Abstract
Aim:
In the present study, bunch stem and cane extracts (Vitis vinifera L. cv. Malbec) rich in phenolic compounds (PCs) like flavonoids, phenolic acids, and stilbenes are studied as potential anticancer candidates.
Methods:
Twenty-three PCs were quantified by liquid chromatography-diode array fluorescence detection (LC-DAD-FLD). In vitro cytotoxic activity of both extracts on healthy (HBL-100) and colorectal cancer (HCT-116) human cell lines was assessed by 3-(4,5-di-methylthiazol-2-yl)-2,5-diphenyl tetrazolium bromide (MTT) reduction assay.
Results:
Cane extract did not show cytotoxic effect for the tested lines, which can be considered as an advantage for its application in the pharmaceutical industry. Conversely, the bunch stem extract showed a dose-dependent cytotoxic effect on HCT-116 and an IC50 of 680 µg/mL after 48 h of incubation; but not reported cytotoxic activity on the healthy cell line, evidencing a beneficial selective activity. The reported results encourage further investigation of these extracts as potential preventive and/or therapeutic drugs, or their combined use with chemotherapeutic treatments that lead to a potential dose reduction.
Conclusions:
The results preliminarily demonstrated that the extracts have potential anticancer properties or do not cause damage at the cellular level, encouraging their application as functional/nutraceutical or phytotherapeutic agents.
Keywords
Bioactive polyphenols, cytotoxicity, grapevine by-products, anticancer activity, phytotherapeutic agentsIntroduction
In recent years, the incidence of cancer has grown exponentially, so finding the best strategy for its treatment is one of the main challenges for health scientists. Chemotherapy is one of the main approaches to cancer treatment. However, drug toxicity and the development of tumor resistance constitute serious limitations of current cancer treatments [1]. The search for new anticancer with improved activity and less toxicity than conventional therapies has drawn particular interest in natural plant compounds.
In the last years, research has focused on the utilization of phytochemicals as potential anticancer candidates because of a lot of successful reports of high efficacy, low toxicity, and minimal side effects [2]. This is the case for the phenolic compounds (PCs), which have been shown to induce apoptosis and cell cycle arrest, and produce antiproliferative effects against many human cancer cell lines [3]. Therefore, these compounds could be safe and effective in potential cancer prevention and therapy. Most of the investigations have been carried out with individual PC for a better understanding of the biological and cellular mechanisms of their anticancer efficacy. However, several studies have confirmed the improvement when they are applied in combination with other different PC, exhibiting synergistic effects and improved bioavailability [4].
Viticulture generates a significant volume of wastes, including bunch stem in the winery and canes from vineyards, which are investigated as alternative sources of a wide range of PCs with potential bioactive properties [5]. Canes are the result of a management practice on grapevine plants performed every year aimed to prune the plants to improve grape yield stability and quality of grapes. Normally, this activity annually generates an average amount of roughly 2.5 tons per ha of grape cane residues [6]. Bunch stems are the other lignocellulosic by-product that accumulates during the destemming step and constitutes 5% of the processed grapes at harvest time [7]. In previous studies, bunch stem and cane extracts have shown considerable amounts of PC-like flavonoids, phenolic acids, and stilbenes [5, 8]. Consequently, the extracts have also reported significant antioxidant activities. Apostolou et al. [9] demonstrated the protective activity of bunch stem extracts against ROS-induced DNA damage. In another work, bunch stem PC modulated H2O2-induced oxidative stress by maintaining the redox balance in human epidermal keratinocytes (HaCaT cells) [10]. This protective antioxidant capacity was also reported in an in vitro intestinal barrier model (differentiated Caco-2 cells) [11], so bunch stem extracts could be useful not only in the treatment of cancer but also in the prevention of its appearance. Whereas for cane extracts, Medrano-Padial et al. [12] reported a protective and reducing role against oxidative stress induced in human cell lines. Some authors also reported that bunch stem and cane extracts can inhibit the growth of cancer cell lines as lung (SK-MES-1), liver (HepG2), cervix (HeLa), stomach (AGS), bladder (J28), colon (Caco-2, HT-29, Lovo), breast (MCF-7, MDA-MB-231), kidney (786-0, Caki-1), thyroid (k1), and prostate (PC-3) [9, 12–17]. This wide range of inhibitory action in several types of cancers suggests that the extracts of both matrices have great potential for the prevention and/or treatment of the disease and could be used as nutraceutical and/or pharmaceutical agents.
The aim of this study was to chemically characterize and evaluate the in vitro cytotoxicity of the bunch stem and cane extracts of Vitis vinifera L. cv. Malbec against human HCT-116 (colorectal cancer) in comparison with non-tumoral cell lines (epithelial HBL-100).
Materials and methods
Analytical reagents and standards
Liquid chromatography (LC)-grade acetonitrile, formic acid, MeOH, and EtOH were obtained from Mallinckrodt Baker Inc. (Phillipsburg, NJ, USA). Other reagents used were of analytical grade. Ultrapure water was obtained from a Milli-Q system (Millipore, Billerica, MA, USA). Anhydrous dimethyl sulfoxide (DMSO) (≥ 99.9%) was purchased from Sigma-Aldrich (Saint Louis, MO, USA) and fetal bovine serum (FBS) from Internegocios S.A. (Mercedes, Argentina). RPMI-1640 and Dulbecco’s Modified Eagle Medium (DMEM), penicillin and streptomycin, phosphate buffered saline pH 7.4, and TrypLE™ Express trypsin were purchased from Thermo Scientific (GIBCO, USA).
