Abstract
Testicular sperm maturation is critical for establishing male fertility. Spermatozoa undergo remodeling of sperm proteins and changes in lipid and ribonucleic acid composition during transport in the epididymal ducts, which play an important role in sperm maturation. The anatomy, epithelial cell types, physiological functions, and epigenetic inheritance of the epididymis are explored, and recent findings in epididymal research are analyzed. Suggesting possible directions for future research on the epididymis. Using the keywords “epididymis”, “sperm”, and “sperm maturation”, a search of the epididymis was performed through databases and official websites of journals related to reproduction. The epididymis was searched in databases and on the official websites of journals related to reproduction. This review introduces the characteristics of the epididymis, as well as the biological functions of cell types such as principal cells, clear cells, and basal cells, providing a detailed description of the overall physiological functions of the epididymis. It highlights current research hotspots in the field of epididymis, including single-cell analysis, epigenetics, and extracellular vesicles, aiming to offer a comprehensive understanding for beginners. The review emphasizes the importance of the epididymis, its impact on sperm maturation and subsequent embryo development, and how it advances research on epididymal diseases while providing new directions for the study and treatment strategies of infertility.
Keywords
Epididymis, sperm maturation, male fertilityIntroduction
It is well known that epididymis is an essential reproductive organ responsible for sperm concentration, maturation, and storage. After being produced by the testis, sperm acquire fertilization and motility in the epididymis [1–3]. Epididymal sperm maturation is critical for establishing male fertility. However, this process is often overlooked, and 40% to 50% of clinically diagnosed cases of male infertility are still labeled as “idiopathic” [4]. At the same time, other functions of the epididymis should not be neglected, such as sperm transportation, increasing sperm concentration, immunoprotection of male gametes, and its role as a sperm bank [1].
The epididymal ducts are long and convoluted, connecting the testicular efferent ducts upstream and the vas deferens downstream. The fluid environment in the epididymal ducts undergoes a series of changes as the sperm are transported along the ducts. The epididymal microenvironment is complex and continuously variated in different compartments, and therefore plays a different role in the different regions (ionic strength, pH, luminal secretory proteins, etc.), as well as for sperm modification [3]. The diverse cell types comprising the epididymal epithelium are crucial in supporting its functions. For example, principal cells (PCs) and clear cells (CCs) play important roles in the acidic luminal environment of the epididymal duct, maintaining sperm in a quiescent state during their transit through the epididymis [5]. Recent single-cell studies have identified region-specific specialization of PCs and functionally specialized subpopulations of stromal cells, which extends existing knowledge of epididymal biology and provides new directions to explore the differentiated luminal environments in different segments of the epididymis [6]. In addition, Leir et al. [7] analyzed the human proximal epididymis and postulated the potential role of basal cells (BCs) as a stem cell compartment in the epididymis, BCs may be the source of stem cells for regeneration of the epididymis epithelium. Through spatiotemporal landscape studies of specific segments of the epididymal cells, Shi et al. [8] discovered that the expression profiles of epididymal cells and sperm vary at two different developmental time points: the first-wave sperm and adult sperm. This reveals the differences in the time points of epididymal and sperm development [8].
Epigenetics has emerged as another topic in epididymal research and has made significant progress in recent years. Baskaran et al. [9] have found that the small non-coding RNA (sncRNA) profile undergoes changes during sperm transit through the epididymis, with the epididymis being considered a key factor in driving these changes [9]. Various sncRNA categories, including microRNA (miRNA), piwi-interacting RNA (piRNA), and tRNA-derived small RNA (tsRNA), have been identified in the epithelial cells (EECs) of mammalian epididymis [10–12]. In addition to their diversity, sncRNAs in the mammalian epididymis also exhibit a certain degree of regional expression patterns [13]. Under certain paternal environmental exposures, the sncRNA of sperm can be reshaped, potentially affecting the phenotypes of subsequent generations [14]. Besides sperm epigenetics, epididymis has specific characteristics to produce epididymosomes which can establish sperm competency to support normal embryogenesis [15, 16]. Understanding the crucial role of the epididymis in epigenetics not only contributes to a better understanding of how parental environmental exposures impact offspring phenotypes but also helps mitigate potential damage to the health of future generations.
However, the well-differentiated structure and specific function of the epididymis are still unclear. In order to have a more detailed and overall in-depth understanding of the structure and function of the epididymis, this paper reviews many functions of the epididymis such as sperm concentration, transportation, protection, and storage, and focuses on the epididymal epithelium in detail in terms of the anatomical structure of the epididymis, the anatomical structure-function, the epididymal cell type and from a single-cell perspective. In addition to this, the exosomes of the epididymis and epigenetics of the epididymis will also be outlined.
