Abstract
For the past 100 years, insulin supplementation has been the mainstay of treatment for type 1 diabetes (T1D), which is characterized by progressive autoimmune-mediated loss of insulin-producing β cells in the islets of Langerhans over the last decades, technological advances in glucose monitoring and therapeutics have greatly improved the care and management of these patients. However, morbidity, mortality, and quality of life remain challenges for patients with T1D. Islet transplantation has been successfully performed, but there are several limiting factors, such as the lack of cadaveric donors and the need for lifelong immunosuppressive therapy. Therefore, there is a great medical need for alternative therapeutic approaches. In the current review, the current knowledge on novel approaches for the treatment of T1D with a focus on the potential of using chimeric antigen receptor (CAR)-T cells and natural killer (NK) cells is summarized.
Keywords
Chimeric antigen receptor, natural killer cells, autoimmunity, type 1 diabetesIntroduction
The main function of the immune system is to protect the host from infection by microbes and parasites. To prevent immune responses against self-antigens, the immune system has central and peripheral mechanisms of tolerance, including regulatory T and B cells. However, disruption of this self-tolerance can lead to the immune system attacking healthy tissues or organs, resulting in autoimmune diseases. In autoimmune diseases, autoantibodies and/or autoreactive T cells recognize self-antigens presented by the major histocompatibility complex (MHC). Post-translational modifications of a peptide antigen can alter the MHC-binding motif or T cell receptor contact residues, converting self-tolerance into autoreactivity [1].
There are over 100 known autoimmune diseases, including rheumatoid arthritis, multiple sclerosis, systemic lupus erythematosus (SLE), and type 1 diabetes (T1D), which affect millions of people globally [2]. These diseases are chronic, debilitating, and occasionally fatal, and their development is the result of unknown interactions between environmental triggers and numerous polymorphic genetic elements [3]. B cells and T cells are the primary contributors to the overactive immune response involved in the pathogenesis of autoimmune diseases [4, 5].
In this review, the current and potential future strategies for the treatment of autoimmune T1D with a focus on the therapeutic potential of chimeric antigen receptor (CAR)-T cells and natural killer (NK) cells is discussed.
T1D
The International Diabetes Federation reported in 2021 that approximately 537 million adults worldwide are affected by diabetes mellitus. It is estimated that the number will increase to 643 million by 2030 and reach 783 million by 2045. In 5–10% of these patients, T1D is diagnosed, which is characterized by progressive loss of insulin-producing β cells in islets of Langerhans due to autoimmune activity involving CD4+ and CD8+ T cells triggered by impaired immune tolerance to β cell antigens [6]. Environmental factors, such as maternal diet, mode of delivery, infant feeding, childhood diet, microbial exposure, and use of anti-microbials, leading to changes in the gut microbiome, have emerged as major disease-driving factors in addition to genetic factors [7]. Viral infections, including enteroviruses and severe acute respiratory syndrome coronavirus 2 (SARS-CoV-2), are also thought to contribute to disease development [8, 9].
Patients with T1D can be identified through autoantibody profiling, with high-risk individuals having two or more autoantibodies against insulin, glutamate decarboxylase 65 (GAD65), islet antigen-2 (IA-2), and/or zinc transporter 8 (ZnT8) detectable in their serum [10]. The human leukocyte antigen (HLA) class II locus has been shown to have the strongest association with T1D among genetic risk factors [10]. Despite progress in identifying risk factors, the factors that influence disease progression remain largely unknown.
