Abstract
Metabolic dysfunction-associated steatotic liver disease (MASLD) is rapidly emerging as a global health crisis, affecting over 30% of the population and demanding urgent attention. This redefined condition, previously known as non-alcoholic fatty liver disease (NAFLD), reflects a deeper understanding of the intricate interplay between metabolic dysfunction and liver health. At the heart of MASLD lies the troubling accumulation of triglycerides (TGs) in hepatocytes, which precipitates insulin resistance and oxidative stress, ultimately leading to more severe forms like metabolic dysfunction-associated steatohepatitis (MASH). Excitingly, recent research has spotlighted the farnesoid X receptor (FXR) as a groundbreaking therapeutic target. FXR not only regulates lipid metabolism but also combats inflammation and insulin resistance, making it a potential game-changer in the fight against MASLD. With only one FDA-approved drug, resmetirom, currently available, the exploration of FXR agonists opens new avenues for innovative treatments that could revolutionize patient care. By harnessing the power of FXR to restore metabolic balance and integrating advanced strategies like lipidomics and fatty acid profiling, we stand on the brink of transforming how we approach MASLD and its associated complications, paving the way for a healthier future. This review delves into the promising role of FXR in combating MASLD and its implications for related metabolic disorders, emphasizing the urgency for advanced strategies to detect and manage this burgeoning epidemic.
Keywords
Metabolic dysfunction-associated steatotic liver disease (MASLD), metabolic dysfunction-associated steatohepatitis (MASH), non-alcoholic fatty liver disease (NAFLD), non-alcoholic steatohepatitis (NASH), farnesoid X receptor (FXR), fatty acids, analytical techniques, lipid quantificationIntroduction
The landscape of liver disease nomenclature has recently undergone a significant transformation, igniting curiosity and discussion among healthcare professionals and researchers alike. The shift from non-alcoholic fatty liver disease (NAFLD) to metabolic dysfunction-associated steatotic liver disease (MASLD), along with the transition from non-alcoholic steatohepatitis (NASH) to metabolic dysfunction-associated steatohepatitis (MASH), reflects an evolving scientific understanding that emphasises the metabolic dysfunction underlying these conditions. This rebranding highlights that the “M” in MASLD and MASH signifies metabolic dysfunction, inherently linking these diseases to prevalent metabolic disorders such as obesity and type 2 diabetes, which are critical contributors to liver injury [1, 2]. The reformulation of these terms was guided by a comprehensive Delphi consensus process involving multiple stakeholders in hepatology, aimed at establishing a more accurate and clinically relevant framework for diagnosis and treatment [3, 4]. Currently, the global prevalence of MASLD is estimated at approximately 32%, with projections suggesting it could rise to 55.4% by 2040 due to increasing rates of obesity and type 2 diabetes [5–7]. In India, the prevalence stands at around 38% among adults, indicating a significant public health concern as the nation faces rising obesity rates and associated metabolic disorders [6, 8–10]. Regions such as Latin America report even higher prevalence rates, reaching 44.4%, underscoring the urgent need for targeted interventions globally [6, 11–13].
Despite advancements in nomenclature and understanding, the pathogenesis of MASLD remains incompletely elucidated, with steatosis defined as the presence of triglycerides (TGs) in hepatocytes serving as the primary precursor for the disease [14–17]. The development of hepatic steatosis arises from an imbalance between lipid intake and lipid removal, which is regulated by four key mechanisms: fatty acid uptake, de novo lipogenesis (DNL), fatty acid oxidation, and lipid export. These mechanisms play a crucial role in both the physiological and pathological progression of MASLD [11, 18–20].
Targeting farnesoid X receptor (FXR) presents a promising therapeutic strategy for managing MASLD because FXR is integral in regulating these four key mechanisms of hepatic lipid metabolism [21]. By selectively modulating FXR activity through agonist ligands, it can effectively control lipid acquisition (fatty acid acquisition) and lipid removal to mitigate hepatic steatosis and its progression to more severe liver conditions [22, 23]. Furthermore, FXR agonists have demonstrated significant histological improvements in clinical trials for liver diseases, indicating their potential to enhance treatment outcomes for patients with MASLD [24–29]. This approach not only targets the underlying metabolic dysfunction but also opens avenues for combination therapies that may improve efficacy while minimising side effects associated with current treatments [30].
As research progresses, exploring FXR’s role in MASLD will be crucial for developing innovative therapeutic strategies to effectively manage this complex disease and its associated metabolic disorders [31]. This review explores the role of FXR in liver physiology and the progression of MASLD. We begin by examining FXR’s normal functions and how its dysregulation contributes to disease pathology. We then identify specific lipid classes that alter during the shift from health to disease, highlighting their significance as diagnostic markers and potential biomarkers. Following this, we discuss treatment strategies using FXR agonists and ongoing clinical trials evaluating their effectiveness in managing MASLD. Additionally, we emphasize the crucial roles of fatty acids and lipid classes in the pathogenesis of MASLD and present lipid analyses conducted with hyphenated techniques to reinforce our findings on relevant biomarkers. To ensure clarity and consistency, we will refer to the disease as MASLD and MASH, regardless of whether the underlying populations in the referenced studies were originally classified as patients with NAFLD or NASH.
Association of MASLD with features of the metabolic syndrome
MASLD condition is characterized by fat deposition in the liver, which can lead to inflammation and fibrosis, ultimately resulting in more severe liver disease, MASH [32–34]. The relationship between MASLD and metabolic syndrome is mutual and bi-directional, highlighting the complexity of these interconnected conditions [35, 36].