Methodology
In this paper, the epididymis was searched by utilizing databases and official websites of journals related to reproduction. Search on Pubmed with the keywords “epididymis”, “sperm maturation”, “principal cells”, “clear/narrow cells”, and “structure/functions of epididymis”, citing literature of importance in epididymis research in “Human Reproduction”, “Fertility and Sterility”, “Bor” and other journals’ official websites for the contents of the epididymis. Considering the current characteristics of epididymal research, the current query is summarized mainly for mouse and human epididymis. Articles that did not specifically address the target material were excluded.
Anatomy of the epididymis
The epididymis in human is normally divided into three parts: the caput, the corpus, and the cauda [17]. In experimental rodents, the epididymis is often divided into four segments: the initial segment (IS), the caput, the corpus of the epididymis, and the cauda of the epididymis [2]. The proximal region (the IS and the caput) plays a role primarily in sperm maturation, the corpus may not only participate in late sperm maturation but may support the sperm transport process through rhythmic contractions of the surrounding smooth muscle layer, and the cauda maintains the sperm in a quiescent state and contributes to in sperm storage [18]. Although the three-part anatomical description of the epididymis applies to all mammalian species, different species have unique morphological features of the epididymis. Compared to laboratory rodents, the human epididymis is poorly differentiated, with no distinct IS of the caput and without the bulbous appearance of the proximal output duct similar to that of other species [19]. Moreover, the human epididymis cauda also does not have distinctive distal tubule swelling [20]. Furthermore, its sperm bank function is hypofunctional relative to other animals [21].
Connective tissue septa (CTS) is present in the epididymis, and based on the presence of CTS, mouse and rat epididymis are subdivided into 10 intraregional segments and 19 intraregional segments, respectively [22, 23]. CTS separates the segments and can establish boundaries for epididymal gene expression, protein presence, or EEC response to phototropic factors [24–26]. During bacterial infection of the epididymis via the urinary tract, segment boundaries can limit bacterial ascension through ductal constriction of uninfected adjacent segments [27]. Although the CTS divides the epididymis into separate interstitial compartments, it has been hypothesized that specific segment-specific intersegmental communication can still occur via paracrine signaling [25]. In contrast, on longitudinal histologic sections of the human epididymis, a number of dysplastic and incomplete septa were observed, and these structures showed a high variability from specimen to specimen and did not show clearly defined epididymal segments as described by Turner et al. [25] in rodents. In summary, the human epididymal septa are organized in a manner that does not allow for the definition of epididymal segments [28], which is another feature of the human epididymal structure (Figure 1).
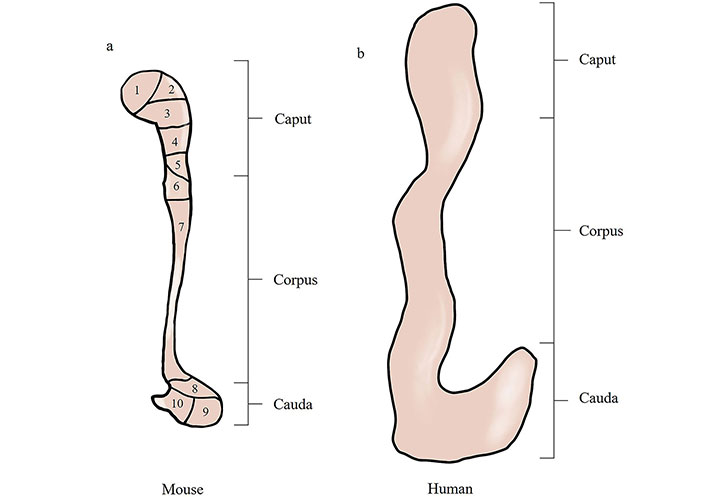
Comparison of mouse and human epididymal anatomy. (a) Schematic diagrams of mouse epididymis; (b) schematic diagrams of human epididymis. Schematic diagrams illustrate that the mouse has a well-developed epididymal caput and cauda structure with the presence of an epididymal septum. Compared to the mouse, the human epididymis has a poorly differentiated epididymal caput and cauda with no epididymal septum. 1–10: ten segments of the epididymal interstitium divided by connective tissue septa (CTS)
Epididymal cell types
The epididymal ducts are mainly composed of pseudocomplex epithelium, and the EECs consist mainly of PCs, BCs, CCs/narrow cells (NCs), lymphocytes, or halo cells (Figure 2) [29]. Rinaldi et al. [6] used single-cell analysis to delineate epididymal cells into eight identified cell populations including PCs, myxoid/fibroblasts, CCs/NCs, macrophages/monocytes, BCs, halo/T cells, endothelial cells, and spermatids.
