Abstract
Metastasis causes a majority of deaths in breast cancer patients. Metastasis is the spread of cancer to distant sites in the body away from the primary tumor, creating secondary tumors, or metastases. A tumor metastasizes when cancer cells strategically regulate genes that play a role in angiogenesis, epithelial-mesenchymal transition (EMT), migration, invasion, and regulation of the cell cycle to bypass apoptosis and increase proliferation and stemness. Several transcription factors have also been identified to play a role in metastatic breast cancer, as they enable invasion, intravasation, transport, extravasation, and colonization of metastasis through other processes such as angiogenesis and EMT, making them a prime target for cancer treatment. Understanding how transcription factors play a role in breast cancer metastasis will enable the development of targeted therapeutics for breast cancer. This paper reviews the roles of E2Fs, hypoxia-inducible factors (HIFs), EMT master regulators, sex determining region Y (SRY)-related high-mobility group (HMG) box (SOX), E26 transformation-specific (ETS), Yin Yang 1 (YY1), forkhead box M1 (FoxM1), BTB domain and CNC homology 1 (Bach1), sineoculis homeobox homolog (SIX), runt-related transcription factor 2 (RUNX2), myelocytomatosis (MYC), Kruppel-like factors (KLFs), and c-Jun in breast cancer metastasis.
Keywords
Breast cancer, metastasis, transcription factors, EMTIntroduction
Transcription factors, DNA-binding proteins, are vital to gene expression, as they allow RNA polymerase to bind to the DNA and begin transcribing the target gene. Transcription factors can significantly impact cancer development because of this crucial role in cell growth and functionality.
Breast cancer first develops in the breast lobules, tubes, or connective tissue [1]. It can be classified into four molecular subtypes: luminal A, luminal B, human epidermal growth factor receptor 2 (Her2)-positive, or triple-negative (TN) breast cancer [1]. Each type of breast cancer has different qualities in prognosis and treatment, and multiple subtypes can be present in the same patient or even the same tumor, which is considered tumor heterogeneity.
This review will focus on the role of transcription factors emphasized in current research to understand the specific aspects of breast cancer metastasis that are impacted by transcription factors.
E2F transcription factors
The E2F family of transcription factors is an evolutionarily conserved family of eight genes that encode ten proteins [2]. The E2F family consists of both oncogenes and tumor suppressor genes that code for transcription activators, transcription repressors, and cell cycle regulators that also seem to regulate metastasis in the early and late stages of progression [2, 3]. E2F1, 2, and 3A are activators, E2F3B, 4, and 5 are passive repressors, and E2F6, 7A, 7B, and 8 are active repressors [2]. Several studies provide evidence that the RB-E2F pathway has a role in therapy resistance [2]. ER-positive breast cancer tumors were treated with tamoxifen, an estrogen receptor antagonist that is the primary therapy for ER-positive breast cancer [2]. E2F1, E2F4, and genes involved in RB-E2F pathway-regulated processes were enhanced in tamoxifen-resistant tumors [2].
In one study by Hollern et al. [3], genomic signaling pathway signatures were applied to mouse tumor model microarray data to hypothesize that E2Fs regulate tumor development and metastasis in mice. To test the hypothesis, the effects of the loss of transcription factors E2F1, E2F2, and E2F3 were observed in different crosses of mice [3]. Loss of E2F1 and E2F2 reduced the metastatic ability of the tumors, although tumor onset was accelerated [3]. The loss of E2F1 was also found to cause upregulation of E2F3A, which is necessary for cell proliferation [3]. E2Fs also have a role in tumor heterogeneity, as each member of the E2F family can respond to different stimuli in various ways to regulate several genes and processes [3]. The Akt pathway was activated by E2Fs in the polyomavirus middle T oncoprotein (PyMT) tumor model [3]. E2F loss also causes the expression of genes responsible for tumor angiogenesis, tumor cell remodeling of the extracellular matrix, tumor cell survival, and tumor cell interactions with vascular endothelial cells to facilitate metastasis to the lungs, all of which are crucial to metastasis and cancer progression [3].
