Abstract
Allogeneic stem cell transplantation is currently the only curative approach for a variety of malignant and non-malignant diseases. In the early transplant era, the intent of this treatment was to apply an intensive myeloablative regimen to eliminate residual malignant cells followed by the hematopoietic rescue of the patients with donor hematopoietic stem cells. However, the focus has shifted over time and allogeneic transplantation is nowadays seen as a cellular therapy in which the donor-derived immune system mounts an anti-infectious and especially an anti-tumor effect in the posttransplant phase. In order to further augment the anti-tumor effect, various approaches have been developed, including the manipulation of the donor-derived immune system in vivo or the adoptive transfer of ex vivo-expanded donor-derived effector cells. Based on their lack of alloreactivity, γδ+ T cells are shifting into the spotlight of research in the context of allogeneic transplantation. Their exploitation with regard to their anti-infectious and anti-tumor properties and their in vivo and ex vivo manipulation will lead to new therapeutic approaches to improve the outcome of patients after allogeneic stem cell transplantation. In this review, the important role of γδ+ T cells in allogeneic matched and mismatched transplantation is summarized and an outlook is discussed on how to best make use of this unique cell population.
Keywords
Allogeneic stem cell transplantation, γδ T cells, immunotherapy, adoptive transfer, chimeric antigen receptor T cellsIntroduction
Despite the rise of new cellular therapies such as chimeric antigen receptor (CAR) T cells, allogeneic (allo) stem cell transplantation (SCT; allo-SCT) remains the only curative treatment for many patients with various hematological malignancies. In the early beginnings, allo-SCT was accompanied by a high risk of often lethal complications, including organ toxicity induced by the myeloablative conditioning, graft-versus-host disease (GVHD), infections due to the delayed immune reconstitution and relapses of the underlying diseases. However, tremendous progress has been made over time to reduce the side effects of allo-SCT. Advances in the prophylaxis and treatment of GVHD as well as a better understanding of the important role of the immune reconstitution have led to a significant decrease of the complications seen after transplantation and the numbers of allogeneic transplantation are steadily increasing [1].
Allo-SCT therapy was initially only offered to patients who had a human leukocyte antigen (HLA)-matched related or unrelated donor. Since not all patients had an HLA-suitable donor, attempts were made to offer these patients a transplant from a related haploidentical donor. Unfortunately, these early haploidentical transplantations were accompanied by severe and often lethal GVHD [2].
However, approaches to avoid GVHD by either ex vivo T cell depletion [3, 4] or the use of post-transplant cyclophosphamide (PTCy) [5] have revolutionized the field and the number of haploidentical transplantation is rising [6]. Therefore, almost every patient in need of a transplant can nowadays be offered this often only curative approach.
As already mentioned, a better understanding of the immune reconstitution is important for a better outcome with respect to decreasing the risk of severe and lethal viral and bacterial infections post-transplant and maybe also to decreasing the risk of relapse of the underlying malignant disease [7]. In this regard, allo-SCT is nowadays regarded as a cellular therapy rather than just a myeloablative chemo/radiotherapy to ultimately destroy the malignant cells. The immune recovery of various immune effector cells, such as natural killer (NK) cells and especially T cell receptor (TCR) αβ+ T-lymphocytes play an important role in fighting infections posttransplant and also in decreasing the risk of relapses. γδ T cells are also an important effector cell population in allogeneic transplantation [8] and have moved into the focus of research [9]. In contrast to TCRαβ+ T cells, γδ T cells are not alloreactive, will not cause GVHD, and can have many positive effects in allogeneic transplantation [10]. Therefore, the manipulation of γδ T cells might become a powerful tool to improve the outcome of patients after allogeneic transplantation. Their role in immune reconstitution, their adoptive transfer and/or their in vivo stimulation will be discussed in this review.