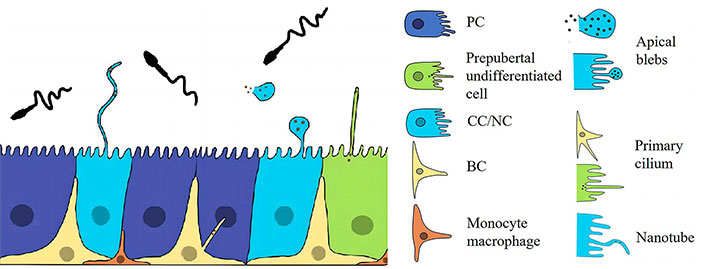
The epididymal epithelium. CCs are involved in the secretion of epithelial proteins through the release of epithelial bodies. They also contribute to the secretion of epithelial amyloid protein through the formation of luminal nanotube-like structures. These CC structures interact with spermatozoa in the epididymis and play a role in sperm maturation. The pseudostratified epithelium lining the lumen of the epididymis is composed of three main cell populations, namely PCs, CCs, and BCs. Epididymal epithelial cells (EECs) communicate in different ways to maintain homeostasis in the epididymal environment. In addition to the interaction between PCs and sperm through the secretion of vesicles and epididymosomes, CCs can also communicate with sperm through direct contact via nanotubes. BC: basal cell; CC: clear cell; NC: narrow cell; PC: principal cell
PCs
Distributed throughout the epididymal ducts, the PC type of the epididymal epithelium, the PCs comprise 65% to 80% of the epididymal epithelium, depending on the region in which they are located [15]. The PCs are secretory and absorptive cells in a highly active state, producing many specialized proteins in the lumen of the epididymis that play a role in the regulation of acidity and alkalinity, sperm maturation modification, immunomodulation, and light signaling [6]. Shi et al. [8] revealed distinct subgroups of PCs through single-cell RNA sequencing, which were defined as Prc1-Prc8. GO analysis indicated that the enriched genes in Prc7 and Prc8 are primarily associated with ciliary tissue and assembly, microtubule-based movement, and cilia-dependent cell motility, consistent with the histological description of epididymal cells. Regional gene expression is a prominent feature of epididymal PCs, as discovered by Rinaldi et al. [6] research. The most striking characteristic of region-specific gene regulation in the epididymis is the segmental expression of several large multigene families’ individual members organized into gene clusters, including beta-defensins, aldo-keto reductases, lipases, serine protease inhibitors, and ribonucleases. These gene clusters correspond to the functions of the PCs [6].
Regulation of acid-base balance
PCs and CCs play an essential role in regulating luminal pH. In different regions within the epididymis PCs rely on different mechanisms to establish an acidic environment. In the IS of the epididymis, PCs reabsorb bicarbonate through a number of transporter proteins and enzymes [30–32]. In the caudal segment of the epididymis, PCs secrete bicarbonate or protons in response to physiological signaling exchanges. The cystic fibrosis transmembrane conductance regulator (CFTR) protein is located in the apical membrane of PCs, and adenosine, adrenergic agonists, genistein, and epithelial basolateral neurotransmitters can activate CFTR and thereby mediate bicarbonate secretion [33]. CFTR has been proven to function not only in the secretion of bicarbonate by PCs but also in the secretion of ATP by these cells, ATP and its hydrolysis product adenosine can coordinate the crosstalk between PCs and CCs to activate proton secretion in CCs, thereby regulating tubular acidification [34]. Furthermore, sodium/hydrogen exchanger 3 (NHE3) in the apical membrane of PCs mediates proton secretion from PCs to regulate and maintain luminal pH [35].
In addition to directly regulating luminal pH, PCs are involved in the regulation of proton secretion by CCs through paracrine signaling. The concentration of cAMP in PCs can affect CTFR-mediated bicarbonate secretion. Elevated cAMP concentration leads to an increase in bicarbonate secretion, and studies have shown that this process is important in the initiation of spermatozoa prior to ejaculation [33]. However, chronically high bicarbonate concentrations in the epididymal ducts may be detrimental to sperm motility.