In another study by Liu et al. [4], transcription and survival data in breast cancer patients from online databases were observed. E2Fs specifically play a role in TN breast cancer, the breast cancer subtype with the lowest survival rates [4]. mRNA expression levels for the eight known E2Fs were higher in breast cancer tissues compared to normal tissue [4]. High levels of E2F1, E2F3, and E2F8, specifically, significantly correlated with lower overall and relapse-free survival [4]. Overall, the study indicated that E2F1, E2F3, E2F7, and E2F8 are potential targets for individualized treatment, and E2F4 could be a prognostic marker for survival [4]. E2Fs were also found to mediate invasion in H-Ras-dependent invasion of breast cancer through the RB-E2F pathway [2].
The cyclin-dependent kinase (CDK)-RB-E2F pathway has also garnered interest among breast cancer researchers. CDKs regulate the cell cycle by binding to cyclins, which build up throughout each stage of the cycle. E2Fs bind to RB1 proteins in this pathway, creating E2F-RB1 complexes that repress genes involved in cell cycle progression, which is a possible explanation for the loss of E2Fs contributing to cancer development [2]. Drugs targeting this pathway using CDK inhibitors are promising [2].
Song et al. [5] found that E2F1 promotes breast cancer metastasis by initiating transcription of the PRSS22 gene. This gene is upregulated in human breast cancer tissues and expressed at even higher levels in patients with lymph node metastasis [5]. Overexpression and knockdown data reveal that PRSS22 enhances migration and invasion abilities, making breast cancer cells more aggressive [5]. ANXA1 is the substrate of PRSS22, the cleaving of which activates formyl peptide receptor 2 (FPR2)/ERK signaling cascades to contribute to breast cancer progression [5].
Hypoxia-inducible factors
Hypoxia is the lack of oxygen available for tissues to adequately carry out their function. Tumor cells must adapt to hypoxia, as regions of the tumors are often too far from a blood vessel to access the same oxygen concentrations as normal tissues [6]. Hypoxia is associated with increased aggressive tumor behavior, metastasis, and resistance to therapy [7]. Breast cancer cells increase the levels of hypoxia-inducible factors (HIFs) when experiencing hypoxia, and these tumors have an increased risk of metastasis [6]. The HIF genes fall under the basic helix-loop-helix (bHLH) + PAS-containing gene family, and their proteins are characterized by the presence of an N-terminal bHLH DNA binding domain upstream of two PAS domains [8]. HIF-1 is a known regulator of processes such as angiogenesis, cell survival, and invasion [9].
Signal transducer and activator of transcription 3 (STAT3) is a transcription factor active in over 70% of breast cancers [10]. STAT3 activates HIF-1α, leading to overexpression of vascular endothelial growth factor (VEGF) and platelet-derived growth factor (PDGF), major signaling proteins that promote angiogenesis [10, 11]. HIFs allow cells to adapt to hypoxic conditions by activating alternate metabolic pathways that are not dependent on oxygen, such as the pentose phosphate pathway, often doing so by targeting genes involved in cell survival, angiogenesis, metabolic reprogramming, immortalization, epithelial-mesenchymal transition (EMT), stem cell maintenance, resistance to radiation and chemotherapy, invasion and metastasis by altering different signaling pathways [6, 7, 9]. The VEGF, PI3K/Akt/mTOR, and MAPK signaling pathways are potential inhibition targets for the treatment of breast cancers [7].
One mouse model study by Liao et al. [9] suggested that HIF-1α is not required for the formation of mammary tumors but does accelerate tumor onset and progression. However, HIFs are necessary for the rapid proliferation of mammary epithelial tumor cells in hypoxic microenvironments [9]. Primary tumors and metastases can react to hypoxia differently, making the study of microenvironments necessary for developing new treatments [9]. A treatment targeting the hypoxic microenvironment of a primary tumor in one way may not be effective for a secondary tumor, and vice versa. In addition, tumors vary from patient to patient, which can impact the treatment’s effectiveness.
The clear correlation between HIF presence and metastasis makes them potential prognostic markers for breast cancer. Drugs that block HIFs have promising therapeutic effects as they decrease primary tumor growth, invasion, vascularization, and metastasis [6]. Inhibitors of the HIF-1 pathway include those that impact HIF-1α protein levels, HIF-1 mRNA, HIF-1 dimerization, HIF-1 DNA binding, and HIF-1 transactivation [12]. The HIF-1 pathway is highly activated in TN breast cancer tumors, so they may be particularly effective in these cases, especially since current treatment options work poorly against this type of breast cancer [12].
