacy [40].
The kidney plays a central role in waste removal, water and electrolyte balance, and pH regulation, with serum urea, uric acid, creatinine, and electrolytes serving as critical indices of renal excretory function [41]. Urea, the primary nitrogenous byproduct of amino acid catabolism, is synthesized in the liver and diffuses into bodily fluids before renal excretion [31]. Elevated serum urea levels, as observed in untreated diabetic animals, may signal a reduced GFR, a characteristic feature of DN. This condition can also stem from dehydration, a common symptom associated with diabetes. Excess urea can exacerbate insulin resistance and suppress insulin secretion, further impairing glucose homeostasis in diabetes [42, 43]. In this study, the administration of EEMS and SeNPs effectively reversed elevated urea levels in diabetic rats, achieving values comparable to those in non-diabetic controls. The most significant reduction was noted in animals treated with 25 mg/kg b.wt of EEMS, highlighting its potential nephroprotective effects. The observed reversal of urea levels aligns with studies by Anjaneyulu and Chopra [44] and Hao et al. [45], which utilized isolated compounds from various plants to demonstrate their nephroprotective potential in experimentally induced DN. Additionally, Hassan et al. [17] corroborated the efficacy of SeNPs in enhancing renal function through reduced urea concentration and improved GFR.
Uric acid is the end product of purine metabolism and is mainly eliminated through the renal system, and its excessive values appear in many cases of kidney and other metabolic disorders [46]. This biological function is important in relation to diabetes, because people with diabetic conditions are characterized by a high concentration of uric acid, due mainly to a filtration dysfunction of the kidneys as well as low GFR [47]. There are other studies such as those by Aktas et al. [48] which demonstrate that hyperuricemia is even worse in diabetic state and it supports the role of uric acid in the further development of diabetes complications. The increase in the uric acid level of diabetic rats receiving distilled water might be due to either increased production or decreased excretion of uric acid by the kidney. Increased serum uric acid, if unregulated, can precipitate hyperuricemia which is known to cause gout as well as increase oxidative stress, endothelium dysfunction, and systemic inflammation. Such pathophysiological alterations lead to microvascular complications that also involve nephropathy, among the most troubling aspects in the management of diabetes [49]. The use of EEMS and SeNPs clearly reduced serum uric acid in diabetic rats virtually to non-diabetic controls. This points to a two-pronged approach of improved clearance of uric acid and a change in renal function. In a comparative study, Abd El-Khalik et al. [50] emphasized the reno-protective attributes of zinc oxide nanoparticles (ZnO NPs) in the case of DN and elaborated pathways through which the effects are exerted, namely inflammatory processes and oxidative stress. ZnO NPs, as with EEMS and SeNPs, were able to restore the elevation of renal markers, such as uric acid.
Creatinine, a breakdown product of creatine phosphate, serves as a critical biomarker for assessing renal function due to its steady production rate and glomerular filtration-dependent elimination [31, 51]. Elevated creatinine levels in the bloodstream typically indicate a reduction in GFR, often resulting from kidney damage or dysfunction. Diabetes exacerbates this condition by inducing hyperglycemia, which leads to glomerular hypertrophy, sclerosis, and tubulointerstitial inflammation, impairing the filtration capacity of the kidneys [17, 52]. In the present study, the significant increase in creatinine levels observed in diabetic rats receiving distilled water aligns with these pathological mechanisms, reflecting the damage to the glomeruli and reduced GFR. Interestingly, rats treated with 25 mg/kg b.wt EEMS and metformin exhibited elevated creatinine levels compared to the normal group, albeit lower than untreated diabetic rats. This could be attributed to drug-induced renal stress [53, 54], which damages the glomeruli in the kidney and can lead to impaired filtration capacity causing increased levels of creatinine in the blood. Administration of 50 and 100 mg/kg b.wt EEMS and SeNPs effectively reversed this trend, bringing serum creatinine levels in treated diabetic rats close to those of non-diabetic controls. This suggests a nephroprotective effect likely mediated by antioxidative properties, as similar outcomes have been observed with other plant-derived compounds and nanoparticles [45, 50]. Comparatively, studies involving plant-isolated compounds [44] and SeNPs [17] demonstrate similar efficacy in reducing serum creatinine levels, supporting the therapeutic potential of EEMS and SeNPs.
Electrolytes are essential charged ions that maintain critical cellular functions such as nerve impulse transmission, muscle contraction, and fluid balance [55]. They serve as important markers for assessing renal function because their regulation—via filtration, reabsorption, and excretion—is mediated by the kidneys [56]. Sodium and chloride, key extracellular ions, are vital for fluid balance, nerve signal transmission, and acid-base regulation [57]. The results revealed no significant changes in the serum levels of sodium and chloride in treated diabetic rats compared to controls, suggesting that EEMS and SeNPs do not interfere with the kidney’s ability to secrete or reabsorb these ions.
