Abstract
The etiology of recurrent spontaneous abortion (RSA) is extremely complex, as there are 40–50% of patients with unexplained miscarriages, known as unexplained RSA (URSA). URSA affects approximately 1–2% of females of childbearing age and has a massive impact on the physical and mental conditions of both patients and their families. The pathogenesis of the disease remains unclear, making its treatment complicated. In recent years, considerable progress has been made in the exploration of the URSA immune balance mechanism and it has been universally acknowledged that a balanced immune response (as abnormal immunity) may be the root cause of poor pregnancy outcomes. This review discussed and summarized the effects of immune cells and blocking antibodies (BAs) on URSA based on the current state of knowledge in this area. Additionally, molecular genetics also plays an essential role in the incidence rate of URSA since the role of genetic polymorphism in the pathogenesis of URSA has been thoroughly studied. Nonetheless, the outcomes of these studies are inconsistent, particularly across populations. This paper reviewed previous studies on URSA and maternal genetic polymorphism, focusing on and synthesizing the most important findings to date, and providing diagnostic recommendation for URSA patients with clinical symptoms.
Keywords
Unexplained recurrent spontaneous abortion, immune cells, gene polymorphismIntroduction
Recurrent spontaneous abortion (RSA) is a disorder distinct from infertility, identified as two or more spontaneous abortions [1], with an incidence rate of 1–5% [2]. The etiology of RSA is multifaceted and complex, involving primarily advanced maternal age, hereditary genetic abnormalities, anatomical factors, infection, and endocrine dysfunction [3]. Nonetheless, in over 50% of patients with RSA, the cause of the miscarriage remains unknown and is recognized as unexplained RSA (URSA).
In recent years, more and more studies have been concerned about the role of immune factors in the pathogenesis of URSA. Wu et al. [4] proposed that URSA is induced by the disruption of maternal immune homeostasis, and natural killer (NK) cells, T cells, macrophages, and dendritic cells (DCs) at the maternal-fetal interface contribute to the maintenance of maternal immune homeostasis throughout pregnancy. The imbalance of immune cells at the maternal-fetal interface is very similar to URSA. Recent studies have demonstrated that the proportion of decidual natural killer (dNK) subsets decrease while the activated DCs subsets increase in URSA patients, at the same time decidual cluster of differentiation CD8+T cells exert cytotoxicity [5]. In addition, the balance of M1/M2 macrophages is disordered at the maternal-fetal interface in URSA patients manifested by the dominance of M1 macrophages. Ultimately, all these unfavorable immune factors result in abortion [6].
The study of maternal genetic polymorphism and URSA traces back to the year 1993 when Obata [7] first studied human leukocyte antigen (HLA)-DR gene polymorphism in the Japanese population. Normal pregnancy is inseparable from the precise regulation between the fetus and the mother, in which pregnancy-related mediators such as cytokines, hormones, and angiogenic factors play a critical role in maintaining normal pregnancy status. Therefore, it is presumed that genetic polymorphisms associated with these mediators are associated with URSA risk [8].
URSA is an essential cause of secondary infertility, and yet its etiology and mechanism have not been fully clarified, which makes URSA one of the most pressing and challenging points in clinical research. Immune factors are considered to be responsible for 60% of unexplained RSA, with autoimmune recurrent abortion being more prevalent. This is primarily due to the abnormal recognition of fetal paternal antigen by the mother, which results in the loss of maternal blocking antibody (BA) [9]. Paternal lymphocyte immunotherapy is an effective treatment for unexplained RSA due to the fact that it is attributed to the production of anti-paternal cytotoxic antibodies, anti-idiotypic antibodies, and mixed lymphocyte response blocking factor (MLR-Bf) during the pregnancy of URSA females [10].
This paper discussed the immune factors at the maternal-fetal interface and the mechanism of BAs that lead to URSA, as well as the common genetic polymorphisms associated with the media of URSA patients and reviewed the potential genetic markers of URSA.
Immunological factors
The immunological events of bilateral communication between mother and fetus during early gestation are complex, and a successful pregnancy requires an accurate immunological dialogue at the maternal-fetal immune interface of the endometrium [11]. The maternal-fetal interface consists of maternal-derived decidua and placenta, and approximately 40% of the decidua is formed by maternal leukocytes [12]. Human decidua leukocytes in early gestation are composed mainly of NK cells (∼70%) and macrophages (∼20%), with a proportion of approximately 10–20% T cells, and DCs, while B cells and NKT cells are rare [13] (Figure 1A). As the pregnancy advances, the number of metaphase NK cells and DCs does not change much in mid-gestation, with a gradual increase in the number of T cells and a gradual decrease in the number of macrophages [14]. As it progresses to late gestation, T cells become the largest immune cell population and the proportion of both macrophages and NK cells decreases [15]. There is an imbalance in the ratio of immune cells at the maternal-fetal interface in URSA patients, which is closely associated with the development of URSA (Figure 1B).
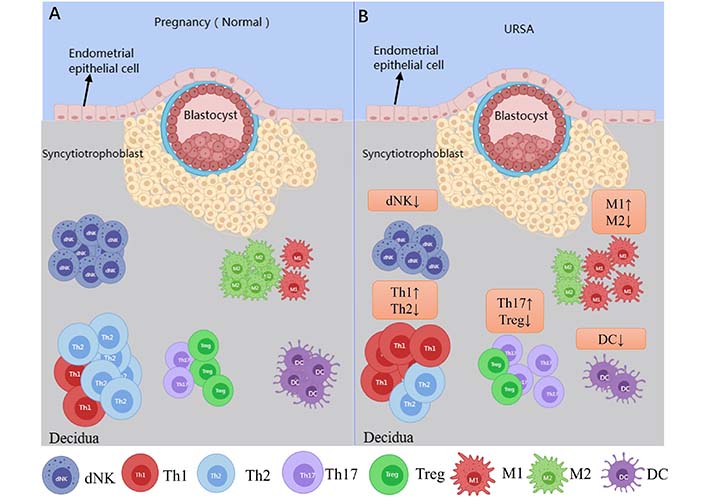
Immune cells at the maternal-fetal interface in normal pregnant women and URSA patients. Picture A shows the immune cells associated with the maternal-fetal interface in normal pregnant women; picture B shows aberrations in the immune cells at the maternal-fetal interface in URSA patients, which is characterized by the decrease in dNK cells, T helper 2 (Th2) type of T cells, regulatory T cells (Tregs), M2 macrophage and DC as compared to normal pregnant women. Whereas an increase in the Th1 immune response, Th17 cells, M1 macrophage lineage are observed in URSA patients as compared to normal pregnant women. ↑: increase; ↓: decrease
NK cells
During the first trimester of pregnancy, dNK cells accumulate and become the dominant leukocyte population, then decline and only 40–50% dNK cells remain by late gestation [15, 16]. dNK cells can secrete interferon γ (IFN-γ) to activate angiogenesis-related gene expression, promote helical artery remodeling, and also stimulate vascular growth directly through the production of vascular endothelial growth factor (VEGF) and placental growth factor in decidua tissue. Besides, dNK cells can also exhibit low cytotoxicity relative to peripheral NK cells [2]. All of the above suggest that dNK cells are essential for the maintenance of early pregnancy.
