Abstract
Aim:
Regenerative and curative strategies would be desirable for neurodegenerative and adrenal diseases, and multipotent adrenal stem cells are considered as promising biological tools for this purpose. Stem-like cells with the potential to proliferate and differentiate in vivo and in vitro were discovered in both cortex and medulla of the adrenal gland. Previously, it was demonstrated that nestin-positive progenitors in the cortex and medulla, play an important role under stress. In the present study, the cultivation of these cells was optimized and their growth in vitro was characterized.
Methods:
Primary cells from the adrenal cortex and medulla from Nes-GFP mice were isolated and the in vitro culture conditions promoting the growth of stem and progenitor cells using different 3-dimensional (3D) spheroid culture models were optimized.
Results:
Both cortical and medullary cells could be cultured for at least one month under several different low-adherence conditions maintaining their viability and potential to differentiate. Medullary cells grew faster than cortical cells. Endothelin did not affect the cultures.
Conclusions:
Adrenomedullary and adrenocortical nestin-positive progenitor cells can be cultured long-term in 3D cultures maintaining their proliferation and differentiation capabilities. Such multidimensional models can potentially be used for drug screening to develop personalized medicines or for transplantation to treat neurodegenerative disorders or adrenal diseases, such as adrenal insufficiency.
Keywords
Stem cells, adrenal, cortex, medulla, spheroids, 3-dimensional culture, nestin, endothelinIntroduction
In the last couple of years, continued progress in stem cell technologies has shown the huge potential of cell replacement strategies for several diseases, e.g., neurodegenerative disorders such as Alzheimer’s disease, Parkinson’s disease, Huntington’s disease, ataxia, and amyotrophic lateral sclerosis, that are extremely challenging to treat with conventional pharmaceutical approaches [1].
For endocrine diseases, such as type 1 diabetes, stem-cell-derived islets have shown promising results for regenerative therapies [2–4], but for adrenal insufficiency, no similar approaches are currently available. Adrenal insufficiency is caused by several adrenal disorders, and lifelong management of these patients with exogenous steroids is challenging [5, 6]. No drug suitably mimics the diurnal pattern of cortisol noted in healthy individuals, and there are no objective variables to measure the quality of hormone replacement therapy [7].
In vitro, adult stem cells from urine, adipose tissue, bone marrow, or blood have been successfully reprogrammed into steroid-producing cells by forced expression of steroidogenic factor 1 (SF-1), the master regulator of steroidogenesis and differentiation of pituitary gonadotrophs, adrenal glands, and gonads [8, 9]. Cells were viable after transplantation into mice, but full functionality and responsiveness to stimuli were not reported [8]. Recently, Sakata et al. [10] succeeded in differentiating human induced pluripotent stem cells (iPSCs) into fetal zone-like adrenocortical cells without forced expression of SF-1. These cells produced androgens but no mineralo- and glucocorticosteroids.
Previously, we developed 3-dimensional (3D) culture models of primary porcine and bovine adrenal cells [11] and were able to demonstrate that total bovine adrenocortical cells transplanted into animal models were functional and could restore adrenal insufficiency [12]. In addition, we developed multidimensional models using human adrenal cell lines and primary cells isolated from adrenocortical tumors and pheochromocytomas [11, 13].
In the current study, we used these multidimensional models for nestin-positive mouse adrenal progenitors, which were found to proliferate and differentiate into steroid-producing and chromaffin cells, respectively [14, 15]. We optimized various methodologies for generating spheroids to cultivate them over extended periods while maintaining their functional properties.
Materials and methods
Animals
Heterozygous Nes-GFP transgenic mice [16] on the C57BL/6N genetic background were generated as described previously [14]. All mouse colonies were maintained under a 12:12 h light/dark cycle and fed ad libitum. Mice were sacrificed by cervical dislocation and all animal experiments were approved according to the German Animal Welfare Act by Landesdirektion Sachsen, Germany.
