Abstract
Arginase-1 (Arg1) and the inducible nitric oxide synthase 2 (NOS2) compete for the common substrate L-arginine, semi-essential amino acid, and central intestinal metabolite. Both enzymes exhibit various, sometimes opposing effects on immune responses, tissue regeneration, or microbial growth and replication. In sub-mucosal tissues of patients suffering from inflammatory bowel disease (IBD), similar as in experimental colitis, the expression and activity of both enzymes, Arg1 and NOS2 are more prominent than in respective controls. Accordingly, the metabolism of L-arginine is altered in IBD patients. Thus, L-arginine represents a promising medical target for clinical intervention in these devastating diseases. Previous studies primarily focused on the host side of L-arginine metabolism. Initial reports using Arg1 inhibitors generated conflicting results in murine colitis models. Subsequently, only the generation of conditional Arg1 knockout mice allowed reliable functional analyses of Arg1 and the L-arginine metabolism in the immune system. Utilizing cell-specific conditional Arg1 knockouts, we have recently reported that Arg1, surprisingly, hampered the resolution of experimental colitis due to the restriction of the intraluminal availability of L-arginine. Reduced levels of L-arginine restrained the compositional diversity of the intestinal microbiota and subsequently the mutual metabolism between the microbiota and the host. Thus, the intraluminal microbiota represents a potential therapeutic target for L-arginine metabolism aside from host-dependent L-arginine consumption.
Keywords
Arginase-1, L-arginine, inflammatory bowel disease, colitis, gut microbiota, intestinal metabolismIntroduction
Inflammatory bowel disease (IBD) is a chronic, relapsing disorder of the gastrointestinal (GI) tract. IBD has been considered to be a problem of industrial-urbanized societies. However, the incidence and prevalence of IBD in developing countries are also steadily rising, presumably due to the rapid modernization and westernization of the population. Furthermore, patients acquire IBD nowadays in their younger and younger years and exhibit an increased risk of developing colorectal cancer (CRC) [1, 2]. Thus, IBD is an emerging threat to global health systems nowadays.
Patients suffering from IBD experience a severely reduced quality of life. Collectively, IBD can result in debilitating physical and psychosocial symptoms for patients and affect society through loss of schooling, absenteeism, and healthcare costs [3].
Current therapeutic options, which primarily aim to restrain intestinal inflammation, include nonsteroidal anti-inflammatory drugs (NSAIDs) as the first-line therapeutics, followed by steroids, and finally biological therapies in a step-by-step therapy regimen [4]. These therapeutic options are accompanied by several, sometimes serious, but frequently reversible side effects (e.g., headache, drug fever, skin fever, etc.) [5]. Importantly, biologic agents targeting genetic, geographic, or microbial factors induce remission in just 40% of all patients after 12 weeks [6]. Thus, new therapeutic targets and approaches are urgently required, as the incidence of IBD is dramatically rising [7, 8].
To date, IBD is known for over 220 years [9]. The term IBD was coined by Samuel Wilks 160 years ago [10]. It includes Crohn’s disease (CD), ulcerative colitis (UC), and indeterminate colitis [11, 12]. IBD represents nowadays the most common disorder of the GI tract. It is characterized by an immune-mediated inflammation and/or ulceration of the gut [12, 13], that causes abdominal pain, (bloody) diarrhea, and/ or weight loss [14]. Both disorders usually affect predominantly the colon with mucosal lesions starting predominantly in the last section of the rectum. From there mucosal lesions can either spread continuously (UC) or in sections (CD) [15–18]. However, IBD patients do not only suffer from intestinal symptoms. Extra-intestinal complications of IBD include anemia, arthritis, or primary sclerosing cholangitis (PSC) [19].
IBD are complex diseases, which arise as a consequence of dysregulated mutual interactions of the commensal microbiota with the humoral, the intestinal epithelial, and the cellular immune network of the gut in genetically susceptible individuals [20]. However, molecules, metabolites, and signaling pathways controlling the interactions of the intestinal microflora with the immune system of the patient and their role in the suppression of intestinal inflammation have been rarely identified.