Sperm maturation modifications associated with epididymal function
PCs have the ability to synthesize and release epithelial proteins. These proteins include various metabolic enzymes, small-molecule-binding proteins, and defensins. They can bind to the surface of sperm cells, contribute to the function of the epithelium, facilitate modifications during sperm maturation, and are typically encoded by genes belonging to multigene families that are clustered together in the genome [36–38]. The proteins encoded by these gene clusters are involved in epididymis-specific functional activities. For example, antimicrobial peptides may be vital in isolating the testis from ascending infections [39, 40], sperm also undergo complex redox remodeling during epididymis, extensive cysteine oxidation leads to disulfide cross-linking of genome-packaged Fischer proteins and many other proteins that are essential for the maintenance of sperm structure [41, 42], and aldosterone reduction protects sperm during transfer to in vitro from oxidative damage. Lipoxins bind to lipophilic substances and have a wide range of functions, from signaling [43] to innate immunity [44]. Serine protease inhibitors with semen coagulant homologous proteins (synthesized mainly by seminal vesicles) and potentially protease-controlled processes play a role in semen coagulation and liquefaction [45] and can also be implicated in acrosomal spermatid maturation of spermatozoa and spermatid capacitation [46]. Finally, the high level of expression of various RNA enzymes may be important for the high level of tRNA cleavage in this tissue [16], and the resulting tRNA fragments may serve as an important factor in preventing autoimmunity [47]. Or they may act as signaling molecules for environmental regulation that are transmitted from the sperm to the fertilized egg at fertilization [16].
Immunomodulation
There is also an immunomodulatory function of the PCs. For example, apical tight junctions between neighboring PCs are an important component of the blood-epididymis barrier. Adhesion junctions are necessary for cell adhesion and can also be found in the junction complexes between neighboring PCs [48]. They also play a crucial role in preventing the production of self-targeting antibodies. Antimicrobial defense peptides secreted by PCs not only promote sperm maturation but also play a key role in preventing foreign infections [39].
In general, epididymal PCs play an essential role in sperm maturation, regulation of luminal pH, and immunomodulation. Thus, it can be seen that many important functions of the epididymis are closely related to the PCs, and the research of the PC function may be an important entry point for the study of the epididymis.
CCs and NCs
To ensure that sperm remains quiescent until mating occurs, the lumen of the epididymal ducts needs to maintain an acidic environment, which is an essential function of the CCs [5]. NCs are found only in the IS and are not distributed in other parts of the epididymis, whereas CCs are distributed throughout the caput, corpus, and cauda regions of the epididymis [3]. NCs have a distinctive “champagne-glass” morphology and CCs are more cuboidal in shape [3]. Despite differences in the distribution and morphology of hyaline and NCs in the epididymis, both are major cells that maintain luminal pH. These cells express the vacuolar proton pump and V-ATPase, which are required for luminal acidification [49]. CCs can re-acidify the lumen by activating soluble adenylate cyclase (sAC) via bicarbonate in the ependymal ducts, elevating the cAMP concentration in the cells, and increasing the proton secretion function of the proton pump (Figure 3). The luminal acidification process requires large amounts of ATP, so NCs and CCs are part of a family of “mitochondria-rich cells” that contain large numbers of mitochondria compared with other neighboring cells.
