Abstract
Melatonin is the primary hormone of the pineal gland that is secreted at night. It regulates many physiological functions, including the sleep-wake cycle, gonadal activity, free radical scavenging, immunomodulation, neuro-protection, and cancer progression. The precise functions of melatonin are mediated by guanosine triphosphate (GTP)-binding protein (G-protein) coupled melatonin receptor 1 (MT1) and MT2 receptors. However, nuclear receptors are also associated with melatonin activity. Circadian rhythm disruption, shift work, and light exposure at night hamper melatonin production. Impaired melatonin level promotes various pathophysiological changes, including cancer. In our modern society, breast cancer is a serious problem throughout the world. Several studies have been indicated the link between low levels of melatonin and breast cancer development. Melatonin has oncostatic properties in breast cancer cells. This indolamine advances apoptosis, which arrests the cell cycle and regulates metabolic activity. Moreover, melatonin increases the treatment efficacy of cancer and can be used as an adjuvant with chemotherapeutic agents.
Keywords
Melatonin, breast cancer, cell cycle, apoptosis, chemotherapyIntroduction
It is well-known that the hormone melatonin has oncostatic effects both in vivo and in vitro conditions in various types of cancers, including breast. Melatonin can disrupt estrogen-mediated cellular pathways, ensuing in a lattice drop of estrogenic stimulus to the breast cancer cells. Studies have shown that sleep and/or circadian disruption, specifically night shift work, chronic jet lag, trans-Mediterranean traveling are correlated with an increased risk of breast cancer development.
Melatonin is an indolamine (N-acetyl-5-methoxytryptamine), identified by Lerner et al. [1] from the mammalian pineal gland. The precursor of melatonin is serotonin, a potent neurotransmitter. The environmental light-dark cycle is the potent regulator of melatonin synthesis. The suprachiasmatic nucleus (SCN) of the hypothalamus receives photoperiodic information and regulates the synthesis and secretion of melatonin through a complex neural connection [2, 3]. Melatonin is the modulator of the central circadian clock and peripheral clock of the different tissues, including the breast [4]. Many laboratories have focused on the functions of the clock genes and proteins that are involved in cellular activities, including cell survival, cell cycle regulation, proliferation, apoptosis, DNA damage repair, and tumor promotion or suppression [5, 6]. With the synchronized output of the central circadian clock in relation to the pineal gland and other peripheral oscillators, melatonin acts as a key role player in the regulation of intermediary metabolism and cancer prevention [7]. Several studies have revealed that circadian rhythm disruption, specifically night shift work is linked with an increased risk of breast cancer [8]. Impaired melatonin level promotes breast, ovarian, prostate, hepatocellular, and other cancers. Melatonin has oncostatic properties that are mediated by induction of apoptosis, arresting the cell cycle, regulation of metabolic activity, and the inhibition of hypoxia-inducible factor 1α (HIF-1α) activity [9]. Excessive exposure to estrogen is a key risk factor for breast cancer [10]. Melatonin primarily regulates steroidogenesis and shows anti-gonadal activity.
Numerous studies had revealed that the increased rate of night shift works suppresses melatonin production and increases the risk of estrogenic stimulation for breast cancer development. An inverse correlation has been observed between melatonin and the risk of development of breast cancer. Melatonin levels are low in women with breast cancer [11]. Additionally, maintenance of the light-dark cycle and oral supplementation of melatonin may have a beneficial effect on women who are facing the risk of breast cancer [12]. This review will touch the oncostatic effects of melatonin in breast cancer and its possibilities in cancer treatment.
Synthesis of melatonin
The pineal gland is the primary site of melatonin synthesis. It begins with the conversion of tryptophan to 5-hydroxy-tryptophan, which is then converted to serotonin, followed by melatonin after methylation (Figure 1) [13]. Melatonin production is increased by induction of the rate-limiting enzyme arylalkylamine N-acetyl transferase (AANAT) [14]. The expression of this enzyme is regulated by the exposure of light to the retina. Photoperiod regulates the synthesis and secretion of melatonin. Light exposure in the daytime represses melatonin production, while darkness acts as an inducer. The peak of melatonin production occurs at about 2 a.m. in humans [15]. Alternatively, light-at-night (LAN) inhibits melatonin synthesis [16]. Multiple studies indicated that pinealectomy or circadian disruption can stimulate spontaneous growth and development of tumors, and metastasis of the existing tumors [17, 18]. In addition, melatonin is also synthesized in extra-pineal organs, including the retina, gastrointestinal tract, skin, lymphocytes, and bone marrow [19].