Genetic susceptibility in IBD
Since concordance rates of 30% for CD and 15.4% for UC have been reported in monozygotic twins, the genetic component of IBD got in the focus of IBD research [21, 22]. Genome-wide association studies (GWAS), frequently using chip assays for the detection of single nucleotide polymorphisms (SNPs), have identified more than 240 different susceptibility genes and potential risk loci for IBD to date [23–25]. For example, genetic polymorphisms of nucleotide-binding oligomerization domain 2 (Nod2), encoding for an intracellular pattern recognition receptor or of the interleukin (IL)-17/IL-23 cytokine axis exhibit the largest effect size in IBD [22, 25]. Since the majority of these susceptibility genes encodes for molecules involved in the recognition of bacteria or pathways regulating the immune response to bacteria, gut-resident bacteria became another focus of interest [26].
The role of L-arginine in IBD
By exploring distinct pathways in IBD patients, which have gotten into remission, the branched-chain amino acid L-arginine and arginase-1 (Arg1), one of the enzymes using L-arginine as substrate, have been identified as promising targets [27]. Arg1 exerts pleiotropic immunoregulatory effects. Importantly, in contrast to the general idea of acting as an anti-resolvin [28], our recent publication suggests, that Arg1 impedes the resolution of colitis by altering the microbiome and metabolome due to the consumption of intraluminal L-arginine [29]. Since L-arginine metabolism is a pivotal factor for the resolution of colitis, several studies linked the complex L-arginine signaling network to genetic polymorphisms. Although there were no associations between Arg1 and Nod2 or between Arg1 and IL-17/IL-23 [30], intracellular pathogens are well known to induce Arg1 in mouse macrophages upon engagement of Toll-like receptors (TLRs) and signal transmission through the signal transducers and activators of transcription (STATs) [31], important genetic loci in IBD [31, 32]. In addition, genetic polymorphisms in nitric oxide synthase 2 (NOS2) encoding for the inducible NOS2 are an increased risk factor specific for CD, and polymorphisms in the gene encoding nuclear transcription factor-kappa B (NF-kB), a pleiotropic transcription factor, are linked to inflammatory Arg1 or NOS2 pathways [32–34].
L-arginine converting enzymes in the pathogenesis of IBD
L-arginine is a central intestinal metabolite [35], which is involved in numerous biologic processes during health or disease, including immune-mediated inflammation of the GI tract. Aside from being a precursor for nitric oxide (NO) and a regulator of nitrogen balance in the whole body, L-arginine is pivotal for the modification of proteins and the synthesis of polyamines [36, 37]. L-arginine is converted either by NOS2 into NO and citrulline or by Arg1 into ornithine and urea [29, 38, 39]. Thus, both iso-enzymes, Arg1 and NOS2 compete for the common substrate L-arginine [40]. While NO is known for potent anti-microbial and immune-regulatory activities, stimulating mucosal blood flow and mucus generation [38], Arg1 serves as a precursor for the synthesis of proline and polyamines which are involved in the proliferation of cells and collagen synthesis [41, 42] (Figure 1). In addition, Arg1 has been also linked to immune cell (dys-)function, resulting in an inhibition of T cell proliferation and subsequent immune suppression, causing increased availability of polyamines and impaired NO production [43]. Today their role in the pathogenesis of IBD is discussed controversially, letting L-arginine and Arg1 appear as Janus-like characters.