Standards of gallic acid (99%), 3-hydroxytyrosol (≥ 99.5%), (–)-gallocatechin gallate (≥ 99%), (–)-epigallocatechin gallate (≥ 95%), (+)-catechin (≥ 99%), procyanidin B1 (≥ 90%), procyanidin B2 (≥ 90%), (–)-epicatechin (≥ 95%), astilbin (≥ 98%), caffeic acid (99%), syringic acid (≥ 95%), caftaric (≥ 97%), trans-piceid (≥ 95%), trans-resveratrol (≥ 99%), trans-piceatannol (99%), (+)-ɛ-viniferin (≥ 95%), quercetin 3-β-D-glucoside (≥ 90%), quercetin 3-β-D galactoside (≥ 97%), myricetin (≥ 96%), kaempferol-3-glucoside (≥ 99%), and naringenin (≥ 95%) were purchased from Sigma-Aldrich (Steinheim, Germany). Pterostilbene standard (> 98%) was purchased from TCI (Tokyo, Japan) and 2-(4-hydroxyphenyl)ethanol (tyrosol) (≥ 99.5%) was obtained from Fluka (Buchs, Switzerland). Stock solutions of PC were prepared in MeOH at concentration levels of 1,000 mg/mL. Calibration standards were prepared in the initial mobile phase for the chromatography procedure.
Selection and preparation of samples
Canes and bunch stems of Vitis vinifera L. cv. Malbec were collected during the 2017/18 season from vineyards located in the Gualtallary region, Mendoza, Argentina. This variety was selected based on previous studies due to its higher content of PCs and total antioxidant capacity compared to the other varieties studied. Both samples were prepared and conditioned according to Ferreyra et al. [6, 7].
Extraction and characterization of PCs
In order to extract the PCs from the samples, a solid-liquid extraction was carried out according to the previous protocol reported by Ferreyra et al. [18]. Briefly, 1 g of powdered sample was extracted with 50 mL of EtOH:H2O (50:50, v/v) in an ultrasonic bath at 50 Hz for 60 min at 60°C. The mixture was centrifuged at 806 g for 10 min at 25°C and filtered. The extract was concentrated in a rotary evaporator at 40°C to remove the organic solvent. The final concentration of the aqueous extracts was set at 0.04 g of sample dry weight per milliliter extracted (g dw/mL). Before use for characterization and anticancer studies, the aqueous extracts were sterilized by filtration (0.22 μm) and stored at −80°C. An aliquot of the extracts was used for the quantification of PC by liquid chromatography-diode array fluorescence detection (LC-DAD-FLD) according to Ferreyra et al. [18].
LC-DAD-FLD analysis
PC separation and quantification were performed on a Dionex Ultimate 3000 (Dionex Softron GmbH, Thermo Fisher Scientific Inc., Germering, Germany) equipped with a vacuum degasser unit, an autosampler, a quaternary pump, a chromatographic oven, a diode-array [Dionex DAD-3000 (RS)] and a dual-channel fluorescence detector (FLD-3400RS Dual-PMT) connected in series. The software Chromeleon 7.1 was used to control all the parameters of the system and to process the obtained data.
The chromatographic separation was achieved on a reversed-phase Kinetex C18 column (3.0 mm × 100 mm, 2.6 μm; Phenomenex, Torrance, CA, USA) and as mobile phases aqueous solutions of 0.1% formic acid (solvent A) and acetonitrile (solvent B). The separation gradient was the following: 0–1.7 min, 5% B; 1.7–10 min, 30% B; 10–13.5 min, 95% B; 13.5–15 min, 95% B; 15–16 min, 5% B; 16–19, 5% B. The total flow rate was set at 0.8 mL/min and the column temperature at 35°C. The injection volume was 5 μL for standards and 1 or 2.5 μL (depending on the estimated concentration of compounds and fluorescence intensity of flavanols and stilbenes) for bunch stem and cane extracts. The analytical flow cell for DAD was set to scan from 200 to 400 nm. A data collection rate of 5 Hz, a bandwidth of 4 nm, and a response time of 1.000 s were used. Wavelengths of 254 nm, 280 nm, 320 nm, and 370 nm were used depending on the targeted analytes for DAD, while an excitation wavelength (Ex) of 290 nm and a monitored emission (Em) responses of 315, 360, and 400 nm were used depending on the targeted analytes for FLD [18]. A data collection rate of 10 Hz, peak width of 0.04 min corresponding to a response time of 0.8 s and a photomultiplier gain of 5 units, was used for FLD.
PCs were identified by comparing the retention times of the samples with those of authentic standards, and quantified by external calibration with pure standards.
Cell lines and culture conditions
In vitro studies were performed on human cell lines: HCT-116, colorectal carcinoma, and HBL-100, non-tumoral epithelium (American Type Culture Collection, ATCC). Cell lines were cultured in DMEM supplemented with 10% FBS, 100 IU penicillin, and 100 μg/mL streptomycin. Cells were cultured at 37°C, in a humidified atmosphere enriched by 5% CO2.
The treatment time selected was based on the doubling time of each cell line to allow the control cells to complete at least two full cell cycles. Treatments were performed for 48 h in both cell lines in addition to the culture media in a dose-response experimental design.