Isolation and culture of adrenal cells
Adrenals from 2–3 mice (age 2–3 months, both sexes) per experiment were resected and placed in Petri dishes with ice-cold phosphate buffered saline (PBS). Fat tissue surrounding the adrenals was carefully removed and the adrenal cortex was thoroughly isolated from the medulla as described previously [17]. All cortical and medullary tissues, respectively, were pooled, pelleted (270× g, 5 min), and digested for 20 min at 37℃ while shaking [1.8 mg/mL collagenase, 10 mg/mL bovine serum albumin (BSA), 0.18 mg/mL deoxyribonuclease (DNase) in PBS; all from Merck]. The digestion was stopped by washing twice in PBS and primary adrenomedullary and adrenocortical cells were suspended in 1 mL complete medium consisting of Dulbecco’s modified eagle medium (DMEM/F12; Gibco, Thermo Fisher Scientific) containing 10% fetal bovine serum (FBS; Merck), 1% antibiotic-antimycotic solution (Gibco, Thermo Fisher Scientific), 1% L-glutamine (Merck) and 20 ng/mL basic fibroblast growth factor (bFGF; Merck).
Generation and cultivation of cell spheroids
Different amounts of cells (500–10,000 cells/well) suspended in a complete cell culture medium containing 20% of a 1.2% methylcellulose solution [0.24% (w/v) in DMEM/F12 medium] were seeded in round-bottom 96-well plates for suspension culture (Greiner Bio-One). In a second setup, cells were suspended in a complete medium and seeded in round-bottom 96-well Nunclon™ Sphera™ plates with an ultra-low binding surface (Thermo Fisher Scientific). In both setups, the medium was replaced every 3–4 days by carefully removing 100 µL medium and adding 100 µL fresh medium. In a third setup, adrenocortical and adrenomedullary cells suspended in complete medium were seeded in 24-well ultra-low attachment Sphericalplate 5D, where each well contains 750 rounded microwells (Kugelmeiers).
Fluorescent-activated cell sorting
For cultivation of nestin-positive cells alone, green fluorescent protein (GFP)-positive and GFP-negative cells from the adrenal medulla and cortex of Nes-GFP mice were flow sorted on a BD FACSAria™ II flow cytometer (BD Biosciences) using BD FACSDiva™ Software using 10 µg/mL propidium iodide (BioLegend) as a live/dead cell marker. Cells were sorted directly in a complete medium containing bFGF. Afterwards, cells were seeded in Sphericalplate 5D (Kugelmeiers).
Cell differentiation
To assess the in vitro differentiation of isolated adrenal cells, spheroids (after 28 days of proliferation), were transferred to Corning® 96-well Polypropylene Microplates (Corning) coated with 1 mg/mL poly-D-lysine (Merck Millipore®) and 3 µL/mL fibronectin (R&D Systems) and cultured in the absence of bFGF as described previously [17]. The medium was replaced by fresh medium every 2–3 days.
5-ethynyl-2’-deoxyuridine labeling and staining
Spheroids cultured for 10 days were transferred to poly-D-lysine and fibronectin-coated 96-well plates as described above. They were treated with 10 mmol/L 5-ethynyl-2’-deoxyuridine (EdU) for 3 h, followed by fixation in 4% paraformaldehyde (PFA) in PBS for 15 min. After careful washing with PBS for 3 × 5 min, spheroids were stained for EdU incorporation using the Click-iT™ Plus EdU Alexa Fluor 647 Imaging Kit (Thermo Fisher Scientific) following the instructions of the manufacturer. Nuclei were stained with 4’-6-diamidino-2-phenylindole (DAPI; Thermo Fisher Scientific) and slides were mounted with a fluorescent mounting medium (Aqua-Poly/Mount; Polysciences).
Immunohistochemistry
Immunofluorescence staining of adrenal sections was performed as described previously [14]. Briefly, adrenal glands were fixed in 4% PFA in PBS for 4 h and cryoprotected overnight in 30% sucrose/PBS at 4℃. Afterward, they were embedded in Tissue-Tek® Medium (O.C.T.; Sakura Finetek), and stored at –80℃. Cryosections were sliced to 7 µm thickness (Leica CM1900; Leica Biosystems) and mounted on Superfrost™ Plus Microscope Slides (Thermo Fisher Scientific). Slides were immunostained using specific antibodies against the endothelin receptors A and B (Alomone Labs). Nuclei were stained with DAPI (Thermo Fisher Scientific) and slides were mounted with a fluorescent mounting medium (Aqua-Poly/Mount; Polysciences).