The role of γδ T cells in immune reconstitution after allogeneic transplantation
Although increased numbers of γδ T cells were found in early studies of patients who developed acute GVHD after allo-SCT [11], later studies did not support these findings and no significant correlation between γδ T cells and the incidence of GVHD in the first 12 months posttransplant was reported in a later study [12]. In patients who received an ex vivo TCRαβ T cell-depleted bone marrow from partially HLA-mismatched donors, a significantly better disease-free survival (DSF) at 30 months posttransplant was observed in patients in whom the percentage of γδ T cells was more than 10% of the total lymphocyte count. There was no difference in the incidence of acute or chronic GVHD [13]. In an extended 42-month follow-up study, the authors confirmed their data [14]. A further follow-up study 8 years later with an increased number of patients (n = 153) revealed a higher 5-year leukemia-free and overall survival in patients who reconstituted with a higher number of γδ T cells [15].
Minculescu et al. [16] reported on an improved overall survival, relapse-free survival, and less GVHD in patients with fast immune reconstitution of γδ T cells 2 months after allo-SCT [16]. These results suggest a protective role of γδ T cells against relapse and interestingly also GVHD. It is of interest in this context that granulocyte-colony stimulating factor (G-CSF) participates in acute GVHD regulation by inducing T-regulatory γδ T cells including Vδ1 regulatory T cells (Tregs), CD27+Vδ1 Tregs and CD25+Vδ1 Tregs [17]. In a murine graft-versus-leukemia (GVL) and acute GVHD model, Song et al. [18] demonstrated that γδ T cells were critical in mediating GVL effects and that γδ T cells also mitigated acute GVHD by suppressing CD4+T cell activation [18].
In a recent study, Klyuchnikov et al. [19] showed that the enhanced immune reconstitution of γδ T cells after allo-SCT overcomes the negative impact of pre-transplant minimal residual disease (MRD) positive status in adult patients suffering from acute myeloid leukemia (AML) [19]. The authors investigated the potential GVL effect of γδ T cells in 100 patients with positive MRD pre-transplant. Younger age (≤ 58 years) of recipients and donors (< 30 years), sex mismatch, matched donors, cytomegalovirus (CMV) reactivation and anti-thymocyte globulin (ATG) were associated with a faster reconstitution of γδ T cell. A multivariate analysis of the MRD+ patients who had a higher than median γδ T cell count on days +30 and +100 posttransplant revealed that these patients had a significantly improved leukemia-free and overall survival. Moreover, higher levels of γδ T cells at day +30 were associated with a significantly reduced risk of relapse. Interestingly, patients who received PTCy for GVHD prophylaxis had a lower posttransplant level of γδ T cells compared to patients who received ATG. No impact of the γδ T cell level was seen in MRD-negative patients and no correlation with the development I of GVHD was observed.
Similar to NK cells, γδ T cells express inhibitory HLA receptors [20]. Dolstra et al. [21] showed that Vγ9Vδ1 T cells derived from peripheral blood of an AML patient after allo-SCT lysed freshly isolated AML blasts and AML cell lines and that the cytotoxic function of the γδ T cells was negatively regulated by the inhibitory immunoglobuline-like receptor (KIR) CD158b [21]. It is currently unknown whether alloreactive or non-alloreactive γδ T cells based on their KIR expression have an important role in the setting of allogeneic and especially in haploidentical transplantation, as it has been described for KIR-expressing NK cells [22]. In a study with pediatric patients with leukemia, the number of γδ T cells was determined after allogeneic transplantation including haploidentical transplantation with ex vivo T cell-depleted mobilized peripheral stem cells grafts [23]. Patients with a higher γδ T cell level had a significantly better event- free and overall survival within a median follow-up period of 2.7 years. Moreover, a lower incidence of bacterial and viral infections was seen in these patients, pointing towards an important anti-bacterial [24] and antiviral function [25] of γδ T cells. It is of interest in this context that especially the Vδ2neg subset expands upon reactivation