EMT master transcription factors
EMT is a developmental process used during embryogenesis and utilized by cancers, for tumor progression in which cells lose cell junctions and apical-basal polarity while gaining cell motility and invasive ability to transition from epithelial to mesenchymal cells [11, 13]. EMT occurs in metastatic breast cancer because it increases the migration and invasion capabilities of cancer cells. EMT is a transdifferentiation process involved in wound healing in many organisms [14]. EMT has been evolutionarily conserved and cancer cells have adapted this function to increase stemness and promote metastasis [14].
EMT master regulators, including the zinc finger E-box binding homeobox (Zeb), Twist, and Snail families of transcription factors, are largely responsible for tumor cell dissemination and chemotherapeutic resistance through their regulatory function in the EMT/mesenchymal-epithelial transition (MET) pathways [10, 11]. These transcription factors induce EMT when overexpressed in epithelial cells, often through positive feedback loops and crosstalk [13, 14]. Overexpression of SNAI1 activates SNAI2, ZEB1/2, TWIST1, and TCF4, SNAI2 activates TWIST1, and ZEB1 and ZEB2 activate each other [14].
Conditional knockout in human mammary epithelial cells was used in one study to identify ZEB1/2 as metastasis regulators [14]. In the same study, Dox-inducible short hairpin RNAs (shRNAs) were used to show that suppression of EMT through depletion of ZEB1/2 blocked spontaneous lung metastasis [14]. ZEB1 represses the expression of E-cadherin, a cell-cell adhesion protein, and transcription of epithelial polarity genes, allowing for increased metastasis in tumors with high ZEB1 expression [15]. ZEB1 downregulates epithelial markers and upregulates mesenchymal markers while also making them more like stem cells, allowing for drug and therapy resistance [13]. The ZEB family members both contain C2H2-type zinc fingers, the common DNA-binding motifs binding to paired CAGGTA/G E-box-like elements in the promoters of their target genes [13]. Cancer cells can activate DNA repair and survival signaling pathways to bypass therapies when ZEB1 increases their stemness, making them more like cancer stem cells [15].
Twist1 promotes malignant tumor transformation by degrading p53 in order to bypass apoptosis and oncogene-induced aging [16]. Crosstalk between the PI3K/Akt and transforming growth factor beta (TGFβ) pathways is enabled by Twist phosphorylation, causing upregulation of the TGFβ2 gene, an EMT activator [17]. Twist family members are also associated with the EMT-reversal process, MET [17]. In this process, mesenchymal cells regain epithelial characteristics after EMT. MET is important to distant metastases (Figure 1) [16].
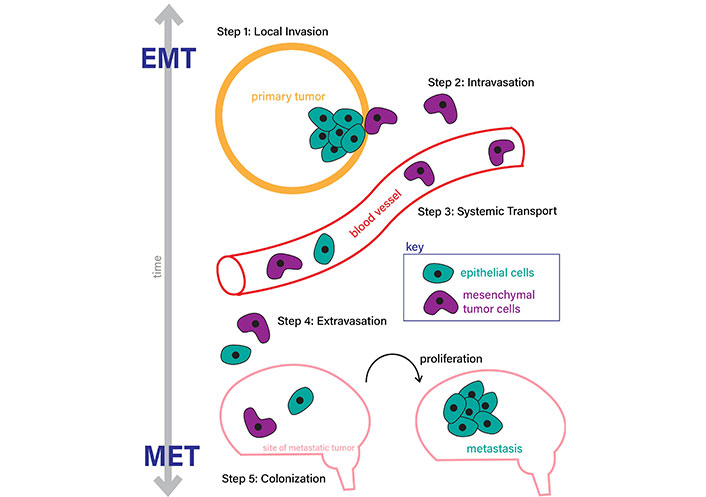
Epithelial-mesenchymal transition (EMT) and mesenchymal-epithelial transition (MET) processes. EMT occurs through (step 1) local invasion and formation of the initial tumor, (step 2) intravasation to enter the bloodstream, (step 3) systematic transport to a metastatic site, usually another organ, (step 4) extravasation out of the bloodstream, and (step 5) colonization and formation of a metastatic tumor through cell proliferation. MET is the reversal process, which occurs in the same steps in the reverse order as labeled in this figure (steps 5 to 1)
Note. Adapted from “Epithelial–mesenchymal plasticity in carcinoma metastasis” by Tsai JH, Yang J. Genes Dev. 2013;27:2192–206 (https://genesdev.cshlp.org/content/27/20/2192.long). CC BY-NC.