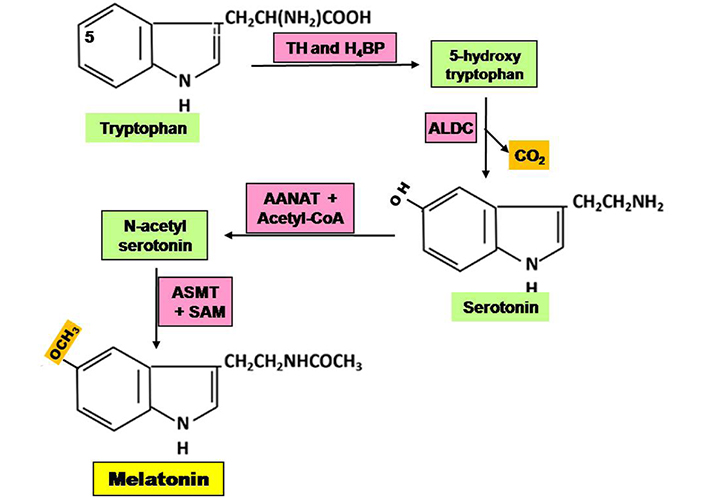
Steps of synthesis of melatonin in pineal gland. ALDC: aromatic L-amino acid decarboxylase; ASMT: acetylserotonin O-methyl transferase; H4BP: tetra hydrobiopterin; SAM: S-adenosyl methionine; TH: tryptophan hydroxylase
Mechanisms of actions
Melatonin is a lipophilic compound. Its action is mediated primarily through binding to the cell surface melatonin receptors (MT1, MT2). These are membrane-bound GTP-binding protein (G-protein) coupled receptors (GPCRs). They modulate the activity of the variety of G-proteins, including Giα2, Giα3, Gαq, and Gα11 [20, 21]. Moreover, melatonin acts directly to the DNA binding nuclear receptors (NRs) [retinoid-related orphan receptor (ROR)-α/isoforms of nuclear receptors of retinoic acid receptor superfamily (RZR)]. In humans and other mammals, the MT1 receptor is encoded by the MTNR1A gene and the MTNR1B gene is responsible for the MT2 receptor [22, 23]. The MT1 receptor inhibits the production of cyclic adenosine monophosphate (cAMP) via pertussis toxin-sensitive inhibitory Giα proteins [20, 24]. Activated MT1 receptor helps in the inhibition of forskolin-stimulated cAMP formation, followed by suppression of protein kinase A (PKA) activity and phosphorylation of the cAMP-responsive element-binding protein (CREB) [25, 26]. Melatonin-mediated activation of the MT1 receptor has also been involved in the modulation of ion channels in cells [26].
In addition, the MT2 receptor has also been associated with G-proteins to decrease forskolin-activated cAMP production. It modulates cyclic guanosine monophosphate (cGMP) formation and amplifies PKC activity [27, 28]. The MT1- and MT2-mediated melatonin signaling is enhanced by the formation of homo- or hetero-dimmers for the modification of receptor functions and activity [29, 30]. Furthermore, G-protein-coupled receptor 50 (GPR50), a melatonin-related receptor is incapable to bind with melatonin but can dimerize with MT1 and MT2 receptors that only repress the affinity of MT1 [31]. The activation of the MT1 receptor shows much of melatonin’s oncostatic actions, resulting in the net reduction of estrogenic stimulation in estrogen receptor-α (ER-α)-positive MCF-7 human breast cancer cells [32].
Studies with MCF-7 human breast cancer cells revealed that melatonin activates the Giα2-, Giα3-, Gαq-, and Gα11-coupled MT1 receptors [33]. MT1 receptor overexpression can enhance the anti-proliferative effect of melatonin on breast cancer cells in both in vivo and in vitro conditions. Application of non-selective MT1 and MT2 receptor antagonists showed reverse effects [30, 34]. Experimental evidence from breast tumor biopsy specimens had revealed that there was a correlation between the MT1 receptor activity and ER-α expression [35]. Besides these, both MT1 positive and triple-negative breast cancers (TNBC) exhibited a lower proliferative rate with smaller tumor size, while MT1 negative TNBC was notably linked with a higher risk of progression of breast cancer [36]. To date, all studies have indicated the MT1 expression with an improved prognosis compared to those with MT1 negative breast tumors [25, 37].
Melatonin can cross the cell membrane due to its lipophilic nature. At the cytosolic site, it binds with the nuclear and mitochondrial proteins to drive a variety of non-receptor-mediated effects in breast cancer cells. Several studies had revealed that melatonin binds to the calmodulin (CaM) receptor, which decreased the sensitivity of adenylate cyclase (AC) [38]. Inactivated AC lowers cAMP levels within cells that can downregulate the activation of PKA, CREB, and p300 co-regulator; the collective effects are attenuation of phosphorylation and trans-activation of different transcription factors and NRs, including ER-α [11].
It had also been reported that melatonin acts as a potent free radical scavenger. The activity is mediated by MT3, a quinine-reductases. The subsequent result is diminution of reactive oxygen species (ROS)-mediated oxidative damage in various tissues, including the breast [39]. In in vitro study, administration of melatonin in ZR-75-1 and MCF-7 breast cancer cells triggered the expression of glutathione and glutathione-S-transferase that decrease tumor cell metabolism and proliferation [25]. Melatonin shows receptor-mediated immunomodulatory effects in the immune system [40] and reduces telomerase activity [41].