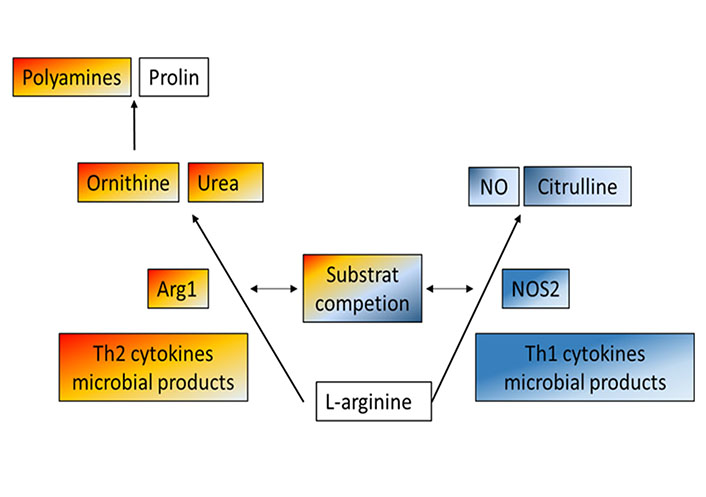
Metabolism of L-arginine-schematic and simplified overview of two main enzymatic pathways: Arg1 and NOS2 compete both for the common substrate L-arginine. While NOS2 converts L-arginine into citrulline and NO, known for potent antimicrobial and immunoregulatory activities, Arg1 metabolizes this semi-essential amino acid into proline and polyamines, which drive cell proliferation and collagen synthesis, but also in immune cell dys-function
On the one hand, Arg1 expression correlates with the degree of inflammation observed in tissue biopsies of patients suffering from IBD and in animal colitis models [29, 44–47]. Subsequently, the intraluminal availability of L-arginine is reduced in IBD patients [48]. Accordingly, improved intraluminal availability of L-arginine and genetic deletion of Arg1 in hematopoietic and endothelial cells mediated significant protection from experimental colitis [29].
On the other hand, the usage of arginase inhibitors led to conflicting results. For example, the blockade of Arg1 due to the oral application of S-(2-boronoethyl)-L-cysteine [49] or 2(S)-amino-6-boronohexanoic acid NH4 did not affect acute ongoing colitis [50]. Anti-colitogenic effects of chemical Arg1 blockade have been also reported [47]. Other studies describing the protective role of Arg1 in colitis, primarily used ex-vivo models. In these experiments, arginase activity was determined by cultivating protein [51] or macrophage supernatants [52] in Hank’s balanced salt solutions (HBSS) containing L-arginine and 14C-arginine with or without the addition of nor-NOHA. For example, an increased Arg1 expression in the macrophages was expected due to indirect measurements of urea levels, a product of Arg1 metabolism [53]. Unfortunately, however, the transfer of the in vitro observations to direct in vivo models is missing in these studies, or in vivo models were not performed, presumably due to the limited availability of arginase inhibitors [52]. However, these data have to be interpreted with caution, as experiments were exclusively performed with arginase inhibitors. These can act unspecific, and their pharmacokinetic action and tissue distribution are not well understood. Furthermore, Arg1 inhibitors just achieve around 40% inhibition of total Arg1 activity [50].
M2 macrophages protect against intestinal inflammation
During intestinal inflammation, macrophages might not control the replication, the growth, and/or the spread of microbes and thus can promote inappropriate inflammatory immune responses in mucosal tissues [54]. On the other hand, M2 macrophages expressing Arg1 as one cell-characteristic feature [55] have been associated with anti-colitogenic effects. M2 macrophages, activated by IL-4, perpetuate protection from experimental colitis through a reduction of inflammatory cytokine production in response to pro-inflammatory stimuli and an increased release of anti-inflammatory cytokines like IL-10 [56]. Protection can be mediated through the catalytic subunit activity of the class I phosphatidylinositol 3 kinase P110δ of M2 macrophages [50].
Several reports suggested that the blockade of Arg1 activity by specific inhibitors resulted in a reduction of Arg1+ macrophages and an upregulation of NO expression [50, 57]. However, it remained unclear whether the blockade of Arg1 was linked to a loss of M2 macrophage polarization. The effect of Arg1 depletion on other cell populations has not yet been explored; publications so far have only focused on macrophages or F4/80+ cell populations. Thus, it is still unknown, whether protection from colitis through M2 macrophages might be even independent of Arg1. Indeed the heterogeneous experimental outcomes suggest signals from the microenvironment rather than arginase inhibition of the host [50, 58]. Surprisingly, microbiota, well-known complex ecosystems that are structurally and functionally altered during colitis, were not investigated in these studies although most of the identified genetic risk loci in IBD were associated with microorganisms or host-microbiota interactions [26]. Thus, futures studies need to take into consideration the whole microenvironment, by determining the effects of Arg1 during colitis development and progression.