The Snail family consists of two members: Snail1/Snail and Snail2/Slug [18]. Snail is a transcriptional repressor of CDH1, the E-cadherin gene, which links Snail to EMT as low E-cadherin expression levels are indicative of the transition to the mesenchymal phenotype [18]. Additionally, cells with Snail overexpression experience lower frequencies of apoptosis when exposed to radiation and chemotherapies, and they have increased immunosuppression [18].
HIF-1α is a clear target for treating TN breast cancer, as it has been proven to induce and promote breast cancer metastasis by regulating EMT, degrading extracellular matrix, inducing pre-metastatic niche, and regulating the directional movement of TNBC cells by transcribing integrins [19].
However, Antón-García et al. [20] have found experimental evidence that SNAI1 and ZEB1 may not be considered EMT master regulators because TGFβ1-induced EMT occurs independently of either transcription factor.
Other transcription factors
There are a multitude of transcription factors involved in breast cancer, and current research has only explored the mechanisms of a limited few.
SOX family
Sex determining region Y (SRY)-related high-mobility group (HMG) box (SOX) genes code for DNA-binding proteins that help regulate cell differentiation, organogenesis, and other developmental processes [21]. The SOX family contains more than 20 members in vertebrates, which are classified into eight groups [21]. SOX transcription factors are involved in cell determination and regulation of cell plasticity in normal cells, but in cancer cells, these processes are abnormally regulated, leading to the promotion of tumor heterogeneity and metastasis [22]. SOX4 contributes to breast cancer metastasis by activating the TGFβ pathway and promoting the expression of EMT inducers [21]. SOX2 expression is positively correlated with tumor size and promotes angiogenesis to enable lymph node metastasis [21, 23]. SOX9 accumulation in the cytoplasm increased proliferation, migration, and invasion, and targeting this gene showed less metastasis [21]. The SOX gene family transcription factors are other potential targets for treatment due to their roles in metastatic processes.
ETS family
The E26 transformation-specific (ETS) family is a large transcription factor family consisting of 27 ETS genes in humans which can be structurally categorized into 11 subfamilies [24]. The ETS family of transcription factors regulates signaling, development, apoptosis, and metastatic processes [24]. Increased expression of ETS transcription factors has been observed in invasive and metastatic breast tumors and indicates poor prognosis [24]. The ETS family plays a role in breast cancer metastasis by affecting genes such as HER2 and VEGF that are related to steps in the metastatic process such as proliferation, transformation, migration, invasion, anti-apoptosis, and angiogenesis [24]. A subgroup in the ETS family called the PEA3 group is overexpressed in both human and mouse mammary tumors [25]. PEA3 overexpression increases invasion and migration and is positively correlated with HER2/neu expression in 25–30% of HER2-positive breast cancers [24]. ETS1 overexpresses Long Intergenic Non-protein Coding RNA 1016 (LINC01016), suppressing some ubiquitination degradation in breast cancer cells [26]. ETS1 is correlated with invasiveness, EMT, and angiogenesis, and ETS2 is correlated with apoptosis, so these transcription factors also impact breast cancer metastasis through differential regulation of metastatic processes [24].
Yin Yang 1
Yin Yang 1 (YY1) is a transcription factor often upregulated in many cancers, including metastatic breast cancer [27]. YY1 is negatively correlated with the survival of breast cancer patients and positively correlated with cancer stem cell markers such as SOX2, OCT4, BMI1, and NANOG, all of which are related to drug resistance [27, 28]. YY1 regulates BRCA1 and HER2 tumorigenic pathways to promote tumor angiogenesis and metastasis [28]. The main contribution of YY1 to metastatic breast cancer is its ability to increase cancer stem cell qualities in tumors, which helps enable metastasis.