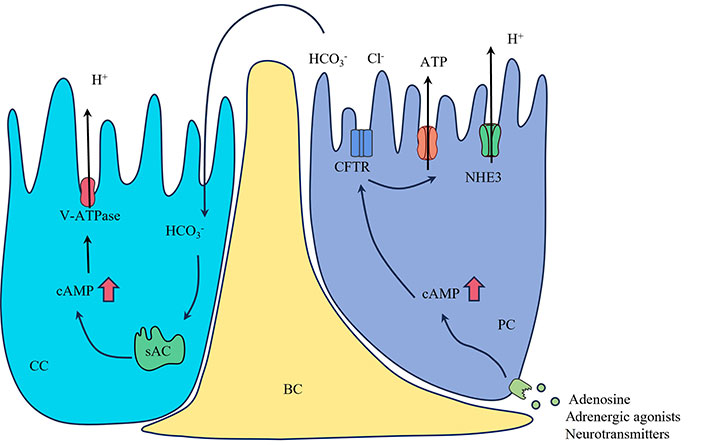
A model showing that PC regulates CC proton secretion. The PC can be stimulated by adenosine, adrenergic agonists, or neurotransmitters, resulting in elevated intracellular cAMP levels. This increase in cAMP then activates bicarbonate secretion through the apical CFTR. The bicarbonate, flowing into the lumen, reaches the CCs and triggers the activation of sAC, leading to an elevation of intracellular cAMP. CFTR also stimulates ATP secretion through the activation of membrane-bound proteins. The activation of the cAMP/PKA pathway by bicarbonate or adenosine induces the accumulation of V-ATPase on the apical membrane and promotes proton secretion. The red arrows indicate that increase in intracellular cAMP levels. BC: basal cell; CFTR: cystic fibrosis transmembrane conductance regulator; Cl-: chloride ion; H+: proton; CC: clear cell; HCO3-: bicarbonate ion; NHE3: sodium/hydrogen exchanger 3; PC: principal cell; sAC: soluble adenylate cyclase
According to the Rinaldi et al. [6] study, two subgroups of tryptophan-negative and tryptophan-positive clear were classified on the basis of tryptophan detection, and these subgroups were found to coexist even in the same region of the epididymis, with tryptophan-positive CCs found in the caput of the epididymis and downstream portions of the epididymis corpus, it will be interesting to determine whether tryptophan-amyloid secreted by the CCs are used for localized signaling within the male reproductive tract. Clustering analysis by transcriptome RNA sequencing has shown that CCs can be assigned functions related to immune activation, immune tolerance, microbial defense, antioxidant protection, inhibition of protein hydrolysis, and protein translocation to mature spermatozoa, in addition to the important function of maintaining an acidic environment [50]. CCs are involved in the secretion of epithelial proteins through the release of epithelial bodies. They also contribute to the secretion of epithelial amyloid protein through the formation of luminal nanotube-like structures. These CC structures interact with spermatozoa in the epididymis and play a role in sperm maturation; CCs can be involved in segment-specific epithelial progenitor protein processing and sperm storage and also play a role in preventing tissue oxidative defense damage; function as immunomodulators and are involved in the establishment and maintenance of a protective immune-privileged environment that enhances conditions for sperm maturation and storage [50, 51].
BCs
BCs are a characteristic feature of the mammalian epididymis epithelium, forming distinct monolayers at its base. These BCs have cytoplasmic protrusions that extend into specific regions of the epididymal lumen [52]. Mammalian BCs are scavenging and are recognized as luminal sensors that regulate the activity of PCs and CCs [53]. BCs regulate the function of CCs via their axial peduncles and cytoplasmic protrusions that cross the blood-epididymis barrier in order to sample the intraluminal environment and increase proton secretion from neighboring CCs [52]. BCs are considered adult stem cells with the potential to differentiate and regenerate the epididymal epithelium in vitro. They are believed to have a crucial role in maintaining the structural integrity of the blood-epididymis barrier [54].
Single-cell analysis revealed three subgroups of BCs, with enriched genes primarily involved in cell adhesion, membrane transport, and lipid metabolism. Further investigation into the diversity of BC subgroups identified two subgroups based on the expression levels of ribosomal protein genes. Additionally, another additional subgroup was determined, characterized by high expression levels of Notch2, Spry2, Pecam1, Slc39a1, Ccl7, Cd93, and Penk in BCs [6]. This subgroup of BCs represents an interesting direction for future follow-up studies.
Lymphocytes
Lymphocytes in the epithelium of the rat epididymis are commonly referred to as halo cells, which under light microscopy are so named because of the round, light-stained cytoplasm that surrounds the nucleus of the cell [55]. Lymphocytes in the epididymis are mainly composed of helper T lymphocytes [cluster of differentiation 4 (CD4)+], cytotoxic T lymphocytes (CD8+), and B lymphocytes. CD4+ T cells are found primarily in the human epididymal mesenchyme, whereas CD8+ T cells are the predominant lymphocytes distributed within the epididymal epithelium [56]. Using a single-cell isolation technique, Voisin et al. [57] observed that the caput and cauda of mouse epididymis had approximately equal numbers of CD4+ T cell numbers were approximately equal (1.1% and 1%, respectively); CD8+ T lymphocyte numbers were similar in the caput and cauda (1.4% and 1.05%, respectively); the number of B lymphocytes was significantly higher in the cauda of the epididymis than in the caput (0.7% and 0.35%, respectively). Adaptive immunity-dependent lymphocytes in the epididymis play a crucial role in inflammation and autoimmune response processes [58]. In view of the very low incidence of epididymal tumors in humans [59], it was speculated that other mechanisms might be involved in the antitumor effects of the epididymis, and according to Voisin et al. [57] study, it is possible that double-negative (DN) T cells replace natural killer cells in the epididymis to prevent tumorigenesis.