Treatment of T1D
In cases of severe autoimmune diseases, hormone substitution or medication may not be enough to effectively control the disease and provide optimal care for the patient [11, 12]. Insulin has been the preferred treatment for T1D for the past century, but despite mitigating the risk of diabetic ketoacidosis, patients still face challenges such as morbidity, mortality, and reduced quality of life. This is due to the absence of physiological and pulsatile glycemic control, as seen in native islets, caused by delays in glucose sensing and insulin absorption. Islet transplantation has been found to improve glucose instability and enhance the quality of life for T1D patients. Studies have shown that in non-diabetic patients, the quality of life and metabolic outcomes improved when pancreatectomy was performed alongside islet autotransplantation [13]. However, islet transplantation has limitations, such as the shortage of cadaver donors, the need for lifelong immunosuppressive therapy, and the decline of graft mass. Therefore, a promising alternative is the differentiation of human pluripotent stem cells (PSCs), either embryonic stem cells (ESCs) or induced pluripotent stem cells (iPSCs), into islet cells, which can be used for transplantation [12]. The use of PSCs offers an encouraging direction for personalized regenerative therapies, which can potentially provide a cure for the disease and resolve the tissue supply shortage issue [14].
Recent approaches using stem cell-derived β-like cells have gained approval from the Food and Drug Administration (FDA) for clinical trials. Nevertheless, stem-cell-based approaches have limitations such as the heterogeneity of cell types, immunogenicity, and in vivo survival. Autogenic transplantation has the potential to eliminate the requirement for lifelong immunosuppression [15]. Nonetheless, the use of autologous stem cell-derived β cells is still susceptible to attack by autoreactive T cells [6].
Immunotherapy
Immunotherapy differs from traditional immunosuppressive treatments by directly targeting affected cells. Its development has been rapid in recent decades, especially in oncology where it stimulates the immune system to recognize cancer cells and initiate an anti-tumor response. In the 1990s, B cell depletion was explored as a potential treatment for immune disorders. Depletion of B lymphocytes using the CD20 pan-B cell-specific monoclonal antibody rituximab has been found to offer some therapeutic benefits to patients with severe autoimmune diseases (Figure 1), such as diabetes [16], Graves’ orbitopathy [17], Addison’s disease [18], and pemphigus vulgaris [19, 20].
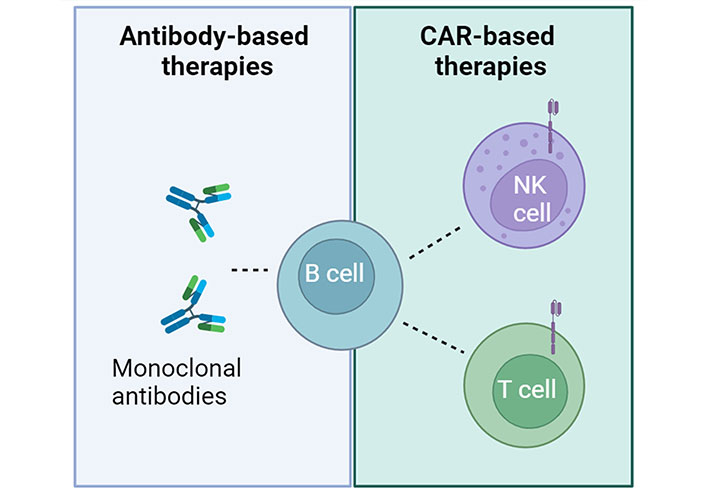
B cell depletion in autoimmune diseases. Two types of immunotherapies, monoclonal antibodies and CAR-based approaches, both targeting B cells, have the potential to be used in clinical applications to treat autoimmune diseases. Created with BioRender.com
In recent years, researchers have observed substantial advancements in the development of immune cell-based therapies for cancer treatment. Primary T cells armed with CARs have demonstrated remarkable efficacy, particularly in patients with acute lymphoblastic leukemia (ALL) and lymphoma. The potential of CAR-T cell therapy in oncology could be extended to the treatment of infectious and autoimmune diseases (Figure 1).
CAR-T cell therapy
CARs are genetically engineered receptors that are capable of recognizing specific antigens and activating downstream signaling pathways. Similar to rituximab, CAR-T cells that target the pan-B cell marker CD19 can effectively deplete all B lymphocytes. This approach has been successful in treating hematological malignancies, and currently, six CAR-T cell products that target CD19 or B cell maturation antigen (BCMA) are FDA-approved for the treatment of B cell leukemia, lymphoma, and multiple myeloma (Table 1).