Mutual association with metabolic syndrome
MASLD is frequently associated with several components of metabolic syndrome, including obesity, type 2 diabetes mellitus (T2DM), dyslipidaemia, and hypertension. These conditions collectively contribute to the development and progression of MASLD [37–39]. For instance, individuals with obesity often exhibit increased hepatic fat accumulation, which can exacerbate insulin resistance and lead to T2DM [40–42]. Conversely, the presence of MASLD can worsen metabolic syndrome features; for example, hepatic steatosis can lead to increased insulin resistance, further complicating glucose metabolism and lipid profiles [43–45]. Research shows that a substantial number of individuals, including those who are lean, are affected by MASLD, with about 25% of lean individuals living with HIV also exhibiting the condition, indicating that metabolic dysfunction is not exclusively associated with obesity [6, 37, 46]. These statements emphasize the importance of recognizing MASLD in diverse populations and its potential to influence metabolic health across different demographic groups.
Bi-directional relationship
The relationship between MASLD and metabolic syndrome is bi-directional [47, 48]. while metabolic syndrome increases the risk of developing MASLD, existing liver disease can also exacerbate features of metabolic syndrome [32, 49, 50]. For example, elevated levels of free fatty acids (FFAs) (for example, oleic acid, palmitic acid, stearic acid, and linoleic acid, etc.) in individuals with MASLD can contribute to systemic insulin resistance and dyslipidaemia by promoting lipogenesis and inhibiting fatty acid oxidation in peripheral tissues [51–53]. Furthermore, the buildup of lipids in the liver can lead to an increased the production of pro-inflammatory cytokines [for example, interleukin (IL)-1, IL-6, IL-12, IL-17, etc.], which may worsen insulin sensitivity and promote cardiovascular risk factors associated with metabolic syndrome [54, 55].
Role of fatty acids
Fatty acid plays a crucial role in intricate relationships. They are central to understanding the pathophysiology of MASLD. The disease is characterized by alterations in hepatic and plasma lipid balance, which are critical for diagnosing and assessing its severity [56]. Hepatic insulin resistance, a key feature of MASLD, correlates with increased levels of diacylglycerol (DAG) and triacylglycerol (TAG), as identified in lipidomics studies [57]. Elevated circulating FFAs may significantly contribute to hepatic lipotoxicity, leading to liver inflammation and fibrosis [32, 58, 59] particularly associated with saturated fatty acids (SFA) such as palmitic acid [60]. In contrast, unsaturated fatty acids (UFA) have been observed to provide protective effects against liver damage and can enhance insulin sensitivity [61–64]. The detrimental impact of SFA on liver health is evident through their accumulation in hepatocytes, which can induce apoptosis and lipotoxicity. Factors influencing fatty acid levels include diet, age, gender and hepatic DNL [65]. Studies indicate that excessive fatty acid accumulation can lead to endoplasmic reticulum stress and hepatocyte injury [66]. The balance between different types of fatty acids is crucial for both the progression of MASLD and the general metabolic health of individuals [64, 67]. Specific lipid profiles have emerged as potential biomarkers for MASLD diagnosis; during the condition, there is an increase in SFA like myristic and palmitic acids [68], while alterations in TG levels and certain phospholipid species may reflect disease progression or treatment response [69–71].
In liver samples from patients with MASLD, there is an observed increase in monounsaturated fatty acids (MUFA) alongside elevated DAG and TAG levels [72, 73]. Inversely, polyunsaturated fatty acids (PUFAs) such as eicosapentaenoic acid (EPA) and docosahexaenoic acid (DHA) tend to decrease in MASLD conditions [74, 75]. Additionally, activation of FXR has been shown to reduce hepatic lipid levels and intestinal lipid absorption, highlighting potential therapeutic pathways for managing MASLD [76]. Overall, understanding the intricate relationship between various fatty acids and their metabolic implications is essential for addressing MASLD effectively. Fatty acids represent a class of lipid profiles, and various alterations in lipid composition associated with different metabolic disorders are detailed in Table 1.
Alteration of lipids in different metabolic disorders
Condition | Lipid alterations |
---|---|
Obesity | Obesity is often associated with dyslipidaemia, which presents as elevated plasma levels of TGs, VLDL, and low HDL values. Every 10 pounds of extra fat increases daily cholesterol production by 10 mg. The lipid profile rises in obese individuals [169]. |
MASLD | Hepatic lipid imbalance results from hepatic absorption of FAs and de novo lipogenesis exceeding fatty acid oxidation and lipid export in MASLD. This leads to modifications in the hepatic metabolism of lipids, potentially precipitating severe complications such as cirrhosis and HCC. Different MASLD patients had various levels of serum lysophosphatidylcholine, sphingomyelin, total cholesterol esters, and TAGs [170]. |
Type II diabetes | Anomalies of plasma lipids and lipoprotein associated with type II diabetes include reduced HDL cholesterol, an abundance of small dense LDL particles, and elevated TG levels. Every one of these dyslipidaemia traits is linked to an increased risk of CVD [171]. |
Type II diabetes with MASLD | There is a bidirectional pathophysiological link between MASLD and type 2 diabetes. On the one hand, the latter encourages the development of MASL into MASH, a more advanced form of the disease. HCC and cirrhosis are two conditions that are made more likely by NASH and may necessitate liver transplantation [172]. |
MASLD + obesity + type II diabetes | The risk of developing cirrhosis and MASH is significantly increased when type 2 diabetes, obesity, and MASLD are present than when MASLD is present, but chronic hyperglycaemia is not. Whether MASLD is a substantial independent predictor of CVD remains debatable [173]. |