MTT cytotoxicity assay
A colorimetric assay using 3-(4,5-di-methylthiazol-2-yl)-2,5-diphenyl tetrazolium bromide (MTT) was performed as was described previously [19] and is schematized in Figure 1. HCT-116 and HBL-100 cells were seeded in 96-well microplates (3–5 × 103 cells/well/100 μL); 24 h later, the medium was aspirated and replaced by supplemented culture medium (control) or extracts (treatment) at concentrations ranging from 0 to 4,000 µg/mL in both cell lines. The cells were then incubated for 48 h, after which the medium was replaced with 100 µL of MTT solution (0.5 mg/mL in RPMI-1640 without phenol red or FBS) and incubated in the dark for a further 4 h. MTT solution was then removed and 100 µL of DMSO was added; the plates were shaken for 15 min to dissolve the formazan crystals. Optical density (OD) was measured using a Thermo Scientific Multiscan Elisa reader at 570 nm. The OD obtained in untreated cells (controls) was taken as 100% viability. From the absorbance data, the percentage viability was calculated using the following equation:
Where DOx and DOc represent the test and control OD, respectively. The results were expressed graphically by means of a dose-response curve. Assays were performed three times in triplicate. The cytotoxic potential was expressed in terms of IC50, corresponding to the extract concentration that inhibited 50% of cell growth.
Statistical analysis
Data were analyzed using GraphPad Prism software, version 8.0.2. One-way ANOVA followed by the Tukey test (P < 0.05) was used. A sigmoidal dose-response analysis was performed to evaluate the IC50. Values were considered acceptable if the goodness of fit was R2 ≥ 0.90. Assays were performed in triplicate and results are expressed as mean ± standard error.
Results
Cane and bunch stem extracts PCs profile
A total of 23 PCs from different phenolic sub-classes were quantified in the extracts. These included non-flavonoids (hydroxybenzoic and hydroxycinnamic acids, stilbenes, and phenyl ethanol analogs) and flavonoids (flavanols, flavanones, and flavonols). Table 1 presents the results of individual PC from Vitis vinifera L. cv. Malbec cane and bunch stem extracts. For cane extract, stilbenes (51%), followed by flavanols (28%) and hydroxycinnamic acids (18%) were the predominating PC constituents. The PC with the highest concentration were ɛ-viniferin (1,041 μg/g dw), followed by (+)-catechin (541 μg/g dw), trans-piceid (533 μg/g dw), and caffeic acid (417 μg/g dw) (Table 1). Figure S1 presents the resulting chromatograms with the quantified PCs obtained after the analysis of cane and bunch stem extracts by LC-DAD-FLD.
Levels (μg/g dw) of PCs quantified in cane and bunch stem by-products
Phenolic compound | Cane | Bunch stem |
---|---|---|
Hydroxybenzoic acids | ||
Gallic acid | 30 ± 1 | 33 ± 1 |
Syringic acid | n.d. | 154 ± 4 |
Total | 30 ± 1 | 187 ± 5 |
Hydroxycinnamic acids | ||
Caftaric acid | 253 ± 7 | 2,283 ± 65 |
Caffeic acid | 417 ± 8 | 331 ± 4 |
Total | 669 ± 15 | 2,614 ± 69 |
Stilbenes | ||
trans-piceid | 533 ± 11 | 284 ± 3 |
trans-piceatannol | 60 ± 2 | n.d. |
trans-resveratrol | 69 ± 1 | n.d. |
ε-viniferin | 1,041 ± 33 | 89 ± 1 |
Pteroestilbene | 161 ± 5 | 168 ± 4 |
Total | 1,864 ± 52 | 541 ± 8 |
Flavanols | ||
Procyanidin B1 | 72 ± 1 | 1,015 ± 32 |
(+)-catechin | 541 ± 9 | 2,421 ± 90 |
Procyanidin B2 | 53 ± 1 | 70 ± 2 |
(–)-epicatechin | 175 ± 6 | 49 ± 1 |
(–)-epigallocatechin gallate | 110 ± 1 | 248 ± 12 |
(–)-gallocatechin gallate | 88 ± 1 | 105 ± 1 |
Total | 1,040 ± 19 | 3,907 ± 138 |
Flavanones | ||
Astilbin | n.d. | 1,391 ± 22 |
Naringenin | 72 ± 3 | n.d. |
Total | 72 ± 3 | 11,391 ± 22 |
Flavonols | ||
Quercetin 3-β-D galactoside | 7 ± 0.2 | 30 ± 1 |
Quercetin 3-β-D-glucosidoe | n.d. | 11 ± 0.1 |
Kaempferol-3-glucoside | n.d. | 31 ± 1 |
Myricetin | n.d. | 93 ± 4 |
Total | 7 ± 0.2 | 164 ± 6 |
Other compounds | ||
OH-tyrosol | n.d. | 348 ± 8 |
Tyrosol | n.d. | 12 ± 0.6 |
total | n.d. | 360 ± 9 |
Total PC | 3,682 ± 90 | 9,163 ± 257 |
n.d.: not detected. PCs: phenolic compounds. Results are expressed as the mean ± standard deviation (SD) (n = 3 replicates)
In bunch stem extract, flavanols (43%) and hydroxycinnamic acids (29%) reported the highest levels. In detail, (+)-catechin (2,421 μg/g dw), caftaric acid (2,283 μg/g dw), and astilbin (1,391 μg/g dw) were the main compounds (Table 1). This matrix is mainly rich in flavanols whose majority components are (+)-catechin, followed by (−)-epicatechin [5, 8].
Cytotoxicity effects on cell lines
The in vitro cytotoxicity of the cane and bunch stem extracts on the human cell lines HCT-116 and HBL-100 were evaluated by MTT (Tables 2 and 3, entries 6, respectively). Cane extract did not exhibit cytotoxicity effect in any of the cell lines studied in a dose-response manner for the range of concentrations tested (250–4,000 μg/mL) (Figure 2A and B). The cytotoxic response of this extract showed statistical significance for the type of cell-cultured (P < 0.05), but not for the concentration factor.