Confocal laser scanning microscopy and fluorescence microscopy
Confocal imaging was performed with a Zeiss LSM 880 inverted confocal laser scanning microscope and ZEN 2010 software (Zeiss). Fluorescence microscopy was performed with a Zeiss Axiovert 200M fluorescence microscope and AxioVision software (Zeiss). Image processing and analysis were carried out using ImageJ software, version 1.52h.
Spheroid formation was followed using an inverse microscope Axiovert 200M (Software: AxioVision 4.8; Carl Zeiss Microscopy). Each spheroid’s area (A) was analyzed using the software package Fiji (ImageJ, 1.52h; National Institutes of Health). The diameter (D) was calculated under the acceptance of an approximately spherical form of the spheroids:
Endothelin
There are striking parallels between the development of carotid body glomus cells and adrenal chromaffin cells. Therefore, to analyze the effect of endothelin 1 on the formation of the adrenal spheroids, the cell suspension (2.500 cells/well) containing 20% of a 1.2% methylcellulose solution was treated with endothelin 1 (10 nmol/L or 100 nmol/L; Sigma-Aldrich) starting on day 4 of cultivation. The medium was changed every 3 days.
Viability assay
The CellTiter 96® AQueous One Solution Cell Proliferation Assay (Promega) was used to investigate cell viability. In analogy to the manufacturer’s instructions, primary adrenocortical cells were seeded in 96-well plates and incubated for 24 h. After 3 h incubation at 37℃ with CellTiter 96® AQueous One reagent the absorption of the whole plate was measured at 490 nm.
Statistical analysis
Statistical analysis was performed using the GraphPad Prism program version 9.0.0 (GraphPad Software Inc.), and statistical significance was determined using two-way analysis of variance (ANOVA) followed by a Bonferroni multiple comparison test correction where appropriate. A student’s t-test was performed when only two means were compared. The significance was defined as not significant (ns) P > 0.05; * P < 0.05; ** P < 0.01; *** P < 0.001.
Results
Spheroid formation
For the development of 3D adrenal spheroid cultures, we utilized adrenals from Nes-GFP mice to specifically follow nestin-positive stem cells/progenitors. To assess the ability of adrenocortical and adrenomedullary primary cells to form spheroids, two different methods were compared. One method made use of low-adherence round-bottom 96 well plates. In the other method, normal 96-well round bottom plates, with 0.24% methylcellulose added to the culture medium to avoid adherence, were used [18].
First, we tested five different amounts of total primary adrenocortical or adrenomedullary cells to achieve the optimal seeding density (500–10,000 cells/well). As shown previously, under these culture conditions within the first days fully differentiated cells die, whereas mainly stem and progenitor cells survive and form spheroids [14]. On day 1 after seeding, cells aggregated at the bottom of the culture plates, but no spheroids were assembled. As observed previously [14], in the medullary cultures around 10% of the cells were positive for GFP, whereas in the cortical cultures, only around 1–2% of the cells were GFP-positive (Figure 1A). On days 3–4, spheroid formation took place and the expression of nestin-GFP increased in both cell populations. Spheroids were formed with all amounts of cells, but within the cortical cultures, the spheroids quickly dissociated when using 5,000 or 10,000 cells per well demonstrating that the biggest stable spheroid diameter is around 100 µm. This was independent of the method used (methylcellulose or low-adherence) (Figure 1B). In the medullary cultures, the spheroids showed the highest stability using methylcellulose (Figure 1B). Therefore, we decided for future experiments to use 2,500 cells per well and culture them in normal 96-well round bottom plates in medium containing 0.24% methylcellulose.