In the trophoblast-independent stage of uterine artery remodeling, dNK cells surround the spiral artery and synthesize a series of soluble factors to induce extravillous trophoblast invasion and cause vascular structural instability, including interleukin-8 (IL-8), IFN-γ induced protein-10 (IP-10, also known as C-X-C motif chemokine ligand 10), granulocyte-macrophage colony-stimulating factor, PLGF, IFN-γ, tumor necrosis factor (TNF)-α, VEGF, angiopoietin-1/2 and matrix metalloproteinases-2/9 [17]. Among them, IFN-γ regulates trophoblast invasion and maintains the integrity of the decidua [18]. IFN-γ produced by dNK cells induces upregulation of long non-coding RNA maternally expressed gene 3 expressions in vascular smooth muscle cell (VSMC), and both the treatment of dNK cells or IFN-γ and the overexpression of long non-coding RNA maternally expressed gene 3 can inhibit VSMC proliferation and stimulate its migration and apoptosis, leading to impaired uterine spiral artery remodeling [19]. Moreover, dNK cells can regulate trophoblast invasion by binding chemokine IP-10 and IL-8 with C-X-C motif chemokine receptor 1 and C-X-C motif chemokine receptor 3 expressed by invasive extravillous trophoblast [20]. The decreased expression of CD276 in the trophoblast of URSA patients impairs the inhibition function of IL-8 and IP-10 secretion by dNK cells and maintains the microenvironment of excessive inflammation [21]. A study comparing dNK cells from age-matched healthy controls and URSA patients found lower levels of CD49a and higher expression of perforin, granzyme B, and IFN-γ in the URSA patients [22]. From the above-mentioned studies, it is clear that dNK cell dysfunction is closely related to uterine spiral artery reconstruction (Figure 2).
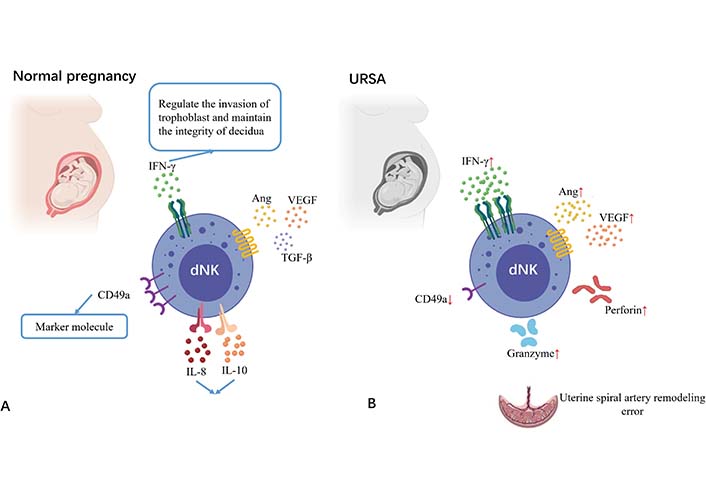
Changes of NK cells at the maternal-fetal interface in URSA patients compared with normal pregnant women. Picture A shows the decidual NK cells at the maternal-fetal interface in a normal pregnant woman; picture B shows the decidual NK cells at the maternal-fetal interface in URSA patients. Decreased expression of CD49a, over expression of perforin, granzyme B, IFN-γ, angiopoietin, and VEGF are observed in URSA patients as compared to normal pregnant women. TGF-β: transforming growth factor-β. ↑: increase; ↓: decrease
According to the production of cytokines, human NK cells are divided into four types, including NK1, NK2, NK3, and NKr1 subgroups. Among them, NK1 generates IFN-γ and TNF-α, NK2 excretes IL-4, IL-5 and IL-13, NK3 cells produce TGF-β, and NKr1 cells secrete IL-10 [23]. In URSA patients, the NK1/NK2 ratio of dNK cells (producing IFN-γ/IL-4 NK cell ratio) increased, and it was independent of whether the karyotype was normal. NKp46 is a receptor on NK cells, which is involved in cytotoxicity and cytokine production [24]. A study showed that in women with low %NKp46+ dNK, the ratio of NK1/NK2 was significantly higher than that of women with high %NKp46+ dNK, and the threshold of %NKp46+ dNK cells was 86.52% [25]. On the whole, the above research suggests that URSA is associated with the interference effect of NK cells on the uterine spiral artery remodeling and the imbalanced proportion of NK cells in the decidua, which may result in the inflammatory state of the micro-environment of the maternal-fetal interface. The expression of NKp46 may help predict this type of URSA, providing a possible detection indicator for its diagnosis.