FoxM1
Forkhead box M1 (FoxM1) is a transcription factor that regulates the cell cycle and mitotic spindle formation in normal cell growth and carcinogenesis by promoting cell motility, invasion, EMT, and metastasis [29]. FoxM1 expression is associated with increased tumor size, lymphovascular invasion, and lymph node metastases [29]. FoxM1 has been implicated in each stage of metastasis by promoting EMT, activating the TGFβ pathway, and modulating the extracellular matrix through the regulation of the other genes [29, 30]. Because of the evident role of FoxM1 in all breast cancer stages and in all breast cancer subtypes, it is a promising potential therapeutic target to block [31, 32].
Bach1
BTB domain and CNC homology 1 (Bach1) is a transcription factor with increased expression in breast cancer cells [33]. Bach1 is a known regulator of heme homeostasis, oxidative stress response, mitochondrial metabolic processes, and the cell cycle [33]. Overexpression of Bach1 promotes migration, invasion, and hypoxia-related pathways [34]. Specifically, in TN breast cancer cells, Bach1 regulates pathways and the expression of genes involved in cellular respiration, affecting the survival of cancer cells [33]. Bach1, like many transcription factors, proves to be a useful target for the treatment and prognosis of metastatic breast cancer.
SIX family
The sineoculis homeobox homolog (SIX) family of transcription factors has six members which are important to cell proliferation, differentiation, apoptosis, adhesion, and migration [35]. One study found that expression levels of SIX1, SIX2, SIX3, and SIX4 were increased in breast cancer cells as compared to low expression in non-cancerous cells [35]. SIX1 promotes invasion and metastasis by enabling EMT through downregulation of E-cadherin, an epithelial marker, promotes angiogenesis through regulation of VEGF, and promotes Ezrin expression, which plays a role in cell adhesion [35, 36]. SIX2 upregulates ZEB2 to downregulate E-cadherin, both of which are related to EMT and MET [35]. SIX1 knockout in animal models has decreased tumor size [36].
RUNX2
The runt-related transcription factor (RUNX) family of transcription factors has three members, each with different physiological and developmental roles [37]. RUNX1 and RUNX3 act as tumor suppressors in breast cancer cells, while RUNX2 can act as either a tumor suppressor or an oncogene in cancer cells [37]. Expression levels of RUNX2 are increased in breast cancer tumors [38]. RUNX2 is involved in responding to DNA damage, regulating VEGF, and in several estrogen signaling pathways and the TGFβ pathway [38]. RUNX2 is important to osteogenesis and bone formation, so it is linked to breast cancer metastasis in the bone as it allows cancer cells to execute osteomimicry and invade bone composition [38].
MYC
The myelocytomatosis (MYC) oncogene family consists of three members, C-MYC, L-MYC, and N-MYC, all of which belong to the superfamily of bHLH leucine zipper (bHLHLZ) DNA binding proteins [39]. MYC proteins regulate a wide range of genes involved in several cellular processes, including cell growth, differentiation, apoptosis, angiogenesis, DNA repair, protein translation, immune response, and stem cell formation [39]. These regulatory functions suggest that MYC can contribute to cancer by enhancing cell proliferation, inhibiting cell death, modulating metabolism, promoting angiogenesis, and regulating stem cell formation [39]. Amplification of c-Myc and the activation of pathways downstream are related to high metastatic ability in breast tumors [40]. Myc-amplification-mediated glutamine-related metabolic pathways are upregulated in the luminal B, HER2-positive, and TN breast cancers [40].
KLFs
Kruppel-like factors (KLFs), a subgroup of the Sp1/KLF family, contains seventeen members, each with three C2H2-type zinc fingers [41]. KLFs play roles in cell proliferation, apoptosis, EMT, and invasion and are connected to pathways involving E2F, MYC, and p53 [41]. Most KLFs are ubiquitously expressed, while others have cell- or tissue-specific expression [41]. KLFs regulate metastatic processes both positively and negatively. KLF8 promotes but KLF6 and KLF9 inhibit changes in the extracellular matrix, KLF8 stimulates and KLF8 and KLF 9 downregulate invasion, KLF4, KLF 5, and KLF17 restrain and KLF8, KLF6-splice variant (SV), and KLF16 promote EMT [41–43]. KLF11 in tumor cells promotes tumor growth [44]. KLF9 represses breast cancer invasion and metastasis by upregulating E-cadherin [42]. KLF16 deletion was found to suppress EMT, migration, and invasion in breast cancer [43].
c-Jun
c-Jun is a protein encoded by the proto-oncogene JUN [45]. c-Jun is involved in several processes in breast cancer including proliferation, differentiation, growth, apoptosis, cell migration, and transformation [45]. c-Jun directly binds to and activates at least four genes associated with breast cancer metastasis to the lungs [45]. c-Jun is closely associated with metastasis and acts as both suppressor and oncogene in different situations [46]. c-Jun is also related to glucose metabolism, which may be a mechanism for metastasis based on how c-Jun is related to the expression of glucose transporter protein type 1 (GLUT1), a membranous glucose transporter [46]. c-Jun promotes cell growth in breast cancer by interacting with estrogen receptors [47].