TGs: triglycerides; VLDL: very low-density lipoprotein; HDL: high-density lipoprotein; MASLD: metabolic dysfunction-associated steatotic liver disease; FAs: fatty acids; HCC: hepatocellular carcinoma; TAGs: triacylglycerols; LDL: low-density lipoprotein; CVD: cardiovascular disease; MASL: metabolic dysfunction-associated steatotic liver; MASH: metabolic dysfunction-associated steatohepatitis; NASH: non-alcoholic steatohepatitis
Physiological role of FXR
FXR is a key nuclear receptor that acts as a bile acid sensor, playing a significant role in maintaining metabolic homeostasis [77–79]. Predominantly found in the liver and intestine, FXR integrates metabolic signals and is activated by bile acids such as, which are crucial for cholesterol and lipid metabolism [77, 80, 81]. Upon activation, FXR heterodimerizes with retinoid X receptor (RXR) and binds to FXR response elements (FXREs) to regulate genes involved in bile acid synthesis and metabolism [82, 83]. It’s essential for coordinating metabolic processes and acts as a therapeutic target for metabolic disorders [31, 84–86]. FXR is an essential modulator of lipid metabolism [87, 88]. Exerting its effects by modulating the activity of several transcription factors, including sterol regulatory element-binding protein-1c (SREBP-1c) and carbohydrate response element-binding protein (ChREBP). SREBP-1c is crucial for the regulation of genes involved in fatty acid and TG synthesis, and FXR activation leads to the repression of SREBP-1c expression. This inhibition is significant as it reduces hepatic TG levels and helps prevent hepatic steatosis, particularly in MASLD [89, 90]. Additionally, FXR interacts with ChREBP to fine-tune lipogenesis based on glucose availability, ensuring lipid synthesis is aligned with the body’s energy status [91, 92]. The activation of FXR decreases the expression of fatty acid synthase (FASN), an enzyme essential for fatty acid biosynthesis, and regulates other pathways critical for lipid homeostasis [93, 94]. The small heterodimer partner (SHP) is another important component in this regulatory network. Upon activation by bile acids, FXR induces SHP expression, which acts as a transcriptional repressor of various target genes involved in lipogenesis and bile acid synthesis [95]. This mechanism helps to prevent excessive TG accumulation and maintain normal lipid profiles within hepatocytes [96, 97]. Furthermore, the FXR-SHP pathway interacts with other nuclear receptors such as peroxisome proliferator-activated receptor alpha (PPARα), enhancing its regulatory capacity on lipid metabolism [90, 98]. Comprehensive, through the modulation of SREBP-1c and ChREBP, along with the involvement of SHP, FXR plays a pivotal role in coordinating lipid metabolism [99, 100]. Xu et al. [101] studied the impact of the activation of the FXR by obeticholic acid (OCA) significantly inhibits hepatic cholesterol 7α-hydroxylase (CYP7A1) and sterol 12α-hydroxylase (Cyp8b1), partly through the induction of SHP. This inhibition leads to a reduced bile acid pool size and altered bile acid composition, which contributes to decreased intestinal cholesterol absorption and enhanced macrophage reverse cholesterol transport (RCT). The reduction in hepatic microsomal cholesterol content triggers an elevation in the expression and functionality of low-density lipoprotein receptors (LDL-R) within the liver [102]. Consequently, this cascade of events leads to a decrease in plasma LDL-cholesterol (LDL-C) levels [103]. In addition to inhibiting CYP7A1, enhanced excretion into bile occurs via the cholesterol transporters known as adenosine triphosphate-binding cassette transporters (ABCG5/8), which can elevate blood cholesterol levels in knockout (KO) mice [104]. Reduction in intestinal bile acids and promotion of transintestinal cholesterol excretion serve as mechanisms to lower cholesterol levels in the intestine [105]. While FXR induces an anti-atherogenic effect in mice, the scenario differs in humans. This was possible in mice due to the presence of enzymes Cyp2c70 (cytochrome P450 2C70) and Cyp2a12 (cytochrome P450 2A12) [106–108].
FXR-induced lipoprotein metabolism is complex and differs mainly in ligands and species. FXR is known to reduce high-density lipoprotein (HDL) cholesterol, resulting in a pro-atherogenic profile. The proatherogenic effect is due to the stimulating effects of cholesterol ester transfer protein (CETP), which increases LDL and decreases HDL cholesterol [109]. In addition, the transformation of very low-density lipoprotein (VLDL) rich in TG is converted to cholesterol-rich LDL particles [110]. The major difference between the normal liver and disease progression.
Pathophysiology of FXR in MASLD and its related disorders
The mechanism behind MASLD primarily involves insulin resistance and lipotoxicity. Insulin resistance leads to increased FFA release from adipose tissue, which accumulates in the liver, causing hepatic steatosis as mentioned in Figure 1 [34, 51]. This accumulation triggers oxidative stress and inflammation, further exacerbated by elevated levels of reactive oxygen species (ROS) that activate pro-inflammatory pathways [111]. Increased ROS levels stimulate kinases such as c-Jun N-terminal kinase (JNK) and p38 mitogen-activated protein kinases (MAPK), promoting inflammation and disrupting insulin signalling. This results in a vicious cycle where inflammation worsens insulin resistance [112]. Additionally, dietary factors like high fructose and saturated fat intake enhance DNL and contribute to the progression from simple steatosis to steatohepatitis. The interplay between gut microbiota dysbiosis and metabolic perturbations also fuels hepatic inflammation and injury [113]. Understanding these specific mechanisms is crucial for developing targeted therapies for MASLD [114], as illustrated by the alterations in disease identifiers depicted in Figure 2.