T cells
Among leukocytes at the maternal-fetal interface, CD4+T cells can be activated and then differentiate into Th1, Th2, and Th17 cells to produce the corresponding types of cytokines. Th1 cells are differentiated by IL-12-driven, and Th2 cells are differentiated by IL-4-driven, and factors other than differences in cytokine environments may also selectively affect Th1/Th2 development [26]. Th17 is a distinct Th cell population that primarily produces IL-17A, and activated Th17 cells also secrete IL-6, IL-17F, IL-21, IL-22, TNF-α, and granulocyte-macrophage colony-stimulating factor which induce inflammatory responses by promoting the recruitment of inflammatory cells [27]. Tregs are a subset of CD4+T cells expressing high levels of CD25 and the transcription forkhead box protein P3 (Foxp3), and Tregs are essential for maintaining immune homeostasis and preventing autoimmunity by suppressing auto-reactive T cells [28]. T cells play a central role in regulating the immune response. In early gestation, the maternal-fetal interface is a Th1 cell environment that promotes blastocyst implantation. In mid-gestation, the maternal-fetal interface converts to a Th2 cell environment, as this period is the main stage of fetal development and an anti-inflammatory immune microenvironment is necessary for fetal growth. Finally, in late gestation, the maternal-fetal interface switches back to a Th1 cell-dominated immune state, which is vital for delivery [29]. The T cell homeostasis at the maternal-fetal interface of URSA is shown in Figure 3.
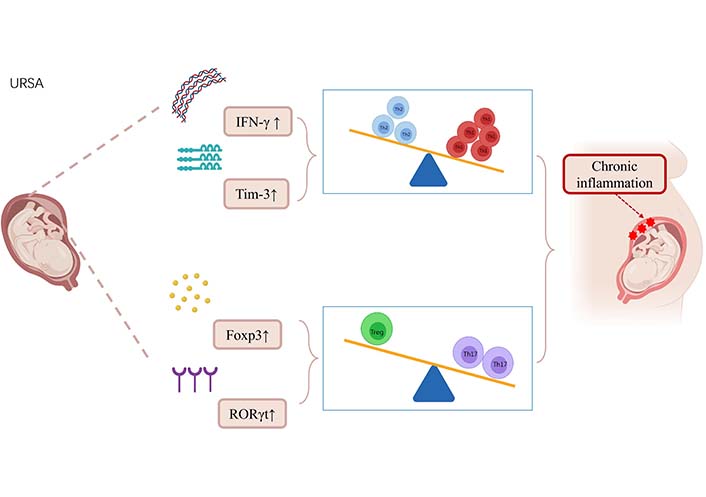
Alterations in T-cell homeostasis in URSA patients leading to chronic inflammation. The pro-inflammatory cytokine IFN-γ secreted by Th1 cells in URSA patients was upregulated by IFN-γ. T-cell immunoglobulin and mucin domain-3 (Tim-3), a subtype of the Tim protein family, was upregulated. These changes result in a shift of Th1/Th2 balance toward Th1 balance at the maternal-fetal interface in URSA patients. In URSA patients, the expression of retinoic acid-related orphan receptor γt (RORγt) related to Th17 cell expression was upregulated, and the increased expression of FoxP3 mRNA and protein led to the shift of Treg/Th17 balance to Th17 balance at the maternal-fetal interface. T-cell immune dysregulation at the maternal-fetal interface in URSA patients leads to chronic inflammation and promotes URSA. ↑: increase
Th1/Th2
Th1 cells secrete IFN-γ, TNF-β, IL-2, and TNF-α. Th1-type cytokines activate macrophages and mediate related cell responses, which plays an important role in resistance to intracellular pathogen infection, cytotoxicity, and delayed-type hypersensitivity. Th2 cells secrete IL-4, IL-5, IL-6, IL-10, and IL-13, which are usually associated with strong antibody responses. Th1 and Th2 cells inhibit each other. For instance, IL-10, a product of Th2 cells, inhibits the development of Th1 cells by acting on antigen-presenting cells, while IFN-γ, a product of Th1 cells, prevents the activation of Th2 cells [30].
Studies have shown that the Th1/Th2 ratio balance is critical for the maintenance of pregnancy and current studies in this area were conducted mainly using maternal peripheral blood [31, 32]. Elevated Th1 cytokines, such as IFN-γ and TNF-α, have been observed in women with URSA, and Th1 cells prevent trophoblast growth and differentiation through pro-inflammatory responses [33]. Gu et al. [34] studied clinically normal women and patients with URSA by using targeted RNA sequencing and found IFNG, the gene that encodes the pro-inflammatory cytokine IFN-γ secreted by Th1 cells, was upregulated in the URSA patient group, suggesting that the main cause of Th1/Th2 imbalance is an increase in Th1. In animal studies, Tangri et al. [35] demonstrated that Th1-type cytokines, such as IFN-γ and TNF-α, are also highly expressed in the placenta of pregnant mice with a propensity to miscarriage. Luo et al. [36] also used the mouse model to prove that the embryo absorption rate of the RSA group was higher than that of the control group, and the higher concentration of Th1 cytokine (IFN-γ) corresponds to a higher level of the transcription factor T-bet expression, while a lower level of Th2 cytokine (IL-4) expression corresponds to a lower level of gata binding protein 3 (GATA-3) expression in RSA mice. This research implied that RSA involves the up-regulation of T-bet and the down-regulation of GATA-3. It is known that T-bet can activate Th1 development, and GATA-3 is crucial for Th2 development [37]. Therefore, the ratio of T-bet/GATA-3 theoretically can be used as a marker to evaluate the Th1/Th2 immune balance in the diagnosis of RSA.
In recent years, interest has been focused on the study of the Tim-3. Tim-3 is a subtype of the T-cell immunoglobulin structural domain and Tim protein family, which is selectively expressed on Th1 cells and can downregulate Th1 responses through galactose glucose-9 involved in transducing apoptotic signaling, and Tim-3 dysregulation is associated with the pathogenesis of RSA [38]. A study by Wu et al. [39] found abnormally elevated levels of soluble Tim-3 (sTim-3) and galactose glucose-9 expression in patients with URSA, suggesting that sTim-3 blocks the binding of Tim-3 to galactose lectin-9 and inhibits the Tim-3/galactose lectin-9 propagation signal. However, it should be noted that clinical validation of the Th1/Th2 cell balance is currently available mainly in peripheral blood, and studies of the Th1/Th2 balance at the maternal-fetal immune interface are more limited and need further validation.