Calcium is vital for bone health, blood clotting, proper muscle contraction, skeletal mineralization, nerve impulse transmission, hormone secretion, insulin production, and glucose uptake in cells [58–60]. In some treated diabetic rats which received just distilled water, calcium levels were raised as a consequence of renal impairment and abnormal deposition of calcium due to DN. However, interestingly in our study, calcium levels were at doses of EEMS and SeNPs elevated as well, except for the rats administered 25 mg/kg b.wt which has a normal calcium level, which could be due to the prevention of calcium absorption into urine by the extract and metformin. This normalization of calcium level by the administration of 25 mg/kg b.wt is very important as it helps in avoidance of nephrocalcinosis and other renal disorders associated with hypercalcemia [61]. As an important internal cation, potassium is responsible for rest membrane potential and cellular excitability [62]. The hyperkalemia seen in diabetic conditions is mostly due to decreased renal potassium elimination. The results of the study showed that treated diabetic rats had stable potassium levels implying that the increased levels were due to the diabetic condition rather than the treatment. Such findings agree with Wagle et al. [63] who also conducted a similar study and found out that the treatments would maintain potassium to normal levels without any toxic effects. Magnesium, a cofactor for many enzymes which is also important for energy metabolism [64] as well as proper functioning of muscles, neurological functioning, and neurotransmitter release [65], can be limited under diabetic conditions and aggravates insulin resistance and inflammation [66, 67]. The treated diabetic rats displayed normalized magnesium levels, similar to those in the non-diabetic control group, indicating that EEMS and SeNPs restored magnesium homeostasis. This is important as magnesium has been known to enhance insulin sensitivity and reduce resistance as indicated by Barbagallo and Dominguez [68] and Feng et al. [69]. In addition, these results correspond with Liu et al. [70] and Parvizi et al. [71] studies, which also demonstrate the protective effect of magnesium on kidney functions and the improvement of glucose metabolism in diabetic models.
Histological examination of tissue sections can provide supplementary evidence to the biochemical assessment [72]. Administration of the doses of ethanolic extract of M. myristica seed and SeNPs led to a mild renal cellular alteration which can be deduced from the overly large capsular spaces, inflammatory cells, degenerative changes, atrophilic thinning of the cortex and few losses of glomeruli. However, there was no significant loss of cellular integrity. The findings from the study corroborates the report by Selby and Taal [1] which identified glomerular features, interstitial fibrosis and tubular atrophy (IFTA), among other characteristics as typical histological features of DN. This is further supported by the fact that STZ-induced diabetes may result to persistent hyperglycemia, characterized by elevated blood sugar levels [73]. In addition, prolonged high glucose levels can cause harm to both blood vessels and the small filtering units (glomeruli) within the kidneys [74]. The observed changes in the kidney’s cellular histo-architecture in this study might be attributed to the effect of the STZ-induced hyperglycemia as well as the effect of administration on all the test groups barring the distilled water administered to non-diabetic control rats. This may have resulted in distorted histo-architecture revealing mild to moderate renal cellular changes. Over time, this damage can progress to DN.
In conclusion, the present study revealed the drug potentials of EEMS and its biogenic SeNPs in the reversal of kidney malfunction caused by STZ-induced diabetes in vivo and measurements of kidney function indices. The ethanolic extract of M. myristica seed and SeNPs exhibited significant anti-diabetic activities and attenuated DN. The presence of compounds such as eucalyptol, scientifically designated as 1,3,3-trimethyl-2-oxabicyclo [2.2.2] octan-6-ol, α-phellandrene, germacradienol, and δ-cadinene could be deemed responsible for the protection of the kidney nephron. The results of this study confirm previous reports on the antidiabetic properties of M. myristica seed oil and its traditional use in therapy.
Abbreviations
b.wt: | body weight |
DM: | diabetes mellitus |
DMSO: | dimethyl sulfoxide |
DN: | diabetic nephropathy |
EEMS: | ethanolic extract of Monodora myristica seed |
FBS: | fasting blood glucose |
GFR: | glomerular filtration rate |
HR-TEM: | high-resolution transmission electron microscopy |
SeNPs: | selenium nanoparticles |
STZ: | streptozotocin |
UV-Vis: | Ultraviolet-visible |
ZnO NPs: | zinc oxide nanoparticles |
Declarations
Acknowledgments
We wish to acknowledge the Research facilities at Fulcrum Innovation Limited, Ilorin, Nigeria for supporting with bio-evaluations and assay.
Author contributions
BOY: Conceptualization, Formal analysis, Methodology, Resources, Writing—review & editing, Project administration. UO: Project administration, Writing—original draft, Software, Formal analysis, Data curation, Supervision, Validation. AOA: Formal analysis, Methodology, Resources, Writing—review & editing, Project administration, Writing—original draft, Software. IAM: Writing—original draft, Software, Formal analysis. ZAB: Writing—original draft, Investigation. HYL: Data curation, Supervision, Validation. OSO, LBA and MAA: Investigation, Visualization. RBI: Writing—original draft, Investigation. All authors read and approved the submitted version.
Conflicts of interest
The authors declare that they have no conflicts of interest.
Ethical approval
Ethical approval to conduct this research was obtained from Summit University Ethical Review Committee (SUERCA). The research was carried out after ethical approval from the departmental Ethical Committee on the Care and Use of Experimental Animals of Chemicals Sciences Department, Summit University, Offa Nigeria with approval number: SUERC/CONAS/2023/002 dated 26th June 2023.
Consent to participate
Not applicable.
Consent to publication
Not applicable.
Availability of data and materials
The raw data used in this study originated from the Department of Chemical Sciences, Summit University Offa, Kwara State, Nigeria while the derived data substantiating the findings can be obtained from the corresponding authors upon reasonable request.
Funding
The authors specifically recognize the financial support provided to B.O. Yusuf [PSTD2204274997] by the National Research Foundation of South Africa. Also, the partial financial support provided to A.O. Akinfenwa by Istabaraqim Nigeria Limited (RC 129541), and Prof. Nafiu Mikhail of Biochemistry department, University of Ilorin Nigeria for their contributions. The funders had no role in study design, data collection and analysis, decision to publish, or preparation of the manuscript.
Copyright
© The Author(s) 2025.
Publisher’s note
Open Exploration maintains a neutral stance on jurisdictional claims in published institutional affiliations and maps. All opinions expressed in this article are the personal views of the author(s) and do not represent the stance of the editorial team or the publisher.