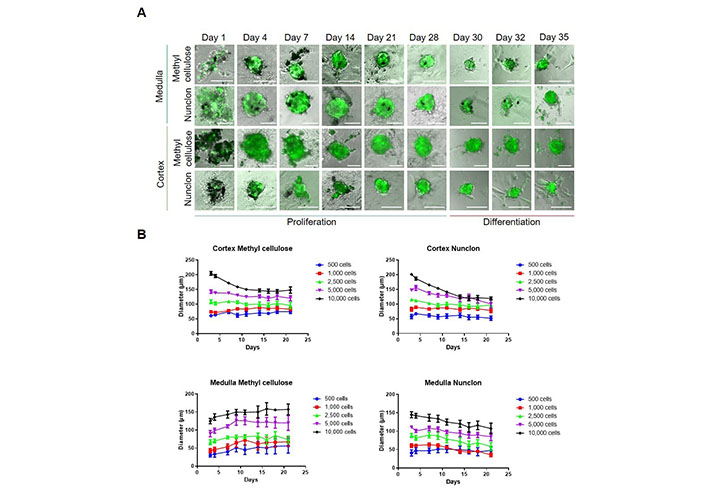
Isolation and spheroid cultivation of adrenal stem- and progenitor cells. A. Medullary and cortical cells were cultured for up to 28 days under low-adherence conditions promoting the survival of stem and progenitor cells. Two different set-ups were used; methylcellulose or Nunclon low-adherence plates. After 28 days, spheroids were transferred to plates coated with poly-D-lysine and fibronectin promoting cell adherence and differentiation. Representative pictures are shown. Scale bars, 100 µm; B. spheroid diameters at different cell concentrations were measured (n = 9). The number of cells seeded per well is indicated
Medullary cells show a higher proliferation rate than cortical cells
To test whether primary medullary and cortical cells differ in their proliferation capabilities, we added the thymidine analog EdU to the cultures on day 9. At this time point, medullary cells showed a significantly higher proliferation rate than cortical cells (Figure 2A). Therefore, we measured the viability of adrenocortical cells after 4 weeks to exclude cell death. No statistically significant differences were found between the different cell concentrations, but viability tended to be higher at 2,500 or more cells per well than at 500–1,000 cells per well (Figure 2B). No difference between low-adherence plates and methylcellulose could be observed. We cultured both medullary and cortical spheroids for 4 weeks and then we changed the culture conditions to adherence and removed bFGF from the medium. Thereby, cells adhered and started to differentiate (Figure 1B) showing the long-term potential of the cultures.
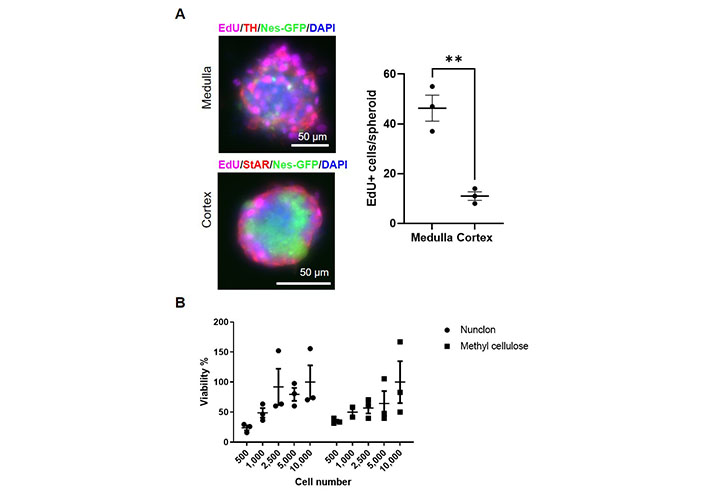
Proliferation and viability of adrenal stem and progenitor cells. A. Day 9 spheroids treated with EdU. The absolute numbers of EdU-positive cells per spheroid were counted. TH: tyrosine hydroxylase; StAR: steroidogenic acute regulatory protein. ** P < 0.01 (unpaired two-sided t-test); B. viability of adrenocortical spheroids cultivated in either Nunclon low-adherence plates or in medium containing methylcellulose after 4 weeks of culture. The number of cells seeded per well is indicated. (ns) P > 0.05 (two-way ANOVA followed by a Bonferroni multiple comparison test)
The effect of endothelin
Neural crest-derived stem cells in the adult mammalian carotid body are highly sensitive to hypoxia and endothelin [19, 20]. As there are striking parallels between carotid body glomus cells and adrenal chromaffin cell development [21], we decided to evaluate the effect of endothelin on adrenomedullary stem cells and compare them to adrenocortical cells.