Tregs/Th17 cells
Th17 cells and Tregs are different subtypes of T cells that are differentiated from initialized T cells under the synergistic stimulation of multiple cytokines. Many studies have linked Th17 cells to pathogenic effects in allograft rejection, suggesting the important role of Th17 responses in negative pregnancy outcomes. During pregnancy, Tregs are essential for maintaining maternal immune tolerance to a hemizygous fetus [29]. A study by Wang et al. [40] defined the enrichment of Th17 cells in peripheral blood and decidua in URSA patients for the first time and confirmed an inverse relationship between Th17 cells and Tregs. The Th17/Treg ratio during healthy pregnancy favors Tregs, however, in patients with URSA (increased Th17 levels and decreased Treg levels), the balance between Th17/Treg is disturbed and the inflammatory immune response is not adequately regulated [41]. Thus, the Treg/Th17 balance at the maternal-fetal interface is closely related to pregnancy outcome.
Tregs function in part by secreting the anti-inflammatory cytokines IL-10 and TGF-β1, where TGF-β1 can promote Foxp3 expression by inducing Treg differentiation with CD4 CD25. Th17 cells play a key role in the development of autoimmune and allergic reactions by producing IL-17 and expressing RORγt [42, 43]. HLA-G interacts with CD4+T cells through specific receptors directly or through DCs indirectly. It initiates the CD4+T cell stimulation cascade during the alloimmune response and supports the growth of diverse Tregs [44]. The latest research shows that the unique phenotypic memory Tregs (mTregs) play an important role in the maintenance of pregnancy. Compared to the normal pregnancy group, the expression of programmed cell death protein 1 (PD-1) in the URSA group is lower, and both the activation of T cells and the highly expressed C-C motif chemokine receptor 6 in Tregs are reduced, resulting in a reduction in the expression of PD-1’s inhibition of immune response and finally causing the occurrence of URSA [45].
In URSA patients, the mean intensity of TGF-β and Foxp3 expression was significantly reduced [46, 47]. Lipocalin is a novel negative T-cell regulator that induces immune tolerance to grafts [48]. Li et al. [49] showed that recombinant lipocalin promotes a shift in serum cytokine polarization from Th17 to Tregs. Furthermore, it can enhance Foxp3 mRNA and protein expression and reduce RORγt expression in the maternal-fetal interface of abortion-prone mice by activating the p38 mitogen-activated protein kinase (MAPK)-signal transducer and activator of transcription 5 (STAT5) signaling pathway. Therefore, it indicates that an imbalance of Th17 and Tregs in patients with URSA causes an excessive inflammatory response, which triggers URSA, and key factors such as Foxp3 and RORγt also take part in the process.
Macrophages
Macrophages, as antigen-presenting cells, account for 20–30% of leukocytes at the maternal-fetal interface [50]. Macrophages are involved in the complex regulation of the maternal-fetal interface through the regulation of secreted cytokines, phagocytosis, and immune homeostasis. In fact, they not only affect vascular remodeling but also are actively associated with trophoblast invasion and pregnancy maintenance [51]. Macrophages can be classified into classically activated (M1) and alternatively activated (M2) subtypes according to their cytokine production and function [52]. In early pregnancy, uterine macrophage populations with an alternatively activated steady-state phenotype are involved in embryo implantation and placental development as well as in the prevention of placental infection in both mice and humans [53]. During the implantation phase, the M1 type predominates, while later it changes to a mixed population of M1 and M2 types, which takes part in trophoblastic invasion, infection resistance, and tissue vascular remodeling [54]. The mixed M1/M2 population persists until mid-pregnancy, and then decidua macrophages shift to an M2-dominant phenotype after completion of placental development, which promotes maternal immune tolerance to the semi-carcinogenic fetus and protects fetal growth until delivery [55]. As labor approaches, decidua M2-like macrophages become more abundant, and they may have an immunomodulatory role before term pregnancy [56]. Research shows that CD206+M2-like MΦs may be essential for embryo implantation through the regulation of endometrial proliferation via Wnt/β-catenin signaling [57]. Consequently, an appropriate proportion of M1/M2 has a vital impact on successful pregnancies. Altered or disturbed M1/M2 balance and related functions during pregnancy may lead to complications and imbalance in the M1/M2 ratio is strongly associated with RSA [58].
A study by Tsao et al. [58] found that M1 macrophages were abundant in the decidua of spontaneous abortion and URSA, while M2 macrophages were significantly more frequent in the endometrium of luteal phase and normal pregnancy, suggesting that M2 polarization is important for successful early human pregnancy. Fetal-maternal tolerance is mediated, at least in part, by the a2 isoform of vacuolar ATPase, an important molecule expressed at the fetal-maternal interface. In the placenta of a mouse model prone to abortion, reduced expression of the a2 isoform of vacuolar ATPase may bias the polarization pattern toward the M1 phenotype and induce inflammation [59]. In placental tissues from URSA patients, peroxisome proliferator-activated receptor γ (PPARγ) expression is significantly downregulated. As it has been confirmed that PPARγ is essential as a nuclear receptor for the maturation of alternatively activated M2 macrophages, the reduced level of PPARγ results in a decreased level of M2 differentiation [60]. Besides, reduced PD-1 expression on decidual macrophages accompanied by reduced programmed cell death-ligand 1 (PD-L1) expression on placental villi was observed in URSA permutations, in which PD-1/PD-L1 axis disorders induce M1 differentiation [61]. The M1/M2 balance in URSA patients is shifted towards the M1 type, which secretes a large amount of pro-inflammatory cytokines (e.g., TNF-α, IL-6, and IL-1β) [62]. URSA patients are found to have abnormally high levels of TNF-α, which leads to the downregulation of stathmin-1, and ultimately by regulating E-calmodulin/ β linked protein pathway promotes the development of URSA [63]. Disturbance of the PD-1/PD-L1 axis at the maternal-fetal interface in URSA patients promotes macrophage polarization toward the M1 type, which may further promote inflammatory cytokine secretion and increase the risk of URSA development (Figure 4).