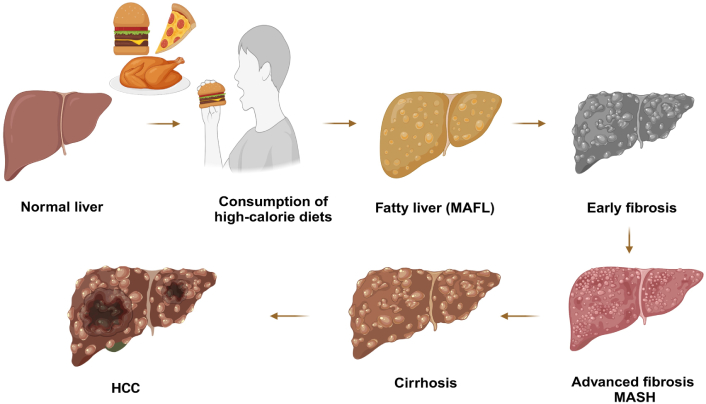
Progression from normal liver to cirrhosis and HCC Induced by high-calorie diet. This visual representation underscores the importance of dietary management in preventing liver-related diseases and emphasizes the cascading effects of excessive calorie intake on liver function. MAFL: metabolic dysfunction-associated fatty liver; MASH: metabolic dysfunction-associated steatohepatitis; HCC: hepatocellular carcinoma. Created in BioRender. Pirangi, S. (2025) BioRender.com/z08d915
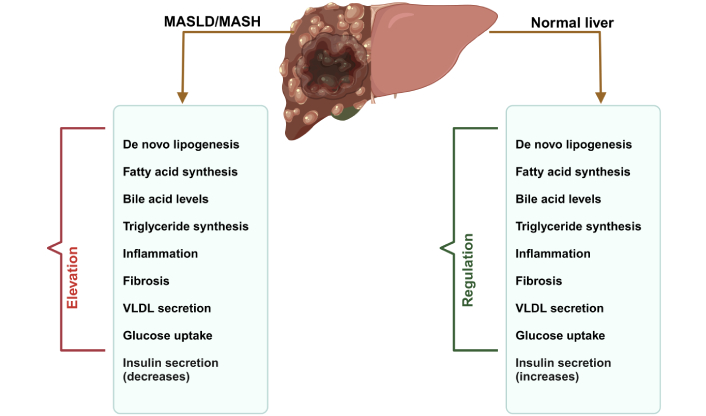
Comparative analysis of liver health: normal vs. MASLD/MASH. This visual emphasizes the critical role of lipogenic gene regulation in the transition from healthy liver function to advanced liver disease, underscoring the importance of dietary choices in preventing metabolic liver disorders. Due to the regulation of lipogenic genes (SREBP-1c, ChREBP, FASN, and SHP) regulated in normal and elevated in MASLD/MASH. MASLD: metabolic dysfunction-associated steatotic liver disease; MASH: metabolic dysfunction-associated steatohepatitis; VLDL: very low-density lipoprotein; SREBP-1c: sterol regulatory element-binding protein-1c; ChREBP: carbohydrate response element-binding protein; FASN: fatty acid synthase; SHP: small heterodimer partner. Created in BioRender. Pirangi, S. (2025) BioRender.com/x79m262
Impaired lipid regulation
MASLD to MASH represents a significant shift in liver pathology, akin to a small canal (MASLD) flowing into a vast river (MASH). MASLD is characterized by hepatic steatosis, where fat accumulation exceeds 5% of liver weight, often associated with metabolic syndrome features such as obesity, insulin resistance and dyslipidaemia. This stage primarily involves fat accumulation without significant inflammation or fibrosis. In contrast, MASH is a more advanced condition that includes inflammation (steatohepatitis) and can progress to fibrosis, cirrhosis, and even hepatocellular carcinoma (HCC) in Figure 1. MASH is marked by hepatocyte ballooning, inflammatory cell infiltration, and varying degrees of fibrosis. Patients with MASLD may experience stable disease or progress to MASH over time. Few studies indicate that approximately one-third of patients with MASLD develop progressive fibrosis, while others may remain stable or even experience regression. The risk of severe complications increases significantly with advancing fibrosis stages, underscoring the importance of early detection and intervention. Understanding the mechanisms driving this progression is crucial for developing effective diagnostic and therapeutic strategies to manage these prevalent liver diseases effectively. MASLD is closely linked to lipid metabolism, particularly TG and cholesterol metabolism. Increased fatty acid uptake and synthesis, along with decreased lipid degradation, significantly contribute to MASLD. Key transport proteins, including fatty acid transport proteins (FATPs), cluster of differentiation 36 (CD36), and caveolin-1 (CAV-1), facilitate fatty acid uptake in the liver. FATP2 and FATP5 are primarily expressed in the liver, with downregulation of FATP2 reducing fatty acid uptake and improving hepatic steatosis. CD36 is crucial for fatty acid uptake; inhibiting its palmitoylation can alleviate metabolic disorders. CAV-1 also plays a role by promoting autophagy and reducing lipid accumulation. Additionally, lipogenic markers like FASN and stearoyl-CoA desaturase (SCD) are upregulated in MASLD [115]. Targeting FASN through mechanisms such as sorting nexin 8-mediated degradation offers a promising strategy for MASLD prevention. FXR agonists, including nidufexor (LMB763), cilofexor, and EDP-305, are currently undergoing clinical trials for the treatment of MASLD. These compounds have shown promise in addressing key aspects of the disease. For instance, 1-adamantyl carbonyl-4-phenylpiperazine is an FXR agonist whose derivative, compound 10A, has demonstrated greater efficacy in ameliorating hyperlipidaemia, hepatic steatosis, and insulin resistance [21, 33, 115, 116].
Insulin resistance
The accumulation of TGs in the liver is closely linked to insulin resistance, a hallmark of metabolic syndrome and MASLD [117]. IR is defined as a reduced response to insulin signalling, particularly in insulin-sensitive tissues like adipose tissue and the liver. This impairment leads to decreased glucose uptake and promotes lipolysis, resulting in elevated FFAs that accumulate in the liver and cause fat overload in hepatocytes [118]. The insulin receptor pathway regulates hepatic lipid metabolism through the transcription factor SREBP-1c, which upregulates genes involved in fatty acid biosynthesis, such as acetyl-CoA carboxylase (ACC) and FAS. MASLD-related hepatic IR, inhibition of the IR pathway leads to unregulated DNL, while gluconeogenesis remains unaffected [119].