Macrophages
Macrophages, the most abundant immunoreactive cells in the mouse epididymis [60], are self-maintained at homeostasis independently of the bone marrow hematopoietic precursors, and colony stimulating factor-1 (CSF-1) is essential for the survival of testicular and epididymal macrophages [61]. Macrophages are predominantly distributed in the mesenchymal and peritubular regions of the epididymis, the highest concentration of macrophages can be found in the IS and the caput region, where the dendritic extensions into the lumen are more extensive. Macrophages in the epididymal corpus serve a dual role in developing immunological tolerance towards descending sperm and defending against retrograde pathogens. In the cauda region, there is a substantial capacity for phagocytosis, antigen processing, and antigen presentation, making it the most powerful in these functions [62]. This change is related to the caput responsible for capturing sperm antigens, while the epididymal tail is responsible for preserving sperm and resisting ascending infections. Macrophages in the mesenchymal region of the epididymis can express major histocompatibility complex class II antigens, which are required for antigen presentation to T cells [63]. In contrast, most macrophages in the periductal region of the epididymis lack expression of major histocompatibility complex class II [56]. Both macrophages and dendritic cells are considered “specialized” antigen-presenting cells and play complex dual roles in the epididymis. Mononuclear phagocyte system (MPS) in the epithelium of the epididymal caput may be involved in the sustained capture of sperm autoantigens to maintain tolerance, as well as in the defense response during infection [64, 65]. Communication between mononuclear phagocytes and other cells in the epididymis is crucial for maintaining the immunological homeostasis of the epididymis. For example, communication between mononuclear phagocytes and CCs helps protect the epididymis from pathogenic invasion and ensures the protection of sperm from autoimmune reactions [51]. Future research may focus on the immune status of different segments of the epididymis and more detailed immune regulation.
Blood-epididymis barrier
EECs in the epididymis form a blood-epididymis barrier in which tight junctions and specific cellular transporter proteins ensure the maintenance of a unique luminal immune-privileged environment, which is important for sperm maturation, protection, and storage [66]. The blood-epididymis barrier is an important component in maintaining the epithelial microenvironment and is formed primarily by the basolateral and apical membranes of the PCs. Tight intercellular junctions ensure epithelial function by separating their apical and basolateral domains and regulating the transport of ions and other molecules [67]. It has been suggested that in addition to tight junctions, proteins that are involved in the maintenance of adherens junctions and gap junctions also affect epididymal function [48]. The blood-epididymis barrier creates a unique intraluminal microenvironment that ensures and maintains epididymal homeostasis.
Alterations in the epididymal blood barrier may trigger inflammatory conditions, such as sperm granuloma formation, that disrupt the integrity of the epididymal blood barrier and lead to decreased fertility. Epididymal sperm granulomas are composed of degenerated spermatozoa, macrophages, neutrophils, and cellular debris [68]. Inflammation has been shown to cause granulomas and can inhibit the expression of tight junction proteins in many tissues. In cases where the integrity of the epididymis is compromised, sperm are vulnerable to attack by the immune system [69]. This indirectly demonstrates the role of the blood-epididymal barrier in protecting sperm from the immune system [68].
Epididymis function
Epididymis and sperm maturation
Epididymal sperm maturation involves the acquisition of motility and the ability of fertilization. Studies in animal models have shown that these physiological changes primarily involve sperm surface modifications that occur during epididymal transmission. (i) Increase in total negative surface charge: it has been studied that electrophoresis of immobilized live bovine and rabbit spermatozoa by Nevo et al. [70] showed that the spermatozoa moved toward the anode with their tails stretched forward. For example, in rabbits, the net surface negative charge of sperm increases during epididymal sperm maturation [42]. (ii) Modification of lectin-binding properties: the reduction of lectin-binding sites in the sperm acrosome during epididymal transport of spermatozoa may be the result of selective activation of certain glycosidases during epihydrotransport, which cuts off the lectin-binding portion of the glycosidically spliced side chain. This may make acrosomal enzymes active and opens up the possibility of selective enzyme activation and carbohydrate modification of acrosomal glycoproteins during sperm maturation [71]. (iii) Changes in membrane composition: the sperm membrane is initially derived from spermatogonia/spermatogonia in the testis, but then undergoes considerable changes during spermatogenesis, epididymal maturation, and capacitation. It is characterized by an unusually high proportion of polyunsaturated phospholipids, which endows it with special physical properties and compartmentalizes many of its component proteins and lipids into discrete domains in the head and tail [72]. In addition to that, it also includes modifications of surface glycoproteins and surface antigen relocalization [73, 74]. These surface modifications occurring during the passage of sperm through the epididymis are crucial for their fertilization ability and motility, making it a key process in sperm maturation. There is ample evidence supporting the role of the epididymis in sperm maturation [19].