of the CMV and that CMV-induced γδ T cells can recognize and lyse primary leukemic blasts [26]. One could speculate that this might be an explanation for the observation that early CMV replication posttransplant is associated with a decreased relapse risk in patients with AML [27]. It has also been shown that Epstein-Barr virus (EBV) infections posttransplant lead to an expansion of Vδ1+ T cells in a patient with acute lymphoblastic leukemia (ALL) and this subset represented more than 80% of the circulating γδ T cells [28]. In a more detailed analysis of the dynamics of the repertoires of γδ T cell antigen receptors before and after transplantation, Ravens et al. [29] showed that patients had a rapid γδ T cell reconstitution posttransplant and that they had profoundly altered TCR repertoires. Of interest, the clonal proliferation of virus-reactive γδ TCR sequences in patients with CMV reactivation hinted towards an adaptive anti-viral γδ T cell immune response [29]. In addition, Liu et al. [30] found an inverse correlation of Vδ2+ T cell recovery with EBV reactivation after allo-SCT. They also reported that immunosuppressive therapy for GVHD prophylaxis had a negative effect on the anti-EBV capacity of the Vδ2+ T cells and that strategies to reduce or omit immunosuppression posttransplant may improve the anti-EBV immunity by increasing the activity of the Vδ2+ subset [30]. Another evidence for the overall importance of γδ T cells in allo-SCT comes from a systematic review and meta-analysis by Arruda et al. [31]. These authors searched 2,412 studies, of which 11 studies with 919 patients were eligible for meta-analysis. They found that high γδ T cell levels after allo-SCT were significantly associated with a lower risk of relapse, with fewer episodes of viral infections and with a better overall and DSF. It is of importance that they did not find an association between high γδ T cell counts and acute GVHD incidence.
The role of γδ T cells in the context of ex vivo T cell depleted transplantation
Most of the so far described results have mainly been observed in patients who received an unmanipulated bone marrow or peripheral blood stem cell (PBSC) grafts mobilized with G-CSF and a subsequent posttransplant GVHD prophylaxis. In the context of ex vivo T cell-manipulated grafts and in the absence of any GVHD prophylaxis, the role of γδ T cells might become even clearer than already described. The initial ex vivo T cell depletion methods comprised the isolation of CD34+ stem cells from G-CSF mobilized PBSC [3] or the depletion of CD3+ T cells [32]. Both of these methods were associated with the co-depletion of γδ T cells and no clear picture regarding the role of γδ T cells in this setting emerged. In order to use the potential anti-leukemic and anti-infectious effects of donor-derived γδ T cells, the TCRαβ depletion of mobilized PBSCs was introduced [33]. This method is associated with a high log depletion of TCRαβ+ T cells while retaining high numbers of NK and γδ T cells in the mobilized PBSC grafts. Due to the high depletion efficacy of this method, only a short or no posttransplant pharmacological GVHD prophylaxis is necessary.
The mobilization of peripheral donor stem cells with G-SCF does not impair the function of γδ T cells. This was shown by Otto et al. [34] who demonstrated that the γδ T cells in the G-CSF mobilized grafts retain a strong tumoricidal activity and produce immunomodulatory cytokines including interferon-γ (IFN-γ) and tumor necrosis factor-α (TNF-α) [34]. They also observed a high proportion of Vγ9Vδ1 T cells in some of the G-CSF mobilized grafts. This finding was recently confirmed by Minculescu et al. [35] in a more detailed analysis of the influence of G-CSF on the mobilization of γδ T cell subsets in healthy stem cell donors [35]. They found that G-CSF preferentially mobilized naive and terminally differentiated CD45RA re-expressing effector memory T cells (termed TEMRA) cells over memory cells and that G-CSF preferentially mobilized cells of the nonVδ2 types and increased the fraction of HLA-DR positive γδ T cells. The highest increase of γδ subsets was of the Vδ1 and nonVδ1-nonVδ2 type. The Vδ1 subset of γδ T cells is of special interest since it was shown that this subset is associated with the effective killing of fresh AML blasts after allo-SCT [21].