Summary of in vitro cytotoxicity studies of cane extracts effects on different types of cancer
Entry | Method | Variety | Pre-conditions | Extraction conditions | Yields | Assay | Pre-treatment | Dose (µg/mL) | Treatment time (h) | Cell line | Major findings | Reference(s) | |
---|---|---|---|---|---|---|---|---|---|---|---|---|---|
Stilbene a | Flavanols b | ||||||||||||
1 | PLE (SWE) | Tinta Roriz (TR), Touriga Nacional (TN), Alvarinho, Loureiro | Dried (50°C in oven) for 24 h, powdered < 1 mm | Water 40 mL/g, 150°C,40 min, 4 MPa. | 186 µg/g dw (TN), 195 µg/g dw (TR) | 3,102 µg/g dw (Loureiro), 2,565 µg/g dw (TN) | MTT | Lyophilized and filtered 0.45 µm Millex GV filters | 0.1–1,000 | 24 |
| Σ phenolic acids > Σ flavanols > Σ stilbenes; ↓cell viability of HaCaT: 24.4% (TR) and 28.8% (Loureiro) 1,000 µg/mL; ↓cell viability of HFF-1: < 7.6% (TR) for > 1 µg/mL, 58.8% (TN)–74.8% (Loureiro) for 1,000 µg/mL. | [20] |
2 | CSLE | Mixture Merlot and Cabernet Sauvignon | Dried (in open air) for 2 months, powdered | Acetone: H2O (6:4, v/v), 5 mL/g, rt, 12 h (2 times), stirring. Filtered, evaporated organic solvent, lyophilized. Washed with water in an Amberlite XAD-7 column, eluted with acetone (55 g/kg stilbene-enriched extract). | 45.4% (w/w) | - | MTT, NR | Stock solution DMSO 0.5%, 1,000 µg/mL | 0–100 | 24–48 |
| ε-viniferin (16.34%, w/w) > trans-resveratrol (8.07%, w/w); ↓cell viability of HepG2: ≥ 30–40 µg/mL (24/48 h), EC50 (MTS): 31.2 (24 h) and 20.6 µg/mL (48 h); ↓cell viability of Caco-2: ≥ 30–40 µg/mL (24/48 h), EC50 (MTS): 55.8 (24 h) and 39.0 µg/mL (48 h). | [12] |
3 | CSLE | Riesling | Cut into pieces, dried (rt) | MeOH:H2O:HCl 1N (80:19:1, v/v/v), 25 mL/g, rt, 2 times (1st: 60 min, 2nd: 30 min), stirring. Filtered (0.2 μm), evaporated organic solvent, and washed with water in an Amberlite XAD16N resin, eluted with EtOH 90%. Evaporated organic solvent and lyophilized. | 12,000 µg/g dwc | AB | Stock solution DMSO ≤ 1.0% | 5–500 | 2–24 | HepG2 | ↓cell viability of HepG2: < 80% for ≥ 200 µg/mL. | [15] | |
4 | OH | Loureiro | Dried (rt), powdered < 8 mm | EtOH:H2O (45:55, v/v), 40 mL/g, 60 min, 80°C, IEF (840 V/cm). Filtered, evaporated organic solvent, lyophilized. | 654 µg/g dwc | - | MTT | Stock solution DMSO 0.5% | - | 24–48 |
| Apigenin > quercetin > ellagic acid > hesperidin; IC50: 49.7–89.7 μg/mL (48 h). | [17] |
5 | UAE | Pinot Noir | Stored 3 months (rt), cut into pieces (1 cm) | EtOH:H2O (80:20, v/v), 10 mL/g, 100 min, 80°C. Evaporated organic solvent. | 6.3% (w/w) | 1.11% (w/w) | MTT | Stock solution DMSO ≤ 1.0% | 0–100 | 72 |
| IC50: 9.6–57.5 μg/mL. | [16] |
6 | UAE | Malbec | After pruning (1 day), cut into pieces (2–4 cm), dried (60°C) until a constant weight. Powdered and stored (rt) | EtOH:H2O (50:50, v/v), 50 mL/g, 60°C, 50 Hz, 1 h. | 1,864 µg/g dw | 1,111 µg/g dw | MTT | Evaporated organic solvent and filtered 0.22 µm. Stock solution 40 mg/mL | 0–4,000 | 48 |
| HBL-100: no toxicity, HCT-116: no toxicity. | (This work) |
a: Σ trans-resveratrol + Ɛ-viniferin; b: Σ (+)-catequin + (–)-epicatechin; c: µg/g dry extract; ↓: decreased regarding a control disease group. CSLE: conventional solid-liquid extraction; UAE: ultrasound assisted extraction; PLE: pressurized liquid extraction; SWE: subcritical water extraction; OH: ohmic heating; rt: room temperature; dw: dry weight; MTT: 3-(4,5-di-methylthiazol-2-yl)-2,5-diphenyl tetrasodium bromide; NR: neutral rep uptake; AB: Alamar Blue® (resazurin); IC50: half maximal inhibitory concentration. Human colon (Caco-2 and HCT-116), breast (MDA-MB-231 and MCF-7), liver (HepG2), gastric (AGS), lung (SK-MES-1), bladder (J82) cancer cell lines. Normal human colon (CCD841 CoN), lung (MRC-5), epithelium (HBL-100), dermal (HFF-1 and HaCaT) cell lines a: Σ trans-resveratrol + Ɛ-viniferin;
Summary of in vitro cytotoxicity studies of bunch stem extracts effects on different types of cancer
Entry | Method | Variety | Pre-conditions | Extraction conditions | Yields (µg/g dw) | Assay | Pre-treatment | Dose (µg/mL) | Treatment time | Cell line | Major findings | Reference(s) | |
---|---|---|---|---|---|---|---|---|---|---|---|---|---|
Stilbene a | Flavanols b | ||||||||||||
1 | CSLE | Mazuelo | Dried (25°C) until constant weight, powered < 0.3 mm | EtOH:H2O (50:50, v/v), 100 mL/g, 24 h, 40°C, stirring (150 rpm). Centrifuged, filtered, lyophilized. | 850c | 980c | SRB | Stock solution 1.5 mg/mL | 62.5–100 | 48–72 |