FDA-approved CAR-T cell therapies
Name | Target antigen | Disease | Year of approval |
---|---|---|---|
Tisagenlecleucel (Kymriah) | CD19 | B-ALL, NHL | 2017 |
Axicabtagene ciloleucel (Yescarta) | CD19 | NHL, follicular lymphoma | 2017 |
Brexucabtagene autoleucel (Tecartus) | CD19 | MCL, B-ALL | 2021 |
Lisocabtagene maraleucel (Breyanzi) | CD19 | NHL | 2021 |
Idecabtagene vicleucel (Abecma) | BCMA | Multiple myeloma | 2021 |
Ciltacabtagene autoleucel (Carvykti) | BCMA | Multiple myeloma | 2022 |
B-ALL: B cell acute lymphoblastic leukemia; NHL: non-Hodgkin lymphoma; MCL: mantle cell lymphoma
The CAR technology can also be used for other indications, including autoimmunity. The first clinical trial investigating autologous CAR-T cells directed against BCMA as a therapeutic strategy for severe autoimmune diseases is underway. A phase I/II clinical trial at the University of Miami is testing autologous CAR-T cells directed against BCMA in patients with generalized myasthenia gravis (NCT04146051). Additionally, a group at the University of Erlangen in Germany demonstrated the successful treatment of five patients with SLE and one patient with refractory antisynthetase syndrome using CD19 CAR-T cells [21–23].
However, CAR-T cell therapy for cancer patients targeting CD19 has been associated with serious side effects such as cytokine release syndrome (CRS) and neurotoxicity. When considering the risk/benefit analysis of using CAR-T cells in autoimmunity, a stricter evaluation is necessary than in malignant diseases where higher therapeutic risks may be acceptable. Furthermore, CAR-T cells recognize allogeneic cells via MHC molecules, and therefore so far, CAR-T cells have only been generated from autologous T cells. The production of autologous CAR-T cells requires individual production with enormous manufacturing costs, product heterogeneity, and longer production times, resulting in limited accessibility for most patients [24]. In addition, CAR-T cells can expand uncontrollably in a patient, and engrafting of CAR-T cells could lead to permanent depletion of all B cells, causing immunodeficiency that requires the lifelong substitution of immunoglobulins.
CAR-NK therapy
Concerns about side effects such as CRS have led researchers to explore alternative vehicles for CARs. NK cells have emerged as a promising alternative due to their ability to eliminate target cells without the risk of CRS, as they do not secrete interleukin-6. Furthermore, NK cells are not MHC-restricted, allowing allogeneic use and potentially creating an “off-the-shelf” product. Various sources of NK cells, such as peripheral and umbilical cord blood, as well as those derived from hematopoietic and iPSCs, have been developed for cancer therapy [25–27]. Genetic modification of NK cells with CARs has shown preclinical efficacy against several cancers [25, 28–30]. Primary CAR-NK cells targeting CD19 have demonstrated promising efficacy and safety in the treatment of hematological malignancies [31]. Alternatively, stable NK cell lines such as NK-92 have been established for clinical purposes and have demonstrated safety and clinical response in some cancers [32–35].
Unlike T cells, NK cells have a limited lifespan in vivo and do not expand, which allows repeated administration without the risk of lifelong immunosuppression [36]. However, the current limitation of primary CAR-NK cells is the difficult genetic modification and limited ex vivo expansion to obtain sufficient amounts of cells for multiple doses. Improvements in gene transfer and ex vivo expansion protocols may overcome these issues (Figure 2). Alternatively, NK cell lines or iPSCs-derived NK cells could be used as an unlimited scalable source of well-defined engineered NK cells, but their use for the treatment of autoimmune diseases needs to be tested.