Inflammation and fibrosis
Chronic inflammation is another critical factor in the progression from simple steatosis to more severe forms of liver disease such as MASH and fibrosis [120]. FXR demonstrates anti-inflammatory effects by inhibiting the nuclear factor-kappa B (NF-κB) signalling pathways that play a role in inflammatory responses [121]. In conditions where FXR signalling is disrupted, there is an increase in pro-inflammatory cytokines and chemokines that promote inflammation and activate hepatic stellate cells (HSCs), leading to excessive extracellular matrix deposition and fibrosis [122]. The fibroblast growth factor (FGF)-15/19 axis is upregulated by FXR in enterocytes, with FGF-19 acting as an enterokinase that travels to the liver via the portal circulation. Upon reaching the liver, FGF-19 interacts with the FGF receptor 4 and β-klotho, leading to the repression of bile acid synthesis and gluconeogenesis. This regulation may support liver regeneration following injury. FXR agonists have demonstrated the ability to reduce fibrosis and steatosis while also exhibiting anti-inflammatory effects. In mice fed a methionine-choline-deficient (MCD) diet, the FXR agonist WAY-362450 effectively reduced liver inflammation and fibrogenesis without leading to an increase in TG levels [123, 124]. In reverse, impaired FXR function can exacerbate inflammatory responses, leading to progressive liver damage [122, 123].
Hepatic steatosis
The buildup of fat in the liver due to impaired FXR signalling not only contributes to steatosis but also increases the risk of developing HCC [125]. Studies have indicated that mice lacking functional FXR develop hepatocellular adenomas and carcinomas spontaneously, suggesting that FXR may act as a tumour suppressor in the liver [126]. By regulating lipid metabolism and inflammatory responses, FXR helps maintain a balance that prevents tumorigenesis [127, 128].
HCC
Recent studies have indicated that FXR may play a protective role against the development of HCC [84, 127]. HCC arises from metabolic dysregulation and chronic inflammation, often driven by increased IL-6 signalling, which plays a leading role in cancer development and progression. FXR has emerged as a significant therapeutic target in HCC, with its agonists, such as OCA, demonstrating tumour-suppressive effects. OCA inhibits the IL-6/Jak-2/STAT3 signalling pathway, thereby reducing STAT3 activation and increasing levels of suppressor of cytokine signalling 3 (SOCS3), a feedback inhibitor of STAT3 [129]. This modulation not only mitigates inflammation but also inhibits HCC proliferation, migration, and invasion, underscoring the potential of FXR agonists in developing effective treatment strategies for HCC [125]. The modulation of lipid metabolism by FXR may help mitigate the risk factors associated with HCC development [84].
Extra-hepatic manifestations: impact on chronic kidney disease
Emerging evidence suggests that FXR also plays a role in extra-hepatic manifestations of MASLD, particularly chronic kidney disease (CKD) [130]. The interplay between liver dysfunction and kidney health is complex; metabolic abnormalities stemming from hepatic steatosis can adversely affect renal function [131]. Elevated levels of circulating FFAs due to hepatic steatosis can lead to renal lipotoxicity, contributing to CKD progression [132]. FXR activation has been shown to exert protective effects on renal tissues by modulating lipid metabolism and reducing oxidative stress [133]. By regulating lipid uptake and metabolism in renal cells, FXR may help prevent renal injury associated with metabolic disorders [132]. Furthermore, FXR agonists have demonstrated potential in mitigating inflammatory responses within the kidneys, thereby offering a therapeutic avenue for addressing CKD related to MASLD [134].
Therapeutic applications of FXR agonists
FXR has emerged as a potential therapeutic target for the management of metabolic syndrome and liver disorders, particularly MASLD. The therapeutic potential of FXR agonists is being explored to mitigate the adverse effects associated with MASLD, MASH, and other metabolic disorders, as clearly discussed in Figure 3 [135]. The ligands for the FXR receptor can be classified based on their chemical nature: natural agonists [chenodeoxy cholic acid (CDCA), cholic acid, ursodeoxycholic acid (UDCA)], semisynthetic bile acids (OCA), and synthetic non-steroidal agonists (GW-4064 and WAY 362450) [136]. FXR agonists, such as OCA, have proven effective in reducing hepatic steatosis, enhancing insulin sensitivity, and providing anti-inflammatory effects in clinical trials. FXR agonists, such as OCA, have proven effective in reducing hepatic steatosis, enhancing insulin sensitivity, and providing anti-inflammatory effects in clinical trials. These agents work by restoring normal FXR signalling pathways that regulate lipid metabolism and inflammation [123]. Deoxycholic acid (DCA) and lithocholic acid (LCA) can activate FXR, albeit with lower efficiency. UDCA is a partial agonist inhibiting CDCA activity [137, 138]. FXR agonists have been used recently for the improvement of ailments like MASH, diabetes mellitus, and primary biliary cholangitis (PBC) [139]. OCA, developed by Intercept Pharmaceuticals, is one of the earlier FXR agonists, and its regenerate phase III trial demonstrated reproducibility with phase II data. However, the FDA denied approval for its use in MASH and has currently banned it from phase III clinical trials due to severe side effects, including pruritus; aside from pruritus, OCA was generally well-tolerated [140]. Notably, there was a reduction in HDL cholesterol levels. The U.S. FDA approved OCA for second-line treatment of PBC in 2016 [123, 141].