Metastatic processes as dictated by transcription factors
Metastasis occurs through invasion, intravasation, transport, extravasation, and colonization [24]. Transcription factors enable these steps through other processes: survival, angiogenesis, and EMT (Figure 2). Transcription factors do not always impact all of these processes, and studying which ones are affected by each transcription factor will give insight into how to implement them in therapeutic strategies.
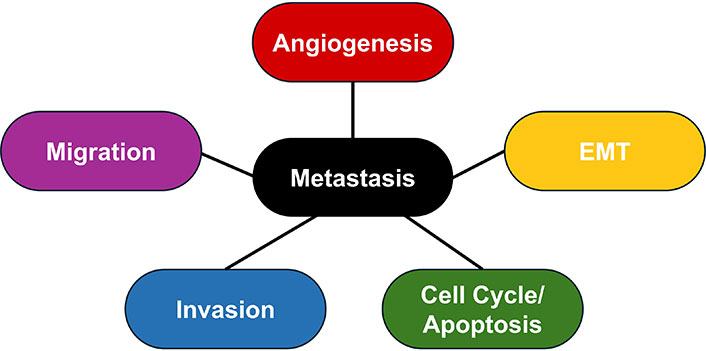
Metastatic processes. The main processes contributing to metastasis are angiogenesis, epithelial-mesenchymal transition (EMT), abnormal regulation of the cell cycle and apoptosis, invasion of tissue, and migration of tumor cells
Survival of tumor cells
Survival of primary tumor cells is crucial to metastasis, especially in the colonization step, as only the cancer cells able to survive in hypoxic conditions, against the human immune system, or against different therapies can go on to form metastases.
E2Fs contribute to tumor cell survival by also promoting cell proliferation by regulating the cell cycle. EMT transcription factors are associated with increased tumor survival and metastatic success because the EMT process often yields cancer stem cells with resistance to chemotherapy, possibly because these cells have a slower cell cycle than the rapidly dividing cells many therapies target [48]. HIFs play a large role in metastasis by providing blood to secondary tumors that would otherwise go through necrosis in deficient blood supply [49].
Angiogenesis and vascularization
Angiogenesis is the development of blood vessels, a process integral to the growth and metastatic capacity of malignant tumors. By increasing angiogenesis, tumors are recruiting their own blood supply to get more oxygen and nutrients to their cells. Vascularization uses angiogenesis to supply oxygen to new areas through blood. HIFs are major transcription factors related to these processes in the cellular response to hypoxia. Activation of the HIF pathway often occurs in breast cancer to stimulate the growth of blood vessels. The concentration of microvessels in a breast cancer sample proves to be an accurate indicator of metastasis or relapse [49].
Angiogenesis in breast cancer tumors results in new vasculature that is disorganized and incomplete compared to angiogenesis in normal tissues [7]. This causes decreased drug delivery and hinders antitumor immune responses, contributing to drug resistance [7].
In terms of metastatic cancer, intravasation and extravasation describe the movement of cancer cells into and out of blood vessels, respectively. These processes are integral to how cancer cells travel through the circulatory or lymphatic system to reach distant tissues. Expression of Zeb1 and Snail1 was found to promote intravasation and transmigration [16].
EMT and migration
Invasion refers to the penetration of neighboring tissues by cancer cells. EMT is one way that cancer cells leave the primary tumor to distant metastatic sites in the body. The transition to mesenchymal phenotype gives tumor cells stem-cell-like qualities. During EMT, the extracellular matrix is degraded to allow for invasion, when cancer cells first leave the primary tumor and enter a neighboring tissue [16]. There are three types of EMT representing different processes, and the regulation of all of them is similar [50]. The main pathways regulating EMT are the Notch, Wnt, Hedgehog (Hh), and TGFβ pathways [51]. One crucial EMT process is the loss of E-cadherin, a protein that maintains cell-cell contacts, which is downregulated by transcription factors like Snail, Slug, Ras homolog gene family, member B (RhoB), and TWIST1 [41].