Previously, we performed bulk RNA sequencing of nestin-GFP cells from the adrenal cortex and medulla, respectively. This showed that nestin-GFP from the cortex and medulla are distinct. Using this dataset (GEO: GSE1402479), in medullary nestin-GFP cells we observed a 2.97 log2fold (padj = 0.0047) higher expression of the endothelin receptor gene Ednrb and a 9.06 log2fold (padj = 2.02 × 10–25) higher expression of the Edn3 gene encoding endothelin 3 than in cortical nestin-GFP cells. At the protein level, we observed both endothelin receptors A and B in the adrenal medulla, but with a very low expression in nestin-GFP cells (Figure 3A). We anyway added endothelin to our spheroids as they might contain other cells that are reactive to endothelin. However, for both medullary and cortical spheroids, no effect was observed on the spheroid diameter (Figure 3B) verifying that the spheroids mainly consist of cells that are not reactive to endothelin.
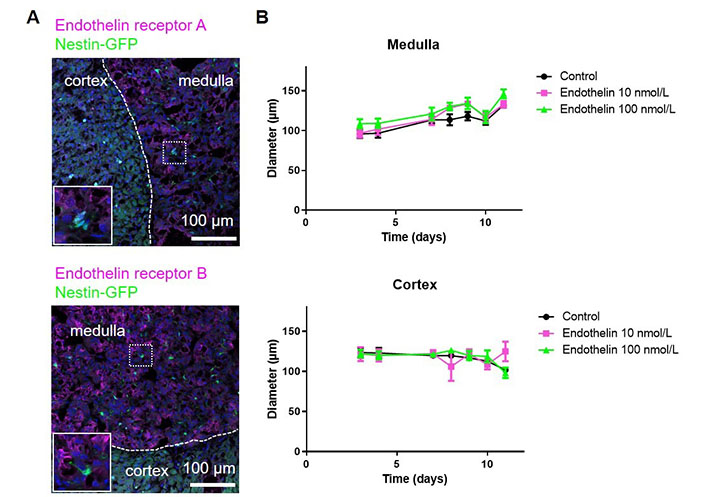
The effect of endothelin on adrenal spheroids. A. Adrenal sections from Nes-GFP mice were stained for the endothelin receptors A and B; B. adrenal spheroids generated from either medullary or cortical cells were treated with endothelin and the spheroid diameters were measured. (ns) P > 0.05 (two-way ANOVA followed by a Bonferroni multiple comparison test)
High-throughput 3D culture
For potential high-throughput applications, such as drug screening or personalized medicine, we upscaled the number of spheroids, by using Sphericalplates 5D with 750 microwells per well in a 24-well plate. In these wells, both cortical and medullary adrenal cells formed spheroids comparable to those created in 96-well plates, although slightly smaller. The percentages of nestin-GFP positive cells (medulla: ~10%, cortex: 1–2%) were as for the spheroids cultured in 96-well plates. For cortical cells, because of the lower proliferation, 500 cells per microwell were necessary, whereas, for medullary cells, only 100 cells per microwell were needed (Figure 4A). To further test if nestin-GFP cells alone were able to create spheroids, we flow-sorted these cells from cortex and medulla, respectively, and small amounts per well were sorted in Sphericalplates 5D (Figure 4B). These cells divided and survived up to at least 4 weeks but did not form spheroids indicating that higher amounts of cells or other supporting cells are necessary for spheroid formation.