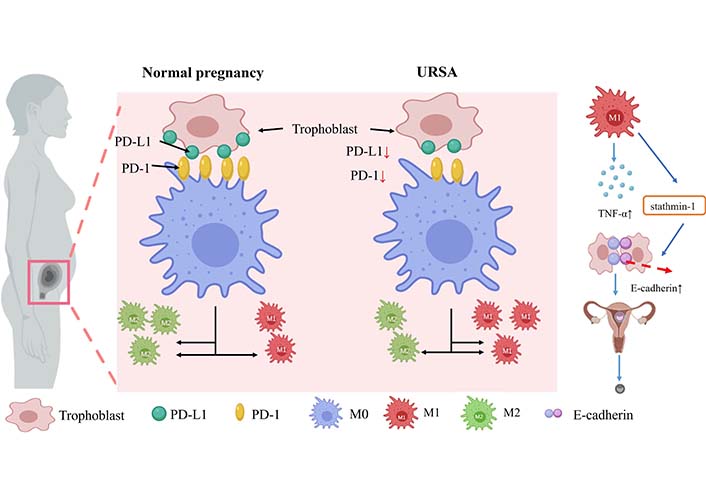
Macrophage expression and secretion in normal females and URSA patients. During URSA seed exchange, PD-1 protein expression on decidual macrophages decreased, followed by PD-L1 presentation on placental villi. PD-1/PD-L1 axis disorder induced M1 differentiation. M1 secretes a large number of pro-inflammatory cytokines such as TNF-α in patients with URSA. The increased levels of TNF-α down-regulate stathmin-1 through E-cadherin-β catenin pathway, which may promote the occurrence of URSA. ↑: increase; ↓: decrease
In addition, current studies suggest that the pathogenic process of URSA may be associated with changes in microRNA (miRNA) expression profiles in decidua and villi [64, 65]. Zhu et al. [66] showed that downregulated miR103 enhances M1 polarization via the signal transduction and activator of transcription 1 (STAT1)/interferon regulatory factor 1 (IRF1) pathway. Also, Ding et al. [67] found that miR-146a-5p and miR-146b-5p of extracellular vesicles secreted by M1 macrophages inhibited epithelial-mesenchymal transition in trophoblast cells by directly suppressing TNF receptor-associated factor 6 expression after transcription, thereby increasing the risk of URSA. The above studies reveal that related miRNA targets may be effective diagnostic markers for URSA and can possibly serve as novel therapeutic targets by regulating M1/M2 homeostasis.
DCs
DCs are important players in linking innate and adaptive immunity [68]. DCs have a dual role as they can differentiate into potent antigen-presenting cells to further activate effector T cells and in an immature state they can enhance immune tolerance by inducing the production of Tregs [69]. In vivo, most DCs are in an immature state [immature DCs (imDCs)]. ImDCs process antigens through DEC+205 receptors and promote CD8+T cell proliferation while inhibiting cytotoxic IFN γ production, which means that imDCs mainly maintain their immune tolerance. In contrast, mature DCs (mDCs) can upregulate class 1 and class 2 major histocompatibility complex (MHC) molecules with binding peptides on their surface. Through presenting binding peptides to T cell receptors, mDCs ultimately induce specific T cell activation and secretion of IL-12, leading to Th1 differentiation of CD4+T cells which may promote URSA formation [70]. Moreover, it was found that mDCs increased and imDCs decreased in URSA patients, suggesting that the immature state of DCs facilitates pregnancy while mDCs may play an important role in the pathogenesis of URSA [71]. Lai et al. [72] showed that DCs in RSA patients and RSA mice were characterized by less expression of CD274, significantly higher serum levels of IL-12 and IL-6 than in the normal pregnancy group, and decreased expression of TGF-β and IL-10, thus allowing T cells to balance Th1/Th2/Th17/Treg subsets to Th1/Th17. Changes in the expression of these functional molecules and cytokine secretion by DCs may disrupt the balance of maternal and fetal immune tolerance status and lead to URSA, so the mechanism of DCs’ role in URSA patients is closely related to T cell differentiation.
BAs
BAs, also known as anti-husband lymphocyte antibodies, are a class of immunoglobulin G (IgG) antibodies produced mainly against the embryonic HLA Il and trophoblast lymphocyte cross-antigens. One view is that early URSA may be related to the patient’s low response to embryonic and semi-allogeneic antigens and inability to produce appropriate BAs, which ultimately leads to embryonic rejection [73]. BAs mainly include anti-HLA-D/DR antibodies, MLR-Bf, and anti-paternal cytotoxic antibodies, etc. [74, 75].
Pandey et al. [76] found that MLR-Bf is essentially IgG-3. It is present in pregnant women and women with URSA who successfully became pregnant after immunotherapy treatment. A clinical study by Nonaka et al. [77] observed that after paternal lymphocyte immunotherapy in URSA patients (BA negative), the pregnancy success rate in BA negative patients was lower (30%) than that in BA positive patients (75%). Khonina et al. [78] also proved that MLR-Bf-negative URSA women had increased proliferative cell responses to paternal alloantigens and enhanced production of soluble inhibitory activity factor (MLR-Bf) after they received paternal lymphocytes immunotherapy, which contributes to improved pregnancy outcomes. Most women with alloimmune causes of URSA have increased sharing of HLA and significantly lower titers of MLR-Bf, which may be associated with preventing the mother from making anti-paternal cytotoxic antibodies, while paternal lymphocyte immunotherapy can increase blocking antibody expression [10]. In conclusion, the above-mentioned studies suggest an increased risk of URSA in women with negative closed antibodies, and the conversion of closed antibodies from negative to positive in URSA patients by lymphocyte immunotherapy would decrease the incidence of URSA. Therefore, closed antibody testing could also be used as a criterion for determining URSA.
Maternal gene polymorphisms
Cytokines, hormones, and angiogenic mediators are necessary for normal pregnancy and embryonic development. However, the differences in specific expression quantity, pregnancy stage, and expression location can affect the interaction of trophoblast and endometrium, which may lead to URSA and other pregnancy complications [79]. Many gene polymorphisms have been reported to play a crucial role in the pathogenesis of URSA. According to different gene functions, gene polymorphisms can be divided into different systems for description.
Cytokines gene polymorphism
Cytokines are cell signaling molecules with a vital role as immune regulators in pregnancy, regulating gametogenesis, implantation, and fetal development [2]. After normal pregnancy implantation, Th1/Th2 is essential for maintaining pregnancy at the maternal-fetal interface, in which Th1 cells play a pro-inflammatory role by secreting TNF-α, IFN-γ, IL-17, and IL1-β while Th2 cells promote anti-inflammatory effects by producing IL-4, IL-6, IL-10, and IL-1RA [80, 81].