Snail, Zeb, and Twist family transcription factors promote EMT pathways, making them potential therapeutic targets. Six1 and RunX2 also drive EMT [52]. HIFs transactivate Snail transcription factors and directly regulate Twist and Zeb [53]. HIF-1α-induced EMT can be eliminated through inhibiting ZEB1 [53].
Another effect of EMT is the increased resistance to chemotherapy acquired by breast cancer stem cells in the process as they have increased expression of anti-apoptotic and DNA repair genes [54].
MET, the reverse process of EMT, is important to the colonization step of metastasis to establish secondary tumors [16]. MET at metastatic sites is induced by the re-expression of E-cadherin, giving these secondary tumor cells greater susceptibility to chemotherapy [55].
Targeting transcription factors
Targeting transcription factors can block EMT, immune evasion, resistance, and stem cell properties, and can increase cell death and interfere with autoregulatory pathways in cancer cells [52].
Treatment
The most successful cancer treatment strategy targeting transcription factors so far has been through nuclear hormone receptor ligand binding domains, in other words, molecules that bind to specific receptors [52]. Drugs currently used to treat breast cancer affect the estrogen receptor, androgen receptor, retinoic acid receptor, and glucocorticoid receptor [52]. The estrogen receptor is a particularly effective target as approximately 75% of breast cancers express it [52]. Widely used current breast cancer therapies include tamoxifen, a selective estrogen receptor modulator, and combination chemotherapy with trastuzumab to target HER2 and bevacizumab to target VEGF [12].
Targeting protein-protein interactions between transcription factors, co-activators, and co-repressors impacts gene expression [52]. The interaction between RUNX1 and ETS1, for example, occurs for DNA binding [52]. This strategy involves using inhibitors to block the proteins. These inhibitors can be small compounds, peptidomimetics, or stapled helix peptides [32].
Transcription factors are naturally regulated in cells through ubiquitylation and proteasomal degradation [52]. E3 ubiquitin ligases drive these processes [52]. One successful example is an inhibitor of the E3 ubiquitin ligase called von-Hippel Lindau (VHL) which interacts with the HIF-1α transcription factor [52]. Deubiquitinases prevent degradation; for example, ubiquitin specific peptidase 10 (USP10) protects SLUG and SNAI2 from degradation, and DUB3 increases SNAI1 while protecting TWIST and SLUG [52].
Proteolysis targeting chimeras (PROTACs) can also degrade transcription factors [52]. Using PROTACs involves a ligand that binds to an E3 ligase covalently attached to a ligand that binds to a specific protein, which drives ubiquitylation and subsequent proteasome-mediated destruction [52]. PROTACs are a promising treatment method because they can knock down all functions of a transcription factor through complete degradation that may not be possible with inhibitory substances, which may also require lower dosage and improved efficacy [52].
The binding pockets of transcription factors can be targeted to prevent them from initiation transcription. Some transcription factors involve ligands, so one approach to inhibit these is to develop structural derivatives of the natural ligands for that transcription factor [32]. Most oncogenic transcription factors do not have these ligands, so a more effective approach for those would be to target the DNA-binding pocket of the protein through other protein interactions or direct binding to the DNA [32].
Biomarkers
Biomarkers are measured substances used to evaluate normal processes or responses to a treatment that can be prognostic and predictive [7]. Transcription factors can not only be targeted directly for treatment but they can be evaluated for diagnostic and prognostic purposes as biomarkers. Measuring and comparing levels of transcription factors can allow for correlation between those levels and metastasis, patient survival, etc.
Conclusions
Transcription factors enable invasion, intravasation, transport, extravasation, and colonization of metastasis through other processes such as angiogenesis and EMT, making them a prime target for cancer treatment. Treatment targeting transcription factors is possible because of their roles as master regulators, including E2Fs, HIFs, and EMT master regulators. These transcription factors can also be markers for breast cancer and metastatic prognosis. Several other transcription factors have also been identified to play a role in metastatic breast cancer, but this paper reviews the roles of E2Fs, HIFs, EMT master regulators, SOX, ETS, YY1, FoxM1, Bach1, SIX, RUNX2, MYC, KLFs, and c-Jun.