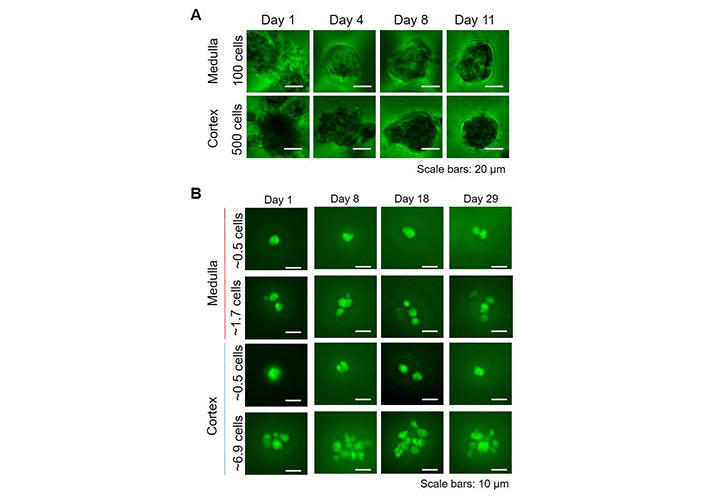
3D high throughput spheroid cultures. A. Total adrenocortical or adrenomedullary cells were cultured in Kugelmeier Sphericalplates 5D. The number of cells seeded per microwell is indicated; B. FACS-sorted nestin-GFP cells from the adrenal cortex and medulla, respectively were seeded in Kugelmeier Sphericalplates 5D in different concentrations. The average number of cells seeded per microwell is indicated
Discussion
Spheroids are promising tools for cell replacement strategies. Furthermore, in a high throughput format, they can be used for in vitro drug screening. In the current study, we have optimized spheroid formation with cells from the adrenal medulla and cortex from mice. For the culture and characterization of separate adrenal spheroids, a 96-well format was the best approach, whereas, for high-throughput approaches, the Sphericalplate 5D turned out to be the best option. We have previously used a serum-free medium (Neurobasal™, Gibco, Thermo Fisher Scientific) supplemented with B27 for the cultivation of adrenomedullary spheroids in 24-well low-adherence plates with several spheroids per well [14, 22]. However, in the current study, where the adrenomedullary spheroids were cultured with just one spheroid per well in a 96-well plate, DMEM/F12 medium containing 10% FBS, demonstrated better long-term growth and allowed for long-term follow-up of individual spheroids. In essence, the adrenomedullary spheroids demonstrated a higher proliferation rate than the adrenocortical spheroids in the same medium. The spherical plate experiment with sorted nestin-GFP cells from both the cortex and medulla revealed that the cells can survive as single cells, but that a certain limit (> 100 cells) has to be reached before the cells start to form aggregates/spheroids. Despite the similarities between the carotid body and the adrenal medulla, endothelin did not show any effect on the spheroid cultures.
As it will not be feasible to transplant humans with cells from mice, the knowledge and methodologies we have acquired and developed with mouse cells may also be applied for the isolation and culture of human stem cells/organoids, which can be used for auto- or allotransplantation in patients. Transplantations of fetal or embryonic stem cell-derived tissues are known to elicit a host immunogenic response, therefore autologous transplantation using cell types derived from one’s own cells eliminates the risks of tissue rejection and reduces the need for immunosuppressants [23]. Another advantage of using adult adrenal stem cells is that the cells are already destined to become either mature adrenomedullary or adrenocortical cells. This compares to other protocols using, for example, urine-derived stem cells, where the cells have been found to produce mainly testosterone rather than glucocorticoids. The disadvantage of adrenal stem cell autotransplantation is that it is costly and time-consuming.