Epithelial immunoprotection
The equilibrium between immune tolerance and immune activation plays a pivotal role in safeguarding male fertility by preventing the infiltration of pathogens into the reproductive system and the generation of sperm autoantigens [57, 63]. The epididymal mucosa is a particularly dynamic environment that constantly interacts with many spermatozoa to prevent an autoimmune response and to initiate an immune defense response against pathogenic microorganisms or stressors when necessary [51]. A proper balance between sperm tolerance and immune activation is essential to protect epididymal function [75]. Myeloid cells located in the epithelium and mesenchyme of the epididymis limit their development after infection or injury to the epididymis as well as to other organs and maintain homeostasis of the epididymis to avoid excessive immune responses [50, 76]. In the prevention of epithelial infections, regional constriction of the CTS of the epididymis plays an essential role in confining the infection to a certain area. However, different regions of the epididymis exhibit varying immune responses when attacked by live bacteria or inflammatory stimuli, with the cauda eliciting a stronger immune response than the caput and damage caused by inflammation [65].
Sperm storage
Prior to ejaculation, functionally mature sperms are mainly stored in the epididymal cauda [1]. The epididymal cauda contains 50% to 80% of the sperm in the entire epididymal cavity [2]. The human sperm bank capacity is relatively limited compared to other species. In order for sperm to remain quiescent during storage, it is critical to maintain the intraluminal environment, and EECs in the tail of the epididymis secrete factors to maintain this homeostasis. Although a number of factors associated with this quiescent state remain unknown, the regulation of luminal pH and the presence of specific proteins and enzymes are believed to play a major role [2].
Epigenetic inheritance of epididymal spermatozoa
There is growing evidence that sperm contribute more to the developing embryo than just providing genomic DNA, the DNA provided by sperm also contains different epigenetic marks [77]. Epigenetic modifications are defined as heritable changes in gene expression that are triggered without altering the underlying DNA sequence, and according to current research summarized epigenetic inheritance occurs through three main mechanisms: RNA-associated silencing of sncRNAs; DNA methylation; and histone modifications. During the passage of sperm through the epididymis, it is particularly vulnerable to epigenetic changes, which effect the development of the embryo [78]. During sperm maturation, changes in DNA methylation occur. Ben Maamar et al. [79] found that the sperm DNA methylation profile of male offspring from female mice exposed to toxic environments showed alterations in the epididymis. Factors such as paternal age, diet, and exposure to toxic environments during sperm passage through the epididymis can lead to sperm oxidative stress, affecting DNA methylation. This may be closely related to miscarriages and embryonic genetic integrity [80]. Histone modifications can also impact the epigenetic inheritance of sperm due to changes in the father’s exposed environment. The study by Hoek et al. [81] suggests that the father’s folate status can alter histone variant expression, thereby influencing the epigenetic inheritance of sperm. During the transport of sperm through the epididymis, changes in miRNA, piRNA, and tsRNA also occur, and this process is equally important for embryo development [82]. These sncRNAs synthesized through the epididymis can be transferred to sperm via epididymal vesicles and serve as crucial epigenetic information transmitted to the fertilized egg, participating in the regulation of certain early embryonic events and influencing the development of offspring [12]. Metabolic disorders and inflammation in the father can affect the content of sncRNAs in sperm, thereby impacting the expression of metabolic genes during early embryo development [83, 84]. Through epigenetic modifications, sperm can transmit the effects of parental environmental exposures to the offspring, and under the influence of male behavior, alcohol and drug consumption, and life conditions including high-fat diets, stress, and malnutrition, epigenetic markers of sperm are altered, which can affect embryonic development and healthy outcomes in the offspring [85–88]. Meanwhile, Sharma et al. [16] study demonstrated that diet can affect posterior phenotype by modulating tRNA levels in mature spermatozoa.