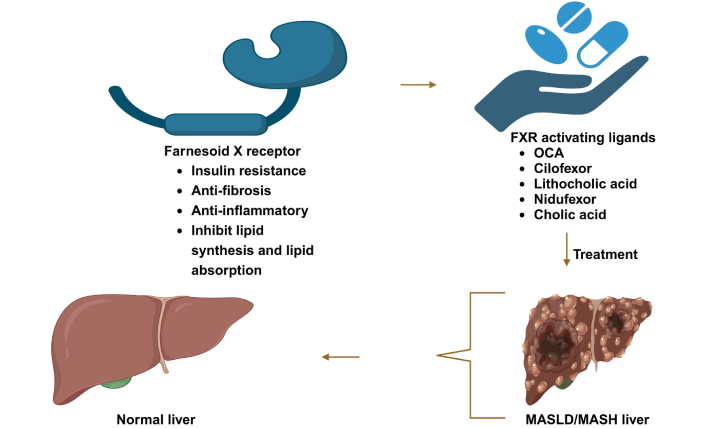
The role of FXR agonists in restoring liver health. This visual emphasizes the critical role of FXR agonists as promising therapeutic agents in managing liver diseases, showcasing their ability to restore liver health and improve overall metabolic function. OCA: obeticholic acid; MASLD: metabolic dysfunction-associated steatotic liver disease; NASH: non-alcoholic steatohepatitis. Created in BioRender. Pirangi, S. (2025) BioRender.com/u29l065
Clinical data of patients and rodent studies using UDCA showed that the benefits were more effective than clofibrate ones. Small trials showed elevated levels of enzymes, which generally show an increase during liver diseases (alanine aminotransferase, aspartate aminotransferase, gamma-glutamyl transferase, and alkaline phosphatases). These are reduced in the treatment [142]. However, norUDCA, a shortened UDCA derivative, gave promising results in phase II dosing trials in mice models [143]. MET-409, a non-steroidal FXR agonist currently in phase IIa clinical trial, is different in structure from bile acids and has an entirely different chemical nature from other FXR agonists (tropifexor and cilofexor) [144]. The agonist showed potent liver fat reduction, but a transient increase in alanine aminotransferase and FGF-19 was observed [145]. The regulation of fatty acid influx into the hepatic plasma membrane is governed by specialized transporters such as FATP, CD36, and caveolins [146, 147]. Depletion of FATP2, a specific isoform of FATP, has been demonstrated to ameliorate hepatic steatosis and diminish the uptake of fatty acids, suggesting a potential therapeutic avenue for mitigating excessive lipid accumulation in the liver [148]. The transportation of elongated fatty acids is facilitated by CD36, a mechanism regulated by PPAR-gamma [149]. There exists a clear association between the expression of CD36 and the concentration of TGs within hepatic tissue [150]. Investigations carried out in obese murine models propose that administration of GW4064 induces a subsequent decline in the expression of CD36 at a molecular scale, thereby reducing circulating lipid levels [151]. Caveolin functions in the formation of lipid droplets by associating fatty acids with binding proteins (FABP) [152]. Vitamin D receptor-interacting protein 205 (DRIP205), coactivator-associated arginine methyltransferase-1 (CARM-1), and protein arginine methyltransferase 1 (PRMT-1) are some of the secondary coactivators that play a role in FXR-mediated transcription [153]. CARM-1 acts by its protein methylase activity, known to interact indirectly with nuclear receptors of the p160 family. Steroid receptor coactivator 1 (SRC-1), one of the nuclear receptors of the p160 family, acts on RXR-FXR heterodimers. Peroxisome proliferator-activated receptor-gamma coactivator-1 alpha (PGC-1 alpha), a well-known coactivator of PPAR-gamma, shows more FXR coactivating properties than SRC-1. FXR activates PGC-1 alpha by binding to the NH2 position [154–156].
Clinical trials and US FDA-approved drugs
FXR is crucial in countering these pathological conditions, as it exerts effects on each of them. Here are a few clinical trial drugs and ligands targeting FXR, with treatment mentioned in Table 2. Rezdiffra (resmetirom), a novel thyroid hormone receptor beta (THRβ) agonist, was first approved for treating adults with noncirrhotic, MASH with moderate to advanced liver scarring [157]. Combined with diet and exercise, this drug reduces liver fat accumulation by selectively stimulating THRβ in the liver. However, Rezdiffra is associated with several side effects like pruritus, diarrhoea, nausea, vomiting, constipation, abdominal pain, and dizziness are commonly reported. Additionally, it may cause liver injury (hepatotoxicity) and gallbladder-related complications such as gallstones (cholelithiasis) and inflammation of the gallbladder (cholecystitis). Therefore, patients undergoing treatment with Rezdiffra should be closely monitored [158]. Due to the numerous severe side effects associated with Rezdiffra, there is an urgent need for new therapeutic options for MASLD. Given these concerns, FXR agonist drugs emerge as a promising alternative to fulfil this requirement, offering a potential pathway for safer and more effective treatment strategies for MASLD while minimising adverse effects.