| IC50: 203–817(MCF-7), 85–911 (MDA-MD-231), 661–759 (Caco-2), 1,454 (fibroblast cells) μg/mL | [11] |
2 | CSLE | Tinto Cão, Tinta Barroca, Malvasia Fina, and Moscatel Branco | Washed, cut into small pieces, dried (40°C) for 72 h, powered | MeOH:H2O (70:30, v/v), 37.5 mL/g, vortexed and agitated 30 min, rt (3 times), filtered (0.45 µm). | 2,940–5,820 | - | AB | - | 0.002–0.500%d | 36 | HaCaT | No cytotoxic effects | [10] |
3 | CSLE Soxhlet | Chardonay | Cut into small pieces | Extracted with H2O (4 mL/g) under reflux for 1 h, filtered and concentrated. Loaded into Amberlite XAD-1180N resin, eluted with EtOAc and MeOH. Evaporated MeOH fraction, chromatographed over Sephadex LH-20 (100 mL) eluting with 30% MeOH, 40% EtOH, 30% acetone, 40% acetone, and 60% acetone sequentially. Purified fraction five over Toyopearl HW40F (63 mL) eluting with 30% MeOH, 40% EtOH, and 60% acetone sequentially. Fraction three was evaporated to yield a light brown powder. | - | - | - | Newly isolated proanthocyanidin | 0–50e | 48 |
| Significant cytotoxic activity; insolated new compound > epigallocatechin 3-gallate | [21] |
4 | UAE | Assyrtiko, Vinsanto, Voidomato, Mandilaria, Mavrotragano, Athiri, Moschato, Ksinomavro, Vilana | Air-dried, powdered, and stored (rt) | MeOH:H2O:HCl 1N (90:9.5:0.5, v/v/v), 4 mL/g, 10 min, rt (3 times). Evaporated organic solvent, dissolved 30 mL MeOH:H2O (1:1, v/v) and centrifuged, extracted Petroleum Ether (3 × 30 mL) and concentrated. Re-suspended 30 mL brine, extracted EtOAc (4 × 30 mL). Combined organic layers dried, dissolved MeOH (1 mg/mL), filtered (0.45 µm) | 7.78–69.64c | 9.48–98.29c | XTT | - | 1–200 | 24 |
| HepG2: IC50 50 μg/mL, HeLa: IC50 32 μg/mL | [9] |
5 | UAE | Hambourg Muscat, Assyrtiko, Voidomato, Mavrotragano | Air-dried, powdered, and stored (rt) | MeOH:H2O:HCl 1N (90:9.5:0.5, v/v/v), 4 mL/g, 10 min, rt (3 times). Evaporated organic solvent, dissolved 30 mL MeOH:H2O (1:1, v/v) and centrifuged, extracted Petroleum Ether (3 × 30 mL) and concentrated. Re-suspended 30 mL brine, extracted EtOAc (4 × 30 mL). Combined organic layers dried, dissolved MeOH (1 mg/mL), filtered (0.45 µm) | 9,090–25,410c | 13,610–31,310c | SRB | Stock solution DMSO ≤ 1.0%, 40 mg/mL. Sterilized via filtration (0.22 μm) | 12.5–400 | 72 |
| IC50: 121–230 (MCF-7), 121–184 (MDA-MD-23), 175–309 (HT29), 159–314 (K1), 180–225 (786-0), 134 to > 400 (Caki-1) μg/mL | [13] |
6 | UAE | Malbec | Lyophilized, powered | EtOH:H2O (50:50, v/v), 50 mL/g, 60°C, 50 Hz, 1 h. | 541 | 5,298 | MTT | Evaporated organic solvent and filtered 0.22 µm. Stock solution 40 mg/mL | 0–4,000 | 48 |
| HBL-100: no toxicity, HCT-116 IC50: 680 µg/mL | (This work) |
a: Σ trans-resveratrol + Ɛ-viniferin; b: Σ (+)-catequin + (–)-epicatechin; c: µg/g dry extract; d: culture media supplemented (× 10−3%) with polyphenolic extracts; e: mM. CSLE: conventional solid-liquid extraction; UAE: ultrasound assisted extraction; rt: room temperature; dw: dry weight; MTT: 3-(4,5-di-methylthiazol-2-yl)-2,5-diphenyl tetrasodium bromide; NR: neutral rep uptake; AB: Alamar Blue® (resazurin); SRB: sulforhodamine B; IC50: half maximal inhibitory concentration. ↓: decreased regarding a control disease group. Human colon (Caco-2, HT-29, HCT-116, and Lovo), breast (MDA-MB-231 and MCF-7), liver (HepG2), cervix (HeLa), renal (786-0 and Caki-1), thyroid (K1), prostate (PC-3) cancer cell lines. Normal human epithelium (HBL-100), dermal (HaCaT) cell lines
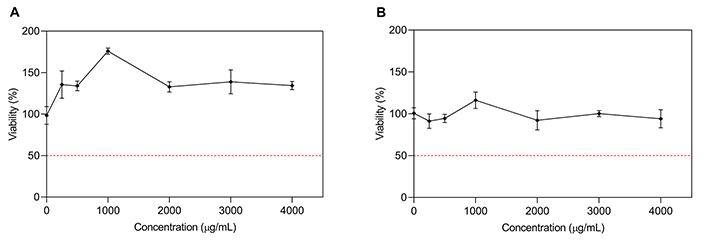
Dose-response curve for cane extract on HBL-100 (A) and HCT-116 (B), respectively. Results are expressed as % viability of control (untreated cells) vs. extract concentration (µg/mL). Curves represent mean values ± standard error (n = 3)
Comparing the action of bunch stem extract on both cell lines, an inhibitory effect was observed on HCT-116 tumor cells, but it did not affect the viability of non-tumor HBL-100 cells (Figure 3A and B). Statistical analysis showed a significant difference in the effect of the extract depending on the type of culture and the concentration (P < 0.05). This would indicate a selective cytotoxic action on cancer cells, while at the same time protecting and stimulating the growth of healthy ones. As observed in Figure 3B, bunch stem extract showed a dose-dependent cytotoxic effect on HCT-116 and an IC50 of 680 µg/mL after 48 h of incubation.