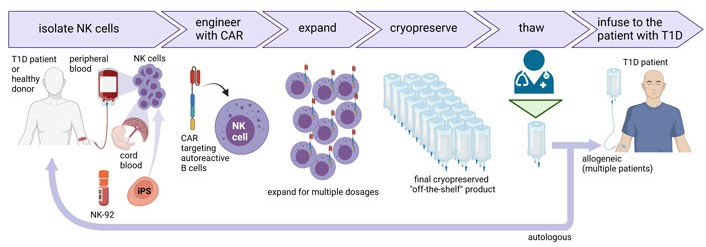
Production of CAR-NK cells for the treatment of T1D. NK cells can be isolated from the peripheral blood of the T1D patient or a healthy donor, or cord blood. NK cells can also be differentiated from iPSCs or NK cell lines such as NK-92 can be used. NK cells are further engineered with CAR under good manufacturing practice (GMP) conditions followed by cell expansion to obtain multiple doses of the CAR-NK cell product that can be cryopreserved and stored as an “off-the-shelf” product. CAR-NK cells can be thawed on demand by medical staff and infused in patients suffering from diabetes or other autoimmune diseases. The universal nature of NK cells allows them to be used for multiple patients. Created with BioRender.com
Preclinical models
To test such CAR-NK cells for the treatment of autoimmune diseases, preclinical models are essential. Since the CAR-T and CAR-NK cells developed for patient treatment are of human origin, immunodeficient animal models must be used to prevent the CAR-T or CAR-NK cells from being rejected by the murine immune system. To study in vivo elimination of human autoreactive immune cells by CAR-T and CAR-NK, these mice must be further humanized. Several humanized mouse models have been developed for SLE [37], for example by transferring human peripheral blood mononuclear cells or peripheral blood lymphocytes from SLE patients to immunodeficient mice. Another approach is to transfer human hematopoietic stem cells to immunodeficient mice and then induce lupus with pristane. Lastly, the use of human tissue slice cultures in biomimetic microfluidic systems could also be a relevant alternative for animal testing. Human tissue cultures of pulmonary and cardiac tissue have successfully been established and used to study basic disease mechanisms as well as therapeutic approaches [38]. The use of CAR-T or CAR-NK cells in these models could be a promising pre-clinical human model. The continuous development of improved preclinical models for autoimmune diseases will ultimately facilitate the translation of CAR-NK and T cell therapy to patients.
Conclusion
The incidence of T1D is increasing worldwide and current treatments are not optimal for all patients. CAR-T cells and CAR-NK cells have been successfully used in the treatment of cancer. Early preclinical and clinical data suggest that CAR-T cells may be used to treat certain autoimmune diseases, but efficacy in the treatment of T1D remains to be demonstrated. CAR-NK cell technology is developing rapidly, and further research is needed to determine whether it will outperform CAR-T cells in terms of safety while achieving sufficient efficacy for the treatment of autoimmune diseases such as T1D.
Abbreviations
BCMA: | B cell maturation antigen |
CAR: | chimeric antigen receptor |
CRS: | cytokine release syndrome |
FDA: | Food and Drug Administration |
iPSCs: | induced pluripotent stem cells |
MHC: | major histocompatibility complex |
NK: | natural killer |
SLE: | systemic lupus erythematosus |
T1D: | type 1 diabetes |
Declarations
Author contributions
CS: Writing—original draft, Writing—review & editing. JE, ITO, MA, SRK, TT: Writing—review & editing. SRB: Supervision. All authors read and approved the submitted version.
Conflicts of interest
The authors declare that they have no conflicts of interest.
Ethical approval
Not applicable.
Consent to participate
Not applicable.
Consent to publication
Not applicable.
Availability of data and materials
Not applicable.
Funding
This study was supported by the German Federal Ministry of Education [Clusters4Future SaxoCell, 03ZU1111DA]. The funder had no role in study design, data collection and analysis, decision to publish, or preparation of the manuscript.
Copyright
© The Author(s) 2024.