Various studies have been performed in pediatric and adult patients using the ex vivo TCRαβ depletion with or without concomitant depletion of CD19+ B lymphocytes in a matched related or unrelated and haploidentical setting. In a prospective multicenter study of TCRαβ and CD19 depleted haploidentical SCT in pediatric and adult patients, it was shown that despite the co-infusion of a median of 10.4 × 106/kg body weight (BW, range 1.1–45 × 106) T cells, the incidence of acute GVHD was low and none of the patients developed a higher grade III or IV acute GVHD [36]. Due to the high number of adoptively transferred donor γδ T cells together with the graft, γδ T cells were, besides NK cells, the predominant subset of cells during the first 4 weeks posttransplant. Despite heavily pretreated patients, the estimated probability of overall survival at 2 years was 70% for the adult patients with a DSF of 60%. An early reconstitution of NK and γδ T cells was also observed in adult patients who received TCRαβ depleted PBSC grafts from HLA- matched related or matched (10/10 or 9/10) unrelated donors [37]. The reconstituting γδ T cell subsets included both Vδ2+ and Vδ2− cells. Lang et al. [38] observed an improved immune recovery after TCRαβ depleted haploidentical SCT in children [38]. The median number of co-transplanted γδ T cells was 11 × 106/kg BW with a wide range of 0.9–89.7 × 106/kg BW. The recovery of donor-derived γδ T cells started as early as day +7 and preceded the recovery of αβ+ T cells. A similar observation was made in pediatric patients with AML who received TCRαβ depleted PBSC grafts from matched unrelated and haploidentical donors [39]. A more detailed analysis of the reconstituting γδ T cells was reported by Airoldi et al [40]. They analyzed the γδ subsets in 27 pediatric patients after receiving TCRαβ depleted PBSC grafts. The median number of co-transplanted γδ T cells was 7.85 × 106/kg BW (range 1.5–94.5 × 106/kg BW). The majority of the reconstituting CD3+ T lymphocytes 2 to 4 weeks posttransplant were γδ+ T cells, preceding the recovery of TCRαβ+ T cells and a higher number of infused γδ T cells was associated with a later increase of αβ+ T cells. Among the reconstituting γδ T cells, mainly 3 subsets were seen, namely Vδ1+, Vδ2+, and Vδ1negVδ2neg T cells. Four weeks after transplantation, the mean percentages for Vδ1+, Vδ2+, and Vδ1negVδ2neg were 65%, 25%, and 9.6%, respectively. They then compared the γδ recovery with pediatric patients who received a CD34+ positively selected graft. Three months posttransplant, the level of γδ T cells was lower in the CD34+ cohort and most of the cells were of the Vδ1 phenotype. In patients who had a CMV reactivation, a significant increase of Vδ1+ T cells was observed [40]. Bertaina et al. [41] reported interesting data in pediatric patients on the in vivo activation of γδ T cells posttransplant after receiving a TCRαβ depleted PBSC graft. Zoledronate was given to 43 patients with ALL (n = 30) and AML (n = 13) every 28 days and most patients received two applications. Zoledronate induced an increase of the Vδ2 subset and an increase of the cytotoxicity against primary leukemic blasts. Moreover, patients who received 3 or more infusions of zoledronate had a better probability of survival compared to patients who received only 1 or 2 infusions (86% versus 54%, P = 0.008) [41]. In an extended study, Merli et al. [42] treated 46 patients with leukemia after TCRαβ depleted SCT in the absence of any immunosuppressive pharmacological GVHD prophylaxis with monthly infusions of zoledronate starting at day +20 posttransplant. A total of 139 infusions with a mean of 3 infusions per patient were given. No adverse effects were observed and patients receiving repeated infusions had lower transplant-related mortality (TRM) compared to those receiving only 1 or 2 applications of the drug (0% versus 16.7%, P = 0.01). Multivariate analysis revealed an independent positive effect on the outcome induced by repeated infusion of zoledronate [42].
Possible therapeutic exploitation of donor γδ T cells in allogeneic transplantation
Based on the available data one can safely assume that allogeneic γδ T cells are not alloreactive, neither in the HLA matched related/unrelated nor in the haploidentical setting. Therefore, γδ T cells are attractive candidates for posttransplant therapeutic strategies in allogeneic transplantation aiming to reduce the risk of infections and the risk of relapses of the underlying diseases. The in vivo activation of γδ T cells posttransplant, as already shown for zoledronate, could be one approach to exploit the anti-tumor and anti-infectious capacity of this cell population. Another approach could be the posttransplant adoptive transfer of donor-derived γδ T cells or subpopulations [43].