In contrast, iPSCs can be produced in large quantities, making it a commercially available product. Xenogenic or iPSC cell sources have drawn attention to macro-encapsulation strategies to control the potential risks of inflammatory responses and graft rejection. In addition, endocrine tissues, such as pancreatic islets and the adrenal, are complex multi-cellular functional entities that are highly dependent upon their specific microenvironment. Our group has developed a macro-encapsulation system that allows sufficient immune isolation while maintaining normal cell function. This concept has been successfully tested in several preclinical diabetes models and in the first clinical case of type 1 diabetes and has now entered the clinical trial phase [24, 25]. Based on this experience, the concept was transferred to adrenal cell replacement therapy, in which bovine or porcine adrenal cortical cells encapsulated in alginate were transplanted into adrenalectomized rats. With this procedure, chronic immunosuppression could be prevented [12]. Moreover, this model provided a microenvironment that ensured 3D cell interaction and in which fibrosis was suppressed.
Another approach to achieve the right microenvironment and extracellular matrix is to use micro-organ matrices (MOMs) that recreate and mimic the normal extracellular environment and provide cells with necessary nutrients and growth factors. For pancreatic cadaveric islets surrounded by MOMs, it was already shown that they are longer viable than naked islets, are more glucose-responsive, secrete insulin in similar quantities to freshly isolated islets in a glucose-regulated manner, and have a better vascularization [26–28].
Nestin-positive cells from the adrenal medulla might have the potential to be used for cellular therapies for neurodegenerative diseases, such as Parkinson’s disease due to the close relationship of chromaffin cells to catecholaminergic neurons. Several studies have promoted the use of chromaffin cells for this purpose [29]. However, as we have described previously, because of lacking success using this approach [30, 31], adrenomedullary stem cells have been suggested as a better cell source for replacement therapy for Parkinson’s disease [32]. Compared to chromaffin cells, a significantly higher population of spheroid-derived cells has been shown to acquire a dopaminergic phenotype [33, 34]. Furthermore, in addition to their neuronal-like phenotype, nestin-positive adrenomedullary cells show glia-like features [22], which could make them suitable for the treatment of Alzheimer’s disease, Parkinson’s disease, and amyotrophic lateral sclerosis [1].
As mentioned above, we have previously shown that nestin-positive cells can differentiate into steroid-producing cells in vitro and in vivo [14, 15]. With the current optimizations of the spheroid cultivation protocol, these cells have the potential to be used for the treatment of adrenal insufficiency. The next step would therefore be to test the functionality of these cells/spheroids in an animal model of adrenal insufficiency, e.g., in mice that have undergone bilateral adrenalectomy. Subsequently, the protocol can be used as a starting point for optimizing the cultivation of various human cell sources, such as embryonic stem cells, iPSCs, or primary adrenal cells. Thus, adrenal spheroids combined with novel biomaterials and encapsulation in immune-isolating devices might offer alternative therapies for patients with adrenal and neurodegenerative diseases. Furthermore, adrenal spheroids derived from mouse or human cell sources might be used for drug screening.
Abbreviations
3D: | 3-dimensional |
ANOVA: | analysis of variance |
bFGF: | basic fibroblast growth factor |
DMEM: | Dulbecco’s modified eagle medium |
EdU: | 5-ethynyl-2’-deoxyuridine |
GFP: | green fluorescent protein |
iPSCs: | induced pluripotent stem cells |
ns: | not significant |
PBS: | phosphate buffered saline |
Declarations
Acknowledgments
We thank Uta Lehnert, Linda Friedrich, and Isabel Poser for their technical assistance. Susann Höffner from the FACS facility at the CMCB Technology Platform at TU Dresden is thanked for her assistance with the cell sorting.
Author contributions
CS and NB: Conceptualization, Investigation, Validation, Writing—original draft, Writing—review & editing, Supervision. SF: Conceptualization, Validation, Writing—review & editing. GAS, RO, PK and BL: Writing—review & editing. All authors read and approved the submitted version.
Conflicts of interest
PK works by the company Kugelmeiers, which sells the Sphericalplate 5D.
Ethical approval
All animal experiments were approved according to the German Animal Welfare Act reference number TVA 17/2017 by Landesdirektion Sachsen, Germany.
Consent to participate
Not applicable.
Consent to publication
Not applicable.
Availability of data and materials
The datasets analyzed for this study can be found in the GEO repository under the number GSE 140247.
Funding
This work was supported by the
Copyright
© The Author(s) 2024.