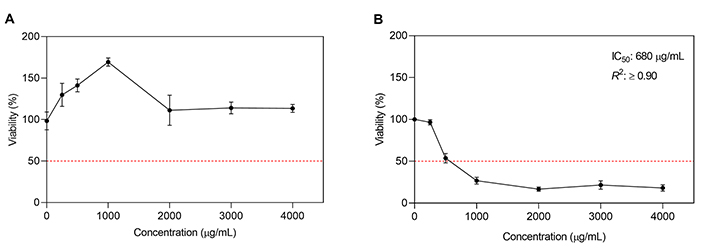
Dose-response curve for bunch stem extract on HBL-100 (A) and HCT-116 (B), respectively. Results are expressed as % viability of control (untreated cells) vs. extract concentration (µg/mL). Curves represent mean values ± standard error (n = 3)
Discussion
In the last few years, several studies have been carried out on the valorization of grape canes [5, 8], although only some of these studies have assessed the cytotoxic activity of the obtained extracts [12, 15–17, 20]. Most studies show high concentrations of stilbenes trans-resveratrol and ε-viniferin. However, the quantification varies depending on the cultivar, the growing conditions, and the extraction conditions used. Sáez et al. [16] reported a 6.3% of stilbenes in the total composition of a Pinot Noir cane extract [Table 2, entry (5)]. The insolated stilbenes were mainly ε-viniferin (3.5%) and trans-resveratrol (1.7%). Medrano-Padial et al. [12] obtained an extract enriched in stilbene (45.4%, w/w) from a mixture of Merlot and Cabernet Sauvignon [Table 2, entry (2)]. ε-viniferin (16.3%) and trans-resveratrol (8.1%) were also the majority stilbene compounds. Moreira et al. [20] showed the same qualitative composition of the cane extracts obtained from different varieties by subcritical water extraction (SWE), but the levels of flavanols were higher than stilbenes [Table 2, entry (1)]. Fuchs et al. [15] prepared a PC-rich extract from cv. Riesling cane and the content was expressed on dry extract (12,000 µg/g) [Table 2, entry (3)]. Instead, Jesus et al. [17] informed 654 µg/g of the total stilbene level from freeze-dried cane extract (cv. Loureiro) [Table 2, entry (4)]. On the other hand, bunch stem has been extensively characterized for its PCs [5, 8]. Only a few studies have evaluated its in vitro cytotoxic potential [9–11, 13, 21]. In terms of phenolic content, this matrix is mainly rich in flavanols. The main components are (+)-catechin, followed by (–)-epicatechin, and procyanidins. Quercetin derivatives were the main flavonols found. Phenolic acids were reported, mainly caftaric and gallic. The literature also reported lower concentrations of stilbenes, with ε-viniferin followed by trans-resveratrol being the most common. In Table 3, flavanols have been reported with concentrations between 9.48 and 31,310 µg/g of dry extract, followed by stilbenes with a concentration range from 7.78 to 25,410 µg/g of dry extract [9, 11, 13]. Domínguez-Perles et al. [10] reported levels of stilbenes (ε-viniferin) between 2,940 and 5,820 µg/g dw from different varieties, and also other PCs (data not shown). Sei-ichi et al. [21] separated and characterized the isolated compounds from the grape stem, focusing this study on the proanthocyanidins. A number of factors explain the variability in the concentration of individual PCs, although there is an overall trend in the PCs profiles of these grapevine by-products. Some of them are reported in the literature, e.g., genetic background, environmental growth conditions, vineyards’ management, winemaking practices, pretreatment/storage of residuals, and the extraction/characterization procedures used [5, 8, 22].