Besides zoledronate, various other agents including interleukin 7 (IL-7) and IL-15 have been identified [44–46] to induce the expansion of γδ T cells or subsets (for review see [47]). The ability of γδ T cells to exert an effective antibody-dependent cellular cytotoxicity (ADCC) via their expression of the CD16 Fc receptor could additionally contribute to their anti-leukemic activity [48–50] and the early in vivo use of anti-tumor antibody could be a strategy to activate γδ T cells and NK cells posttransplant, since these are the first lymphocytes to emerge after transplantation of T cell depleted grafts. Such a strategy has been described [51] and warrants further prospective clinical trials.
Only a few in vivo studies are currently performed which aim to stimulate γδ T cells in the haploidentical posttransplant setting. In one study, zoledronate is given posttransplant in patients receiving a TCRαβ depleted haploidentical graft (study identifier: NCT02508038), and in the other study, the combination of zoledronate and IL-2 is administered posttransplant in patients after haploidentical T-replete transplantation (NCT03862833).
Some studies are currently performed aiming at the ex vivo expansion of donor-derived γδ T cells with subsequent adoptive transfer in patients after allogeneic transplantation. In these early phase clinical studies, donor γδ T cells are stimulated and expanded ex vivo and infused to the patients with malignant hematological diseases after allo-SCT (NCT03885076, NCT04008381, NCT04028440, NCT03533816). It is currently not clear which subset of the γδ T cells would be superior for adoptive transfer posttransplant. The Vδ2 subset can be activated and expanded using synthetic phosphoantigen or bisphosphonates [52]. Once activated, Vδ2 cells produce pro-inflammatory cytokines such as IFN-γ and TNF-α. Most of the peripheral γδ T cells are of the Vγ9Vδ2 subset and these cells can exert a potent and broad anti-tumor cytotoxicity in an HLA-independent manner. In addition, they are resilient to the suppressive action of programmed cell death-1 (PD-1) and can co-activate NK cells [53]. Vδ1+ γδ T cells are the second most frequent subset in the peripheral blood. These cells mainly reside in mucosal epithelial tissue and also display potent anti-tumor activity [54]. Various approaches have been described to ex vivo expand Vδ1 cells [55]. Based on the lack of alloreactivity, γδ T cells would constitute an ideal tool for donor-derived CAR T cell therapy posttransplant. Rozenbaum et al. [56] described a 14-day procedure to produce anti-CD19 γδ CAR T cells from peripheral blood mononuclear cells from healthy donors with a median expansion of 185-fold. The transduction efficiency was 60.5% ± 13.2%. The cells were effective against CD19 positive targets in vitro and in an in vivo mouse model. Interestingly, multiple injections and priming of the γδ CAR T cells with zoledronate increased their in vivo anti-tumor function. Based on the natural cytotoxicity of γδ T cells, the anti-CD19 γδ CAR T cells were also able to lyse CD19 negative targets, which was further enhanced by priming the CAR cells with zoledronate [56].
Another approach to manufacture large numbers of γδ CAR T cells of the Vδ1 phenotype is described by Nishimoto et al. [57]. Nishimoto describes the use of an agonistic monoclonal antibody (mAb) that selectively activates and expands Vδ1 γδ T cells in healthy donor-derived peripheral mononuclear cells to achieve clinically relevant yields. Once activated, Vδ1 γδ T cells are transduced with a self-inactivating, replication-incompetent gamma-retroviral vector to express a CD20 CAR. After transduction, γδ T cells are further expanded in culture, enriched by TCRαβ depletion, and then formulated for cryopreservation. This process has also been used to support the production of multiple CAR γδ T cell products expressing glypican- 3(GPC-3)-targeted CARs [58, 59].
A first-in-class clinical study using off-the-shelf third-party anti-CD20 Vδ1 CAR T cells is currently underway in patients with lymphoma (NCT04735471). Since the adoptive transfer of allogeneic donor-derived TCRαβ+ T cells might be associated with an increased risk of GVHD, donor-derived Vδ1 γδ CAR T cells manufactured with this method could be a powerful effector cell population, and clinical studies using posttransplant donor γδ CAR T cells are warranted.