Represents FXR agonist drugs for the MASLD/MASH under clinical trial phase II/III trails
Primary mechanism | Agent (trial name) | Structure | Clinical trials | NCT number(clinicaltrials.gov) | Reference |
---|---|---|---|---|---|
FXR agonist and a chemokine receptor type 2/5 antagonist | LJN452 and CVC (cenicriviroc) | ![]() ![]() | Phase 2b | NCT03517540 | [174, 175] |
FXR agonist | Cilofexor (GS-9674) | ![]() | Phase 2 | NCT02854605 | [176] |
FXR agonist and statin | Obeticholic acid and atorvastatin | ![]() ![]() | Phase 2 | NCT02633956 | [177] |
Non bile acid FXR agonist | HPG1860 | ![]() | Phase 2a | NCT05338034 | [178] |
FXR agonist | CS0159 | ![]() | Phase 2 | NCT05591079 | [179] |
FXR agonist | Obeticholic acid | ![]() | Phase 3 | NCT03439254 | [180] |
FXR agonist | EYP001a (vonafexor) | ![]() | Phase 2 | NCT03812029 | [181] |
FXR agonist | EDP-305 | ![]() | Phase 2 | NCT03421431 | [182] |
Tricyclic FXR agonist | HEC96719 | ![]() | Phase 2 | NCT05397379 | [183] |
Non-steroidal FXR agonist | PX-104 | ![]() | Phase 2 | NCT01999101 | [184] |
FXR: farnesoid X receptor; MASLD: metabolic dysfunction-associated steatotic liver disease; MASH: metabolic dysfunction-associated steatohepatitis
Exploring lipid diversity: a new frontier in understanding MASLD
Lipidomics is an emerging field that provides a comprehensive analysis of lipid compositions in biological samples, offering valuable insights into MASLD [159]. Studies of liver biopsies from MASLD patients have revealed significant alterations in lipid profiles, particularly in glycerophospholipids and sphingolipids. Notably, an increased n-6/n-3 fatty acid ratio and decreased levels of essential long-chain PUFAs, such as arachidonic and EPA, have been observed, indicating a shift in lipid metabolism that contributes to disease progression [53, 160]. In addition to liver tissue analysis, lipidomic studies of plasma samples have identified specific lipid changes that may serve as non-invasive biomarkers for disease progression. For example, significant alterations in phosphatidylcholine (PC), phosphatidylethanolamine (PE), and sphingomyelin levels have been documented between healthy individuals and those with varying stages of MASLD, including MASH as detailed described in Table 3 [161, 162]. These lipid changes highlight the critical role of lipid metabolism in the development and progression of MASLD. The accumulation of lipids within hepatocytes leads to increased metabolic demands, resulting in the production of ROS and oxidative stress [163]. This process is exacerbated by obesity-related expansion of adipose tissue and insulin resistance, which promote the release of FFAs and activate inflammatory pathways. Elevated levels of ceramides a class of sphingolipids have been linked to lipotoxicity and hepatic inflammation, further contributing to the pathogenesis of MASLD [34].
Quantification of fatty acids by using various analytical techniques
Authors name | Disease condition analysed | Metabolites altered | Analytical technique | Healthy vs. NASH patients |
---|---|---|---|---|
Masoodi et al. [185] | MASLD and MASH | Amino acids, fatty acids, triglycerides, phospholipids, and bile acids | Metabolomics and lipidomic by MS | Altered amino acid metabolism, and lipid disturbances |
Kartsoli et al. [186] | MASLD | Liver lipid species | Lipidomic approach by LC-MS/MS | Impact of liver lipid species on MASH development and progression |
Garcia-Jaramillo et al. [187] | MASH | Hepatic neutral and membrane lipids | Lipidomic analysis by UPLC-TOF-MS/MS | Profound alterations in lipid composition |
Wang et al. [188] | MASLD and MASH | The main types of lipids are glycerides, glycerophospholipids, sphingolipids, fatty acyl lipids, and sterol lipids. | Lipidomic profiling by UPLC-MS/MS | Differential lipid profiles in urine samples |
GC-FID | ||||
Piras et al. [189] | MASLD | Circulating lipid biomarkers | LC-MS | Potential biomarkers for MASLD |
Tan et al. [190] | MASLD and MASH | Circulating fatty acids, triglycerides, phospholipids, and bile acids | Metabolomics and lipidomics by imaging particle detector | Altered metabolic pathways |
Kalopitas et al. [191] | MASLD and MASH | Potential differences in plasma lipids | Lipidomic profiling by LC-MS and GC-MS | Comparison in MASH, MASLD, and heathy |
Zhu et al. [192] | NAFL and NASH | Urinary extracellular vesicles | Lipidomic analysis by mass-based approach | Lipidomic changes as potential markers |
Gaggini et al. [193] | NAFLD | Circulating lipids | Metabolomics by mass-based approach | Links between lipids and NAFLD |
NASH: non-alcoholic steatohepatitis; MASLD: metabolic dysfunction-associated steatotic liver disease; LC-MS: liquid chromatography-mass spectrometry; UPLC-TOF-MS: ultra-performance liquid chromatography time-of-flight mass spectrometry; UPLC-MS: ultra-performance liquid chromatography-mass spectrometry; GC-FID: gas chromatography flame ionization detector; GC-MS: gas chromatography-mass spectrometry; NAFL: non-alcoholic fatty liver; NAFLD: non-alcoholic fatty liver disease
To analyse these complex lipid profiles, researchers utilize hyphenated techniques such as liquid chromatography-mass spectrometry (LC-MS) and gas chromatography-mass spectrometry (GC-MS) as represented in Figure 4. These methodologies enable the identification and quantification of thousands of lipid species, enhancing our understanding of their roles in liver disease [164–166]. Lipidomic profiling is crucial for uncovering the specific lipid alterations associated with MASLD [60, 167]. By identifying potential biomarkers for distinguishing between MASLD and MASH, lipidomics offers promising avenues for early diagnosis and targeted therapeutic strategies aimed at restoring lipid homeostasis and improving patient outcomes [168].