This study, unlike those cited, is the first to evaluate the cytotoxic effect of an extract from pruning Vitis vinifera L. cv. Malbec on human non-tumoral breast epithelial cells (HBL-100) and colorectal adenocarcinoma (HCT-116). Therefore, a direct comparison with the rest of the studies is not possible, despite the fact that some have used colon cells but from other cell lines (Caco-2 and CCD 841 CoN) [12, 17]. However, several reports demonstrated that pruning extracts generate a cytotoxic action on various cell lines at the in vitro level (Table 2) [12, 15–17, 20]. Moreira et al. [20] found no adverse effects on dermal cell lines (HaCaT and HFF-1) treated with cane extracts from different varieties at concentrations below 100 and 1,000 μg/mL, respectively [Table 2, entry (1)]. However, for concentrations higher than 1 µg/mL from Tinta Roriz (Dão Region), the cell viability of human fibroblasts (HFF-1) was reduced to < 7.6%. In contrast, Medrano-Padial et al. [12] reported a concentration- and time-dependent decrease in the growth of two cell lines (HepG2 and Caco-2) when exposed to a cane extract rich in stilbenes (45.4%, w/w) [Table 2, entry (2)]. This extract reduced cell viability in the concentration range of 30 to 100 μg/mL after 48 h of exposure in both cell lines. Likewise, Fuchs et al. [15] also reported dose- and time-dependent cytotoxic effects on HepG2 for an extract from this matrix [Table 2, entry (3)]. When using a concentration of 500 μg/mL, cell viability was reduced by 50% after 24 h of incubation. Jesus et al. [17] reported the inhibitory action of cane extracts on the growth of four cancer cell lines (MDA-MB-231, MCF-7, HepG2, Caco2) and one non-cancer line (CCD 841 CoN) [Table 2, entry (4)]. The range of IC50 values was 49.7–89.7 μg/mL for the 48 h treatment. The authors hypothesized that these results could be attributed to the abundant presence of PC such as apigenin, quercetin, ellagic acid, and hesperidin in the extracts, which have reported the ability to induce apoptosis and inhibit the progression of human cancer cell lines. Sáez et al. [16] also evaluated the antiproliferative activity, but of oligostilbenes isolated from pruning residues, in four cell lines (MRC-5, AGS, SK-MES-1, and J82) [Table 2, entry (5)]. trans-resveratrol presented the best IC50 value for the MRC-5 and AGS lines, while trans-piceatannol was more effective for the SK-MES-1 and J82 lines. In addition, they evaluated the crude extract, obtaining IC50 values of 57.5, 23.3, 45.0, and 9.6 μg/mL for MRC-5, AGS, SK-MES-1, and J82, respectively.
In recent years, the ability of PCs to modulate (prevent or induce) apoptosis, a mechanism of programmed cell death, has been tested by monitoring the specific ability to respond to inducing factors such as oxidative stress [23]. Using mainly in vitro experiments, PCs have been tested in cancer and normal cells. These studies provide experimental evidence for the selective apoptosis of malignant cells, while the cell viability of normal cells was not affected by these compounds [24]. This apoptotic selectivity has been demonstrated with quercetin, by inhibiting the growth of prostate cancer tumor cells, while it did not affect normal cells (PC-3 and DU-145 cell lines, respectively). Domínguez-Perles et al. [10] reported an antiapoptotic effect from a bunch stem extract on HaCaT cells [Table 3, entry (2)].
A number of studies have pointed out the anticancer potential of bunch stem extracts [23]. Apostolou et al. [9] have suggested the use of bunch stem extracts as cytotoxic agents, given their ability to inhibit the growth of liver and cervix cancer cell lines [Table 3, entry (4)]. The mean IC50 values for HepG2 and HeLa cancer cells were 50 ± 12 and 32 ± 16 μg/mL, respectively. In another study, Sahpazidou et al. [13] demonstrated anticancer potential of bunch stem extracts of Greek varieties against colon, breast, kidney, and thyroid cancer [Table 3, entry (5)]. IC50 values were: 121–230 (MCF-7), 121–184 (MDA-MD-23), 175–309 (HT29), 159–314 (K1), 180–225 (786-0), and 134 to > 400 (Caki-1) μg/mL. Quero et al. [11] studied the effect on cell viability of a bunch stem extract on the Caco-2, MCF-7, and MDA-MB-231 cancer lines, and the results showed that it was capable of inducing cell death by apoptosis [Table 3, entry (1)].
The success in in vitro cytotoxic results of the majority of stem-derived extracts is related to their PCs content and profile. Although the relative amounts of individual PCs in extracts derived from this matrix may vary due to various factors mentioned above, they stand out for their abundant content of flavonoids and phenolic acids. Some PCs in the extract are highly reported as active compounds against cancer cells, though the potential synergism between them and other chemically different compounds present in the extracts should not be forgotten [13, 25]. For example, the most potent bunch stem extract against all cancer cell lines tested, except 786-0, was characterized by the highest content of gallic acid and trans-resveratrol (Voidomato variety) [13]. Proanthocyanidins have shown inhibition of cancer cell proliferation, and epicatechin pentamers and hexamers were reported to inhibit cancer cell invasive activities [21]. trans-resveratrol has reported the ability to inhibit carcinogenesis at multiple stages (initiation, promotion, and progression) [25, 26]. The in vitro antiproliferative and proapoptotic effects of trans-resveratrol in tumor cell lines have been widely documented [27, 28]. In the treatment of colorectal cancer, this compound has been effective, causing a 70% inhibition of CaCo-2 cell growth. In in vivo trials, trans-resveratrol has shown efficacy in the prevention and treatment of tumors of the skin, esophagus, intestine, and colon [4]. Oral administration of high doses of trans-resveratrol in potable water and diet reduces the incidence of tumors in mice. However, its short half-life and low bioavailability have made its use difficult [28], limiting its efficacy to tumors with which it may come into direct contact (e.g., skin or gastrointestinal tract cancers). Although serum concentrations may be relatively low, local concentrations at the site of action may be sufficient to exert a pharmacological effect. On the other hand, trans-resveratrol together with other PC, such as quercetin and (+)-catechin, have exhibited synergies that could overcome their scarce bioavailability [26]. By way of an example, a freeze-dried extract of natural red wine promoted differentiation and reduced the proliferation of HCT-116 colon cancer cells [27]. trans-piceatannol, reported antiproliferative activity against hepatocellular cancer cells and suppressed metastasis of these cells in rats [25]. Meanwhile, pterostilbene inhibited in vitro the proliferation of pancreatic cancer cells. OH-tyrosol, also present in the extract, has shown a wide range of antitumor effects, inhibiting proliferation and promoting apoptosis in various human tumor cell lines through various mechanisms [26].