Microbiota in IBD
Gut microbiota is similar to the host a source of different metabolites including polyamines [59]. Bacteria like Firmicutes or Bacteroidetes associated with human health and/or the presence of anti-inflammatory microorganisms such as Bifidobacterium or Faecalibacterium are decreased in fecal samples of IBD patients [27, 60, 61]. At the same time Enterobacteriaceae, including Escherichia coli [62], and Helicobacter spp. are increased, which are known to contribute to the destruction of the colonic mucus layer [29, 60]. We also observed that the ratio of Firmicutes to Bacteroidetes is higher in Tie2-Cre × Arg1fl/fl mice lacking Arg1 in hematopoietic and endothelial cells compared to wild-type littermate controls (Arg1fl/fl). In addition, bacterial genera are associated with human health as well as maintaining gut homeostases, like Ruminocococcus, Turicibacter, Fodinicurvata, Christensensella, Acetanaerobacteria, and Clostridia spp. show significant expansion in the Tie2-Cre × Arg1fl/fl mice [29, 63, 64].
Reduced intraluminal availability of L-arginine impedes the resolution of colitis
In the last two decades, in vivo models including the global or conditional knockout of either Arg1 or NOS2 were created [29, 65–67]. However, most of the published literature about the role of Arg1 during colitis, focused on specific conditional knockout models in different cell subsets, because a global knockout of Arg1 is lethal [68].
In the in vivo knockout models, L-arginine exhibited a Janus-faced function, depending on which kind of iso-enzyme utilized it as substrate. Recently published data assumed that an increased Arg1 expression triggered by the intestinal microbiota and by the T helper type 2 (Th2), cytokines IL-4 and IL-13, perpetuated intestinal damage [29]. Knockouts of Arg1 in either myeloid (Cx3cr1-Cre × Arg1fl/fl) or endothelial cells (Cdh5–Cre × Arg1fl/fl) alone were not sufficient to significantly impact the progression of colitis. In contrast, mice with a knockout of Arg1 in endothelial and hematopoIetic cells (Tie2-Cre × Arg1fl/fl), resolved colitis faster compared to respective littermate controls. The additional deletion of Arg2 encoding the mitochondrial iso-enzyme and NOS2 even decreased further the severity of colitis, as reported before [29, 51]. Thus, we concluded that the enzymatic consumption of intraluminal L-arginine through NOS2, Arg1, or other L-arginine converting enzymes promotes colitis. Subsequently, the supplementation of L-arginine in dextran sodium sulfate (DSS)-treated mice improved intestinal pathology [48], while dietary L-arginine restriction resulted in progressive weight loss, IL-17 production, and fatal wasting disease [29]. The compositional changes of the microbiota and their reduced diversity promoted the production of pro-inflammatory cytokines in DSS-treated wild-type mice (Figure 2), while in Arg1 knockouts the anti-inflammatory immune response combined with the accumulation of intraluminal polyamines accelerated mucosal healing [29].
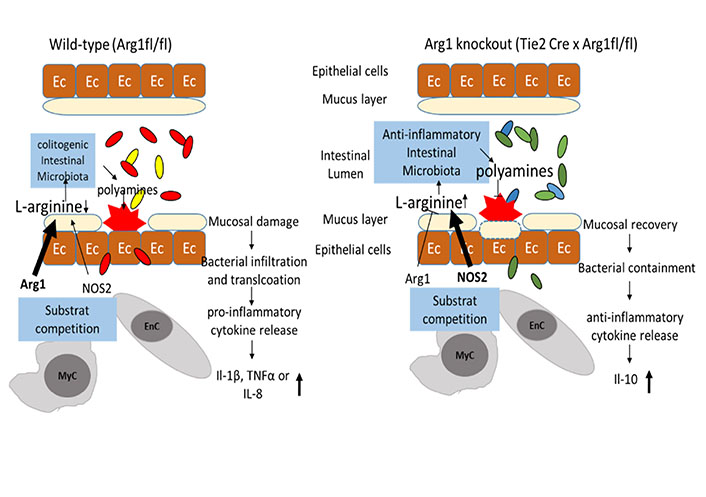
The availability of intraluminal L-arginine and subsequent changes in the composition of the intestinal microbiota: the availability of intraluminal L-arginine influences the bacterial diversity between wild-type mice (left panel) and mice with an Arg1 knockout in endothelial and hematopoietic cells (Tie2-Cre × Arg1fl/fl; right panel). Once colitis is established, the increased expression of Arg1 in intestinal tissues accelerates the consumption of L-arginine, subsequently resulting in reduced diversity of intestinal microbiota. In contrast, intraluminal L-arginine is more available in Tie2-Cre × Arg1fl/fl mice, due to the lack of consumption by Arg1 in endothelial and myeloid. Commensal bacteria known for human health are increased in Tie2-Cre × Arg1fl/fl mice, metabolize L-arginine into polyamines and induce mucosal healing
Note. Adapted from “Arginase impedes the resolution of colitis by altering the microbiome and metabolome” by Baier J, Gänsbauer M, Giessler C, Arnold H, Muske M, Schleicher U, et al. J Clin Invest. 2020;130:5703–20 (https://www.jci.org/articles/view/126923). © 2020, American Society for Clinical Investigation.