In URSA patients, TNF-α gene polymorphism is studied most widely. TNF-α-308G/A and/or -238G/A are two common sites in research. A meta-analysis by Zhang et al. [82] showed that TNF-α-308G/A and -238G/A polymorphisms were not significantly associated with the risk of RSA in the whole population. However, the studies of Zhao X and Liu C indicated that TNF-α-238G/A is significantly associated with URSA risk in Asian populations [83, 84]. Lee et al. [85] reported similar findings in the Korean population and proposed that TNF-α-1031t>C may also be a risk factor for URSA.
Among all these related genes, the IL-6 gene is the most extensively studied anti-inflammatory gene polymorphism in URSA patients, and IL-6-174G/C is the most common research site. However, all studies have failed to prove the association between this specific polymorphism and RSA [79]. One study investigated the relationship between RSA and the single nucleotide polymorphism of -634C→G in the promoter region of the IL-6 gene in the Japanese population. The results showed that there was a significant difference in the -634C→G genotype frequency (CC and CG/GG) between URSA patients and the control group, and the risk of URSA in G allele carriers was lower than that in wild-type (CC) women [86]. Ma et al. [87] also reported that IL-6-634C/G polymorphism may be a genetic protective factor of URSA in the Chinese population.
HLA gene polymorphism
Located on chromosome 6, HLA is characterized by a wide range of polymorphisms [88]. HLA coding genes are human MHC, which have become the focuses of reproductive immunity [89]. HLA can be divided into HLA class 1 and HLA class 2. Classical HLA-A, -B, and -C genes have a high degree of polymorphism, while nonclassical HLA-E, -F, and -G genes show decreased polymorphism. Class 2 genes encode five different molecules, among which HLA-DP, -DQ, and -DR are polymorphic, while HLA-DM and HLA-DO exhibit reduced polymorphism and function in regulating other HLA class 2 molecules [90].
At present, most of the studies on this aspect focus on the relationship between HLA-G, HLA-D, and URSA, while few pieces of literature study the relationship between HLA-I (A, B, C) and URSA.
Non-classical HLA Class 1 (HLA-G and HLA-E)
Being a kind of non-classical HLA class 1 molecule, HLA-G is mainly expressed in extravillous trophoblast (EVT) cells, exerting immune regulation, anti-inflammation, and other functions, and can inhibit cellular immunity at the maternal-fetal interface [91]. Yazdani et al. [92] analyzed the HLA-G gene haplotype and found that the H1 haplotype of the HLA-G gene would reduce the risk of URSA, while the H2 haplotype increased the risk. Compared with the H1 haplotype, the risk of URSA in women carrying the H2 haplotype increased by 142%. Several polymorphisms have been confirmed in a non-coding region of the HLA-G gene, including polymorphisms in the 3’ untranslated regions (UTR) which affect the expression of the HLA-G gene. The specific 3’ UTR haplotype of HLA-G was associated with 2 consecutive times of URSA, but not with 3 or more times of URSA in women [93]. Among polymorphism in the 3’ UTR of the HLA-G gene, the 14base pair (bp) insertion/deletion (I/D) is the most extensively studied [94]. A meta-analysis by Fan et al. [95] revealed that 14bp I/D polymorphism variants in the HLA-G gene were associated with the risk of URSA in patients with three or more abortions. Another meta-analysis by Monti et al. [91] found that the risk of URSA was increased by 56.2% in the European population carrying the HLA-G gene 14bp insertion/insertion genotype, but there was no correlation between the HLA-G gene 14bp I/D genotype and the occurrence of URSA. This study also found that there was no relation between the HLA-G gene 14bp I/D genotype and URSA in women who had two or more URSA and three or more URSA.
HLA-E is also a kind of non-classical MHC class 1 antigen. Being expressed on trophoblast cells during pregnancy together with HLA-G, HLA-E promotes maternal tolerance to semi-allogeneic fetuses by binding to inhibitory receptors on NK cells [96]. However, currently there are few pieces of research on the correlation between HLA-E gene polymorphism and URSA. Fotoohi et al. [97] investigated the HLA-E gene locus of Iranian women and found that women carrying HLA-E 0101/0103 heterozygous genotypes had a 73% higher risk of URSA compared with the general population.
Classical HLA Class 1 (A, B, C)
Currently, not many literature reports on HLA Class 1 exist. Shankarkumar et al. [98] studied the HLA-A, B, and C allele subtypes of Indian URSA patients and found that HLA-A*030301, HLA-B*5701, and HLA-Cw*12021 were susceptibility genes of URSA, and HLA-A*2402, HLA-B*4006, and HLA-Cw*15021 were protective genes. The study by Silva et al. [99] conducted in the Brazilian population showed that the frequency of the HLA-A*34 allele was higher in URSA patients, and the frequency of HLA-A*24 and HLA-B*35 was significantly lower in URSA patients. Killer-cell immunoglobulin-like receptors bind to HLA ligands on the surface of target cells, which are closely related to viral infection, malignant tumors, autoimmune diseases, organ transplantation, and pregnancy [100]. The HLA-C gene has a high degree of polymorphism, and these alleles can be divided into two allotypes, namely C1 and C2. Of the two groups, HLA-C2 positive women are more likely to experience URSA [101].
HLA-D gene
The expression of HLA in maternal-fetal interface tissues plays a key role in the success of pregnancy and fetal development, and the immune deficiency associated with HLA-DQ/DR region leads to the dysfunction of the immunosuppressive mechanism during pregnancy [102, 103]. Bompeixe et al. [104] found that the genotypes HLA-DQB1*02:02 and 03:01 significantly decreased and the allele HLA-DRB1*11:04 increased in RSA patients. A meta-analysis demonstrated that HLA-DRB1*4 and HLA-DRB1*15 were susceptibility genes of RSA, while HLA-DRB1*13 and HLA-DRB1*14 were protective genes [105]. Hviid and Christiansen [106] discovered that HLA-DR3, DQA1*0501, and DQB1*0201/0202 were all associated with RSA.
The above literature reports on the polymorphism of HLA alleles vary due to differences in sample size, race, region, and other factors. Therefore, the research in this area should include more experimental samples to confirm the association between HLA genes and URSA.