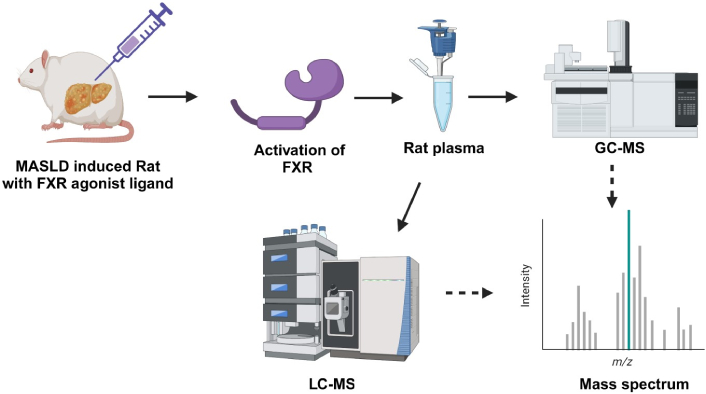
Quantification of lipid profiles in MASLD-induced rats treated with FXR agonists. This visual emphasizes the potential of FXR agonist therapy as a promising intervention for MASLD and illustrates the critical role of lipid profiling in monitoring disease progression and therapeutic outcomes. MASLD: metabolic dysfunction-associated steatotic liver disease; FXR: farnesoid X receptor; GC-MS: gas chromatography-mass spectrometry; LC-MS: liquid chromatography-mass spectrometry. Created in BioRender. Pirangi, S. (2025) BioRender.com/x10t173
Conclusions
In this review, we have clearly articulated the renaming of NAFLD to MASLD and NASH to MASH. We explored the association of MASLD with features of metabolic syndrome, emphasizing the central role of fatty acids in disease development and progression. Additionally, we examined the role of FXR in both physiological and pathological conditions, detailing how hepatic steatosis can progress to severe outcomes like fibrosis and CKD. The therapeutic potential of FXR agonists in treating MASLD and other metabolic diseases was discussed, alongside a review of clinical trial drugs targeting the FXR receptor. Furthermore, we highlighted the importance of lipidomic studies for quantifying fatty acids responsible for MASLD and MASH pathogenesis using advanced analytical techniques.
Despite these insights, significant research gaps remain due to the complex pathology of MASLD. Over 30% of the global population is affected by MAFLD, highlighting this silent epidemic’s growing prevalence. Current diagnostic techniques are limited, necessitating improvements in lipidomics through advanced methods like LC-MS/MS and GC-MS/MS to accurately assess disease severity. Optimizing sample preparation protocols and minimizing matrix effects are essential steps forward. Currently, only one drug, resmetirom, has been approved by the FDA for MASLD treatment. While FXR agonists show promise in mitigating disease pathology, side effects have hindered others, such as OCA, from progressing to phase III trials. There is a pressing need for early-stage identification of MASLD and further advancements in lipidomic studies, alongside the development of new therapeutic options to address this widespread health issue. By pursuing these avenues, we can enhance our understanding and management of MASLD, ultimately improving patient outcomes in this prevalent liver disease.
Abbreviations
CARM-1: | coactivator-associated arginine methyltransferase-1 |
CAV-1: | caveolin-1 |
CD36: | cluster of differentiation 36 |
CDCA: | chenodeoxy cholic acid |
ChREBP: | carbohydrate response element-binding protein |
CKD: | chronic kidney disease |
DAG: | diacylglycerol |
FASN: | fatty acid synthase |
FATPs: | fatty acid transport proteins |
FFAs: | free fatty acids |
FGF-15: | fibroblast growth factor-15 |
FXR: | farnesoid X receptor |
FXREs: | farnesoid X receptor response elements |
GC-FID: | gas chromatography flame ionization detector |
GC-MS: | gas chromatography-mass spectrometry |
HCC: | hepatocellular carcinoma |
HDL: | high-density lipoprotein |
LC-MS: | liquid chromatography-mass spectrometry |
LDL: | low-density lipoprotein |
MASH: | metabolic associated-steatotic hepatitis |
MASLD: | metabolic-associated steatotic liver diseases |
MS: | mass spectrometry |
MUFA: | monounsaturated fatty acids |
NAFL: | non-alcoholic fatty liver |
NAFLD: | non-alcoholic fatty liver disease |
NASH: | non-alcoholic steatohepatitis |
OCA: | obeticholic acid |
PBC: | primary biliary cholangitis |
PPARα: | peroxisome proliferator-activated receptor alpha |
PUFAs: | polyunsaturated fatty acids |
RXR: | retinoid X receptor |
SCD: | stearoyl-CoA desaturase |
SFA: | saturated fatty acids |
SHP: | small heterodimer partner |
SRC-1: | steroid receptor coactivator 1 |
SREBP-1c: | sterol regulatory element-binding protein-1c |
T2DM: | type 2 diabetes mellitus |
TAG: | triacylglycerol |
TGs: | triglycerides |
THRβ: | thyroid hormone receptor beta |
UDCA: | ursodeoxycholic acid |
UFA: | unsaturated fatty acids |
VLDL: | very low-density lipoprotein |
Declarations
Acknowledgments
The authors sincerely thank NIPER, S.A.S Nagar, and the Department of Pharmaceuticals, Ministry of Chemicals and Fertilizers, India, for providing infrastructure and Ph.D. fellowship.
Author contributions
PS: Conceptualization, Investigation, Writing—original draft, Writing—review & editing. KMS, VP, and DK: Writing—original draft, Writing—review & editing. SN: Conceptualization, Investigation, Validation, Writing—review & editing, Supervision.
Conflicts of interest
The authors declare that there are no conflicts of interest.
Ethical approval
Not applicable.
Consent to participate
Not applicable.
Consent to publication
Not applicable.
Availability of data and materials
Not applicable.
Funding
Not applicable.
Copyright
© The Author(s) 2025.
Publisher’s note
Open Exploration maintains a neutral stance on jurisdictional claims in published institutional affiliations and maps. All opinions expressed in this article are the personal views of the author(s) and do not represent the stance of the editorial team or the publisher.