The increased availability of intraluminal L-arginine is linked to a more physiologic and diverse microbiota. Interestingly, a fecal microbiota transfer from Arg1 knockout donors into wild-type recipients induced a significant reduction of intestinal damage and enhanced the recovery from colitis. Tie2-Cre × Arg1fl/fl mice show in comparison to wild-type controls also a different cytokine profile. Pro-inflammatory cytokines and cytokine receptors like IL-1β, tumor necrosis factor (TNF), or IL-8 are reduced in Arg1 knockout mice and anti-inflammatory cytokines like IL-10 are enhanced [29].
According to the Janus-faced role of L-arginine during colitis, these studies suggest L-arginine as a promising new therapeutic approach in IBD and the metabolism of the microbiota as a pivotal target. To date clinical human trials and data from long-term studies are missing; however, they are urgently required to evaluate potential adverse effects and safety issues.
Conclusion
IBD affects patients life-long and severely affect their quality of life. Even though our knowledge about the pathogenesis of IBD has advanced in recent years, we are still just beginning to understand these devastating disorders. Therefore, we need to extend and improve current therapeutic options, which do not cure the underlying disease and force patients into remission by improving clinical symptoms at their best. Thus, novel targets and approaches for clinical intervention are urgently required.
Recent publications suggest the metabolism of L-arginine, a central intestinal metabolite, and its metabolizing enzymes as promising novel avenues for treating IBD. Although the role of Arg1 and NOS2 during IBD is still controversial and results due to experimental limitations have to be interpreted and discussed with caution, one needs to consider that not only the host metabolizes L-arginine, but also its commensal microbiota, particularly in the gut. Thus, dietary supplementation of intraluminal L-arginine might act protective, due to the expansion of a local diverse microbiota. Furthermore, this locally restricted application of L-arginine might avoid the severe adverse effects observed following systemic infusion [69–71]. Thus, we need to further focus on the metabolism of the intestinal microbiota and the complex, mutual host-microbiota network in the future to establish L-arginine supplementation as a therapeutic option for IBD in the future [29, 48, 72, 73].
Abbreviations
Arg1: | arginase-1 |
CD: | Crohn’s disease |
GI: | gastrointestinal |
IBD: | inflammatory bowel disease |
IL: | interleukin |
NO: | nitric oxide |
NOS2: | nitric oxide synthase 2 |
UC: | ulcerative colitis |
Declarations
Author contributions
BN wrote the first draft of the manuscript, selected the references, and designed the figures; JM wrote the manuscript. Both authors contributed to manuscript revision, read and approved the submitted version.
Conflicts of interest
The authors declare that they have no conflicts of interest.
Ethical approval
Not applicable.
Consent to participate
Not applicable.
Consent to publication
Not applicable.
Availability of data and materials
Not applicable.
Funding
This study was supported by the German Research Foundation DFG (grant MA 2621/4-1 to JM; grant CRC1181, project C04 to JM). The funders had no role in study design, data collection and analysis, decision to publish, or preparation of the manuscript.
Copyright
© The Author(s) 2021.