Thrombophilia-associated gene polymorphism
MTHFR and MTRR
The methylenetetrahydrofolate reductase (MTHFR) enzyme plays a key role in processing amino acids, especially in converting homocysteine to methionine. The mutation of the MTHFR gene may cause an enzyme deficiency, leading to a slightly increased level of homocysteine, especially in individuals with folate deficiency [107]. At present, MTHFR C677T and A1298C are closely related to URSA in both internal and external research, while only a few studies focus on methionine synthase reductase (MTRR) A66G. The balance between coagulation and fibrinolysis is an important part of early pregnancy, and thrombosis is believed to be one of the causes of recurrent abortion. MTHFR and MTRR are two key enzymes in folate metabolism, whose abnormal activity may cause increased metabolic levels of homocysteine and decreased levels of folate, bringing about the inadequate synthesis of fetal DNA and protein. Moreover, hyperhomocysteinemia may result in vascular endothelial injury and affect the balance between coagulation and fibrinolysis, which ultimately causes URSA [108, 109].
A meta-analysis by Du et al. [110] suggested that there were some differences in the association between MTHFR C677T polymorphism and RSA, especially in the Asian population, but no significant correlation was found between A1298C and RSA. A meta-analysis by Wang et al. [111], which analyzed research conducted only in Chinese women, showed that the MTHFR 677T allele, 677TT genotype, and MTHFR 1298CC genotype were all related to URSA. However, Hwang et al. [112] did not observe significant differences in the genotype distribution of MTHFR C677T and A1298C polymorphisms between recurrent pregnancy loss (RPL) patients and controls in the case-control study of Korean women. Mazokopakis and Papadomanolaki [113] found that MTHFR C677T gene polymorphism was related to RPL in the population study of Greek women, while A1298C had no such correlation. Studies of Syrian women showed that RPL women in Syria with homozygous genotypes (C677T and A1298C) had a high risk of abortion, regardless of whether they were single or multiple heterozygous genotypes. A meta-analysis by Yang et al. [114] manifested that not only are the maternal and paternal MTHFR gene C677T and A1298C polymorphisms related to RSA, the fetal MTHFR A1298C polymorphism is also significantly associated with RPL. The differences in the results of the above studies may be attributed to ethnic variances and the choice of major case-control studies. Systematic meta-analyses included different ethnic groups, which showed that MTHFR C677T and A1298C are closely related to URSA, while the results varied from race to race. Since MTHFR and MTRR are closely related to folate synthesis, the decision to supplement folate and the supplemental doses of folate should be made on a case-by-case basis during pregnancy determined by the patient’s circumstances.
ACE (I/D) and PAI-1 (4G/5G)
Plasminogen activator inhibitor 1 (PAI-1) is a single-chain glycoprotein. As a major inhibitor of fibrinolysis, PAI-1 exerts a vital role in the regulation of the fibrinolysis system [115]. The level of PAI-1 in the plasma of healthy pregnant women gradually increased in the second trimester of pregnancy and reached the maximum at 32–40 weeks of pregnancy. Within 5–8 weeks after delivery, the level of PAI-1 gradually decreased to the levels before pregnancy [116]. The 4G and 5G alleles of PAI-1 are expressed in a codominant way. Individuals who were homozygous of 4G have the highest plasma PAI-1 level, while heterozygous individuals showed an intermediate level, and 5G homozygous individuals displayed the lowest PAI-1 level [117]. As an effective vasopressor, the angiotensin-converting enzyme (ACE) converts angiotensin I to angiotensin II and is a key component of the renin-angiotensin system [118]. The level of ACE varies among different genotypes of ACE intron 16 I/D polymorphism. The ACE D allele can raise the PAI-1 level through increasing PAI-1 4G allele expression, which leads to fibrinolysis inhibition and thrombosis, thereby causing URSA [8].
A meta-analysis reported that ACE I/D polymorphism had an obvious correlation to RSA, showing that PAI-1 4G/5G had high clinical heterogeneity but did not have an association with RSA [119]. Another meta-analysis concluded that the frequencies of 4G/4G and 4G/5G alleles in RSA patients were higher, which was more obvious in Asian and African groups [120]. Studies also pointed out that ACE I/D and PAI-1 4G/5G polymorphisms were associated with RSA in Iranian and Polish women [121, 122]. Compared with the non-European population, the frequency of 4G alleles in the European population is higher. However, among women from European countries, only in Czech and Bulgarian women was a statistically strong association found between 4G alleles and RSA [123]. In general, the correlation between ACE I/D and PAI-1 4G/5G polymorphisms and URSA is significantly different among ethnic groups, thus further subgroup research should be carried out and the sample size should be expanded.
Placental angiogenesis-associated gene polymorphism
Angiogenesis is an important step in chorionic villus formation. Thrombin-activatable fibrinolysis inhibitor (TAFI) regulates fibrinolysis and inflammation. VEGF, endothelial nitric oxide synthase (eNOS), and thrombin can activate genetic polymorphisms of TAFI, leading to the occurrence of URSA [124].
VEGF-1154G/A (rs1570360), +936C/T (rs3025039), -634G/C (rs2010963), and -583T/C (rs3025020) polymorphisms are found to be associated with URSA susceptibility [125]. Interestingly, a study conducted in a specific population suggested that VEGF-1154G/A polymorphism was irrelevant to the URSA susceptibility of Chinese Han women [126]. However, the GA genotype of VEGF-1154G>A polymorphism was found to have a remarkable difference between URSA patients and the control group in the Korean population [127].
The 27 bp variable number tandem repeat (VNTR) polymorphism in intron 4 and Gly298Asp polymorphism in exon 7 of eNOS seem to affect nitric oxide production and are relevant to different pregnancy diseases [128]. There was a strong relationship between eNOS Glu298Asp polymorphism and URSA in East Asians, while in Caucasians and East Asians, no correlation was observed between eNOS intron 4 VNTR polymorphism and unexplained RPL [129]. In contrast, the 4 VNTR polymorphism in Northern Indian women was found to be associated with URSA [130].
Pruner et al. [131] studied the TAFI+1040C/T allele and found that compared with the T allele, women who carried the C allele had a 9% lower risk of URSA, though the results were not statistically significant. In addition, two studies pointed out that TAFI+1040C/T could not be used as a predictor of URSA in Egyptians [124, 132]. However, the data of Fang et al. [133] implied that the TAFI+1040C allele has a protective effect against URSA and may be related to its genetic susceptibility.