Data confirming the in vitro bioactivity of PC usually use the pure compounds or their mixtures in (primary) plant extracts. Therefore, their bioavailability and biotransformation after ingestion is a parameter that not considered. That is, in vitro micromolar concentrations may not be operative in vivo. Hence, it is hard to draw reliable conclusions about any “real” health-promoting properties of PCs, knowing that they may have low bioavailability and extensive metabolism. Biological activity and bioavailability, are rarely investigated at the same time, so the bioactivity of their derived metabolites, present in human blood and tissues, are almost completely unknown [29]. It is not possible to obtain meaningful insight into biological effects without counting on sufficient evidence about the uptake of specific PCs in humans when exposed to cultured cells. Except when these compounds can go into direct contact with cells without undergoing uptake or metabolism [30]. So, this supports the idea of investigating the in vivo effects of PCs and/or their extracts on colon or skin cancer cells. Synergistic and/or additive effects of PC could be increased by their encapsulation with conventional anticancer therapies or their combination with other PC. In this context, future research that advances the bioavailability of PCs will be relevant, as well as the study of its metabolites, which facilitate the extrapolation of the results to in vivo studies first in animal models. Subsequently, clinical studies will be needed to explain the in vitro/vivo findings. This will help to understand the potential benefits of these extracts and their active compounds in patients with cancer.
The current study reports the content of PCs of cane and bunch stem extracts Vitis vinifera L. cv. Malbec and their in vitro cytotoxicity in human tumor and non-tumor cell lines. Cane extract did not inhibit cell proliferation at the in vitro level for the tested lines, which is an advantage for its potential application as a functional or nutraceutical agent. On the other hand, the bunch stem extract showed cytotoxic activity against the human tumor line HCT-116, representative of colorectal adenocarcinoma, with selective and significantly lower effects on non-tumor cells. Colorectal cancer is the third leading cancer in the world. There is great interest in the development of new chemopreventive products and/or anticancer agents from natural and sustainable sources such as agro-industrial residues. This disease, due to its condition in the intestinal lumen, does not necessarily require the intestinal absorption of the active principles to exert its antitumor function. Importantly, this is the first time that the cytotoxic effect of Vitis vinifera L. cv. Malbec bunch stem and cane extracts were assessed in the HBL-100 and HCT-116 cell lines. This information reinforces the importance of further investigation of these extracts as potential preventive and/or therapeutic phytoderivatives that could lead to valuable biological effects in targeted cells without causing toxic side effects in normal cells. Likewise, a potential alternative can be opened for its combined use with traditional drugs with the aim of reducing treatment doses or preventing their toxicities, especially in detoxification organs such as the liver and kidneys. Additional studies are needed to gain a deeper knowledge of the molecular mechanisms involved, the effects and the bioavailability of the compounds (and/or their metabolites) in target tissues.
Abbreviations
dw: | dry weight |
FBS: | fetal bovine serum |
LC-DAD-FLD: | liquid chromatography coupled to diode array and fluorescence detectors |
MTT: | 3-(4,5-di-methylthiazol-2-yl)-2,5-diphenyl tetrazolium bromide |
PCs: | phenolic compounds |
Supplementary materials
The supplementary figures for this article are available at: https://www.explorationpub.com/uploads/Article/file/101049_sup_1.pdf.
Declarations
Acknowledgments
The authors are also grateful to Roy Urvieta for providing the samples.
Author contributions
SF: Conceptualization, Methodology, Investigation, Formal analysis, Data curation, Writing—original draft, Writing—review & editing. ASL: Methodology, Formal analysis. CGL: Conceptualization, Methodology, Formal analysis, Investigation, Resources, Data curation, Supervision, Funding acquisition, Writing—review & editing. AC: Supervision, Writing—review & editing. AF: Conceptualization, Methodology, Formal analysis, Investigation, Resources, Data curation, Visualization, Supervision, Project administration, Funding acquisition, Writing—review & editing.
Conflicts of interest
The authors declare that they do not have any conflict of interest.
Ethical approval
Not applicable.
Consent to participate
Not applicable.
Consent to publication
Not applicable.
Availability of data and materials
Data can be shared on reasonable request.
Funding
This work was supported by ANPCyT [Grant number: BID PICT 2017-2325 and BID PICT 2020-1367] and SIIP-UNCuyo A016-T1 to AF. Additionally, we received the support from ANPCyT [PICT-2019-2019-03791] and SIIP-UNCuyo [06/J019-T1] to CGL. SF have a CONICET scholarship. ASL have an INC-Argentina scholarship. AC, CGL, and AF are fellows of CONICET. The funders had no role in study design, data collection and analysis, decision to publish, or preparation of the manuscript.
Copyright
© The Author(s) 2024.