Transcription factors have diverse and crucial roles in metastatic breast cancer, as well as in every genetic illness. The possibilities for treatment and prevention are vast due to the multitude of transcription factors involved, so research focusing on promising transcription factors should be done, especially because metastasis causes 90 percent of breast cancer deaths [6]. The multitude of transcription factors that target processes integral to breast cancer metastasis and tumor progression provide further insight into the pathways and processes that should be targeted when aiming to treat breast cancer. Most known transcription factors do not have singular or concrete roles and functions, but impact metastasis through several processes (Table 1). Additional transcription factors can be potential treatment targets with a less direct approach, as there are several that impact cancer progression by regulating more than one related gene.
Summary of the discussed metastatic processes of breast cancer affected by various transcription factors
Transcription factor | Metastatic process affected | ||||
---|---|---|---|---|---|
Angiogenesis/Vascularization | EMT/MET | Migration | Invasion | Cell cycle/Apoptosis | |
E2F | √ | √ | √ | ||
HIF | √ | √ | √ | ||
EMT master regulators | √ | √ | √ | √ | |
SOX | √ | √ | √ | √ | |
ETS | √ | √ | √ | √ | √ |
YY1 | √ | ||||
FoxM1 | √ | √ | √ | ||
Bach1 | √ | √ | √ | ||
SIX | √ | √ | |||
RUNX | √ | ||||
MYC | √ | √ | |||
KLFs | √ | √ | √ | √ | |
c-Jun | √ | √ |
EMT: epithelial-mesenchymal transition; MET: mesenchymal-epithelial transition; HIF: hypoxia-inducible factor; SOX: sex determining region Y-related high-mobility group box; ETS: E26 transformation-specific; YY1: Yin Yang 1; FoxM1: forkhead box M1; Bach1: BTB domain and CNC homology 1; SIX: sineoculis homeobox homolog; MYC: myelocytomatosis; KLFs: Kruppel-like factors; RUNX: runt-related transcription factor
Current gaps in research include studies on combination therapies, such as targeting more than one transcription factor at once to increase effectiveness. Further research on tumor microenvironments and tumor heterogeneity in relation to transcription should be conducted because these topics are crucial to understanding therapy resistance in metastatic breast cancer. Since primary and secondary tumors possess different qualities, one transcription factor may only be partially effective for a patient.
The main takeaway is that metastasis is largely controlled by transcription factors, making them a target for the treatment of this deadly condition in breast cancer patients.
Abbreviations
Bach1: | BTB domain and CNC homology 1 |
bHLH: | basic helix-loop-helix |
CDK: | cyclin-dependent kinase |
EMT: | epithelial-mesenchymal transition |
ETS: | E26 transformation-specific |
FoxM1: | forkhead box M1 |
Her2: | human epidermal growth factor receptor 2 |
HIFs: | hypoxia-inducible factors |
KLFs: | Kruppel-like factors |
MET: | mesenchymal-epithelial transition |
MYC: | myelocytomatosis |
PROTACs: | proteolysis targeting chimeras |
RUNX2: | runt-related transcription factor 2 |
SIX: | sineoculis homeobox homolog |
SOX: | sex determining region Y-related high-mobility group box |
TGFβ: | transforming growth factor beta |
TN: | triple-negative |
VEGF: | vascular endothelial growth factor |
YY1: | Yin Yang 1 |
Zeb: | zinc finger E-box binding homeobox |
Declarations
Author contributions
SM: Conceptualization, Investigation, Visualization, Writing—original draft, Writing—review & editing. CM: Conceptualization, Funding acquisition, Supervision. YL: Project administration, Validation, Supervision. All authors read and approved the submitted version.
Conflicts of interest
The authors declare that there are no conflicts of interest.
Ethical approval
Not applicable.
Consent to participate
Not applicable.
Consent to publication
Not applicable.
Availability of data and materials
Not applicable.
Funding
This paper was funded by The Assisi Foundation of Memphis, Brown, Chester, PhD (PI). The funders had no role in study design, data collection and analysis, decision to publish, or preparation of the manuscript.
Copyright
© The Author(s) 2024.