Conclusions
It is commonly accepted that allo-SCT can be regarded as an effective form of cellular therapy. Many effector cell populations including NK cells, TCRαβ+ T cells, and γδ+ T cells may play together to exert a strong anti-tumor effect posttransplant. It is therefore important to analyze the individual contributions of each of these cell populations in order to maximize their therapeutic efficacy. In contrast to TCRαβ T cells, γδ T cells are, similar to NK cells, not alloreactive and therefore an interesting target for further augmenting their anti-infectious and anti-tumor properties posttransplant. Especially in the absence of posttransplant pharmacological immunosuppressive prophylaxis for GVHD, they can be activated in vivo with various drugs and cytokines, even in the haploidentical setting. The lack of alloreactivity also allows the adoptive transfer of ex vivo activated and expanded γδ T cells as well as the posttransplant application of donor-derived γδ CAR T cells. However, future research must also investigate the potential suppressive activity of adoptively transferred γδ T cells. In Figure 1, various possible approaches are summarized. Hopefully, these approaches will lead to an improvement of the outcome of allogeneic transplantation by reducing the infectious complications and more importantly by reducing the risk of relapses of the underlying malignant diseases.
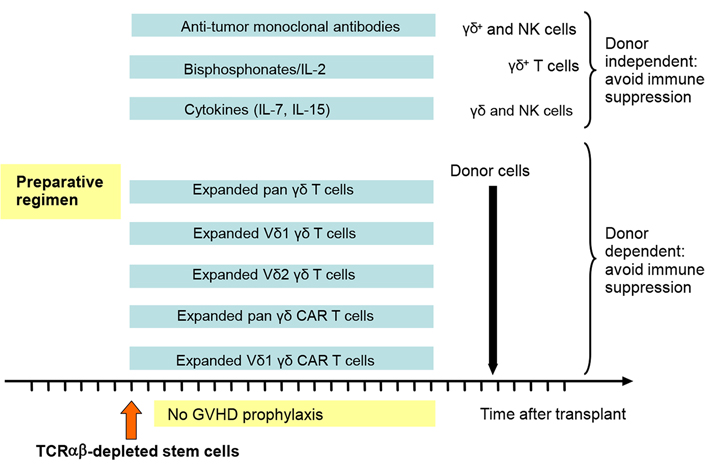
Different approaches to how to exploit γδ T cells in a platform of TCRαβ depleted SCT are suggested. Since there is no GVHD prophylaxis necessary, the in vivo and ex vivo treatment can be started early after transplant when the first donor-derived γδ and NK cells reappear
Abbreviations
allo-SCT: | allogeneic stem cell transplantation |
AML: | acute myeloid leukemia |
BW: | body weight |
CAR: | chimeric antigen receptor |
CMV: | cytomegalovirus |
DSF: | disease-free survival |
EBV: | Epstein-Barr virus |
G-CSF: | granulocyte-colony stimulating factor |
GVHD: | graft-versus-host disease |
GVL: | graft-versus-leukemia |
HLA: | human leukocyte antigen |
IL-7: | interleukin 7 |
KIR: | killer cell immunoglobulin-like receptor |
MRD: | minimal residual disease |
NK: | natural killer |
PBSC: | peripheral blood stem cell |
SCT: | stem cell transplantation |
TCR: | T cell receptor |
Treg: | T-regulatory |
Declarations
Acknowledgments
The authors acknowledge the continuous support of the Elternverein and Stiftung für krebskranke Kinder Tübingen e.V.
Author contributions
All authors contributed to the literaure search. RH wrote the first draft. All authors contributed to the manuscript and read and approved the submitted version.
Conflicts of interest
RH is a co-patent holder of the TCRαβ depletion technology together with the Miltenyibiotec company. The other authors declare that they have no conflict of interest.
Ethical approval
Not applicable.
Consent to participate
Not applicable.
Consent to publication
Not applicable.
Availability of data and materials
Not applicable.
Funding
Not applicable.
Copyright
© The Author(s) 2022.