Hormone-associated gene polymorphism
As the most important steroid hormone in the female reproductive system, estrogen plays a variety of roles in the uterus to ensure successful pregnancy and conception, including cervical maturation, embryo implantation, and placental formation. The physiological function of estrogen is mediated by two estrogen receptors, namely estrogen receptor 1 (ESR1) and ESR2, which are expressed in various tissues of the female reproductive system. Studies have shown that estrogen receptors may induce immune tolerance at the maternal-infant interface, indicating that ESR is a key factor in the pathogenesis of URSA [134, 135].
Among research on ESR1, Yin et al. [136] reported that ESR1 rs9340799 (-351A/G) polymorphism may have diverse roles in RSA in different ethnic populations. For Asians, ESR1 rs9340799(-351A/G) polymorphism may reduce the risk of RSA, while for non-Asians it may increase the risk. Tang et al. [135] found that ESR1 gene polymorphisms rs9340799, rs2234693, and rs3798759 were not related to URSA in Chinese Han and Hui populations. Another study conducted on Tunisian and Egyptian women showed there was a significant association between the ESR1 variant and the increased risk of RSA [137, 138]. However, no significant association was found between ESR2 gene polymorphism and URSA [138–140].
It is well known that genetic research is complex and needs further confirmation in different environments. Also, ethnic differences may interfere with genotype frequency, thus affecting the research results. The above results show that the relationship between gene polymorphism in different media and the risk of URSA is closely related to different ethnic groups and different environments. It is worth noting that most of the above studies are limited by regions and races, and the sample size is also insufficient. In this regard, a large number of sample studies need to be conducted in different regions and populations to further explore the relationship between gene polymorphism in different media and URSA.
Conclusions
URSA can lead to secondary infertility, and bring great harm to patients’ bodies and minds, and the limited understanding of the pathogenesis pose new challenges for the clinical diagnosis and treatment of URSA. At present, some achievements have been made in the research on the etiology of URSA, but the specific pathogenesis of URSA has not been fully elucidated so far. By reviewing and synthesizing the existing literature, this article summarizes the associated etiologies of URSA. The balance of the proportion of immune cells at the maternal/fetal interface, such as NK1/NK2, Th1/Th2/Treg/Th17, and M1/M2, was closely related to URSA. However, one limitation is that at present, only the content of immune cells in peripheral blood can be detected, and the proportion of immune cells at the mother-to-fetus interface cannot be detected. Therefore, it is important to develop more effective detecting methods of URSA. According to current studies, relevant markers can be used as detection markers to predict the balance of immune cells at the mother-to-fetus interface. For example, it is mentioned that the %NKp46 dNK ratio can represent the maternal NK1/NK2 cell ratio, and thus %NKp46 dNK may be possibly used as a marker in the future to diagnose patients with URSA. In addition, paternal lymphocyte immunotherapy is an effective treatment for URSA in that its effectiveness is due to the production of BAs in treated URSA patients. Hence, BA is very important for the detection of URSA in women. Moreover, presently genetic polymorphisms are closely related to the pathogenesis of URSA, but their specific correlations are inextricably linked with regional and population racial differences. Meta-analysis can improve the accuracy of these studies but often cannot obtain complete information. Therefore, it is necessary for future research on gene polymorphism to collect a large number of samples and integrate samples from multiple ethnic groups. In general, this article will enhance people’s understanding of patients with URSA through discussion and help develop new preventative measures or treatments.
Abbreviations
ACE: |
angiotensin-converting enzyme |
BA: |
blocking antibody |
bp: |
base pair |
CD: |
cluster of differentiation |
DCs: |
dendritic cells |
dNK: |
decidual natural killer |
eNOS: |
endothelial nitric oxide synthase |
ESR1: |
estrogen receptor 1 |
Foxp3: |
forkhead box protein P3 |
GATA-3: |
gata binding protein 3 |
HLA: |
human leukocyte antigen |
I/D: |
insertion/deletion |
IFN-γ: |
interferon γ |
IL-8: |
interleukin-8 |
imDCs: |
immature dendritic cells |
IP-10: |
induced protein-10 |
mDCs: |
mature dendritic cells |
MHC: |
major histocompatibility complex |
MLR-Bf: |
mixed lymphocyte response blocking factor |
MTHFR: |
methylenetetrahydrofolate reductase |
MTRR: |
methionine synthase reductase |
NK: |
natural killer |
PAI-1: |
plasminogen activator inhibitor 1 |
PD-1: |
programmed cell death protein 1 |
PD-L1: |
programmed cell death-ligand 1 |
PPARγ: |
peroxisome proliferator-activated receptor γ |
RORγt: |
retinoic acid-related orphan receptor γt |
RPL: |
recurrent pregnancy loss |
RSA: |
recurrent spontaneous abortion |
TAFI: |
thrombin-activatable fibrinolysis inhibitor |
TGF: |
transforming growth factor |
Th: |
T helper |
Tim-3: |
T-cell immunoglobulin and mucin domain-3 |
TNF: |
tumor necrosis factor |
Tregs: |
regulatory T cells |
URSA: |
unexplained recurrent spontaneous abortion |
UTR: |
untranslated regions |
VEGF: |
vascular endothelial growth factor |
VNTR: |
variable number tandem repeat |
Declarations
Author contributions
YK and QX equally contributed to: Conceptualization, Software, Visualization, Writing—original draft, Writing—review & editing. SC, QL, and XD: Investigation, Writing—original draft. TZ and SF: Software, Writing—original draft. DH and QL: Conceptualization, Funding acquisition, Supervision, Writing—review & editing. All authors read and approved the submitted version.
Conflicts of interest
The authors declare that they have no conflicts of interest.
Ethical approval
Not applicable.
Consent to participate
Not applicable.
Consent to publication
Not applicable.
Availability of data and materials
Not applicable.
Funding
This work was supported by the National Natural Science Foundation of China [81771575] and the Guangdong Basic and Applied Basic Research Foundation [2021A1515220178]. The funders had no role in study design, data collection and analysis, decision to publish, or preparation of the manuscript.
Copyright
© The Author(s) 2023.