Secreted exosomes and embryonic processes
Epididymal exosomes are exosomes within the lumen of the epididymal ducts that originate from the epididymal epithelium and are released by the epididymal epithelium [89]. Exosomes are found in abundance in biological fluids, including semen, and their internal components contain lipids, proteins, miRNA, and messenger RNA, which play an important role in intracellular communication. One study examined the proteomics of epididymal microsomes isolated from bull epididymis and found that the proteins within the epididymal corpus varied from caput to cauda, possibly reflecting the delineation of specific functions [90]. Sperm maturation undergoes interactions with proteins in the luminal fluid of the epididymis, resulting in morphological and biochemical changes in male gametes [91]. Different segments of the epididymis exhibit distinct profiles of sncRNAs, as evidenced by variations in the sncRNA spectra of sperm isolated from the proximal and distal regions of the epididymis [92]. Embryos generated through intracytoplasmic injection of sperm obtained from the proximal epididymis display immature sncRNA characteristics, which may hinder their normal development compared to embryos formed using sperm from the distal epididymis [93]. Considering the significant role of sperm-carried sncRNAs in embryonic development control, the epididymis acts as a critical regulatory factor for sperm functional transformation [82].
Epithelial vesicle-associated proteins are translocated to the subcellular or membrane structural domains of spermatozoa, and the functions of these proteins are related to and involved in the acquisition of insemination competence, motility regulation, and protection against oxidative stress [94–97]. In conclusion, exosome-associated proteins are integral for sperm maturation and are excellent biomarkers for early diagnosis of male infertility. In-depth histologic studies of semen exosomes can help identify specific proteins associated with defects in exosomal function, which can contribute to the development of relevant drugs to treat exosomal dysfunction in infertile men [9].
In summary, the epididymis is an important organ in the male reproductive system, with key functions in sperm maturation, immune protection, sperm storage, and regulation of embryo development. In-depth research into the functions of the epididymis contributes to the diagnosis and treatment of infertility. Exploring the mechanisms and potential applications of the epididymis in the future holds great promise for significant breakthroughs in the field of male reproductive health.
Conclusions
The epididymis, located downstream of the testis, is a basic reproductive organ with well-characterized functions and plays a crucial role in sperm maturation and storage. Current research has revealed complex features of epididymal cell function, appendages, and epididymal-derived factors that are involved in sperm-epididymal interactions, which in turn influence sperm maturation, early embryonic development, and epigenetic transmission of paternal traits to offspring [1, 19, 77]. Recent studies have revealed the complexity of epididymal cell types, the existence of specificity and coordination among various cells, and the regulatory mechanisms and interactions among different cells that maintain epididymal homeostasis and normal function, such as the importance of CCs versus mononuclear phagocytes for epididymal immunoregulation as revealed in the Battistone et al. [98] study. On the epigenetic side, the contribution of small RNAs in the paternal environment to the metabolic phenotype of the offspring was revealed [82]. Despite significant progress in sperm maturation in the last few years of research, however, information on the exact mechanisms of epididymis in sperm maturation is still limited, thus further emphasizing the importance of advancing basic research in epididymal disease.
This review aims to provide a detailed exploration of current research on the epididymis in conjunction with single-cell studies, seeking to gain a better understanding of the epididymis’s functions and characteristics from a single-cell perspective. The epididymal lumen serves as the site for sperm maturation, and elucidating the factors influencing sperm maturation can offer solutions to issues such as poor sperm motility and functional competence, as well as the fertilization process, thereby providing avenues for treating infertility. To further advance researchers’ understanding of the precise mechanisms behind sperm maturation in the epididymis, focusing on single-cell research will be a crucial direction for the future.
Abbreviations
BCs: | basal cells |
CCs: | clear cells |
CD4: | cluster of differentiation 4 |
CFTR: | cystic fibrosis transmembrane conductance regulator |
CTS: | connective tissue septa |
EECs: | epithelial cells |
IS: | initial segment |
miRNA: | microRNA |
NCs: | narrow cells |
PCs: | principal cells |
sncRNA: | small non-coding RNA |
Declarations
Author contributions
CW: Conceptualization, Investigation, Writing—original draft, Writing—review & editing. YX: Writing—original draft, Writing—review & editing, Formal analysis. JL and QX: Investigation, Methodology. YK and XD: Investigation, Writing—review & editing. DH: Validation, Supervision.
Conflicts of interest
The authors declare that they have no conflicts of interest.
Ethical approval
Not applicable.
Consent to participate
Not applicable.
Consent to publication
Not applicable.
Availability of data and materials
Not applicable.
Funding
Not applicable.
Copyright
© The Author(s) 2024.