Anti-proliferative effects of melatonin on breast cancer cells
Animal studies and clinical data had revealed that melatonin decreases the occurrence of in vitro tumor cell-induced cancers [11]. It also significantly inhibits the growth of some human breast tumors [25, 42]. Melatonin exerts both anti-proliferative and apoptotic effects in breast cancer cells in multiple ways (Figure 2) [25, 43]. The anti-proliferative functions of melatonin are mediated by the activation of the MT1 receptor in MCF-7 cells-induced transfection model [34, 44]. Melatonin receptor suppresses ER-α-mediated transcriptional activity in ER-α-positive breast tumor cells. The arrest of cell cycle and the cell proliferation are mediated by the inhibitory actions on Ca2+ signaling and CaM expression [25], stimulation of p53 expression [45], and p21-gene transcription (Figure 2) [46]. It was reported that blocking of MT1/MT2 receptors in MCF-7 cells showed impaired p53-mediated repairing of DNA damage [47]. This indolamine arrests the cell cycle of breast tumor cells in the gap phase 1 (G1) phase [41], prevents estrogen synthesis by inhibiting aromatase activity [34, 48]. Melatonin also suppresses the uptake of linoleic acid (LA) in the tumor cells [49].
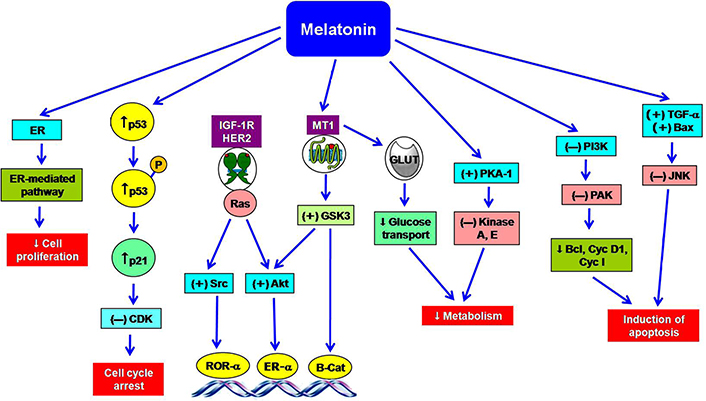
The multiple actions of melatonin in the prevention of breast cancer. ↑: increase; ↓: decrease; (+): stimulation; (–): inhibition; Akt: protein kinase B; Bax: B-cell lymphoma-2 associated X protein; Bcl: B-cell lymphoma; B-Cat: beta catenin; CDK: cyclin dependent kinase; Cyc I: cyclin I; Cyc D1: cyclin D1; GLUT: glucose transporter; GSK3: glycogen synthase kinase 3; HER2: human epidermal growth factor receptor 2; IGF-1R: insulin like growth factor 1 receptor; JNK: Jun N-terminal kinase; P: protein; PAK: p21 activating kinase; PI3K: phosphoinositide 3-kinase; Ras: rat sarcoma virus; Src: sarcoma; TGF: transforming growth factor
Modulation of estrogen-ER-α-signaling pathway
Under the natural photoperiod, melatonin is expected to be a regulator of reproduction in seasonally breeding animals [50]. It modulates the hypothalamic-pituitary-gonadal axis (HPGA) [51], resulting in the decrease of ovarian estrogen synthesis [25]. However, humans are not seasonal breeders and melatonin exercises some modulatory actions on steroidogenesis in human luteal cells [52, 53].
The early report indicated that melatonin downregulated the synthesis of sex steroids, particularly estrogen. Thus, melatonin has a negative impact on estrogen-signaling [54]. Melatonin suppressed estrogen-mediated proliferation of human breast cancer cells in in vitro system. Hill et al. [25, 34] suggested that melatonin regulates the estrogenic actions on breast and mammary tissue in three ways: (i) diminution of synthesis of sex steroids, including estrogens and reduction of their circulating levels; (ii) binding with ER-α as a selective ER modulator (SERM) to inhibit estrogen binding, nuclear translocation, DNA-binding, and transcriptional activation; (iii) decreasing the activity of the enzymes such as aromatase, sulfatase, and aldo-keto reductases (AKRs) involved in the synthesis of estrogens from cholesterol. Administration of melatonin might be a key inhibitor of breast cancer. The cell line study indicated that 1 nmol/L solution of melatonin can repress the proliferation of ER-α-positive human breast cancer as well as suppression of the ER-α mRNA expression [25].
Melatonin is a powerful repressor of estrogen-induced ER-α-dependent transcriptional activities. It inhibits several estrogen-induced mitogenic expressions and anti-apoptotic gene transcription including Bcl-2. Alternatively, melatonin influences the expression of growth-inhibitory and pro-apoptotic genes like TGF-α and Bax [55]. Melatonin-Gαi2 protein suppresses cAMP production, followed by a diminution of PKA levels, leading to impaired phosphorylation of serine-236 of the ER-α. This PKA-sensitive site of ER-α plays a crucial role in the inhibition of ER-α-dependent transcriptional activities [20, 25]. Melatonin can couple with CaM and suppresses ER-α-mediated functions by modulating the PKA-driven Ca2+/CaM pathway.
A chromatography-mediated immunoprecipitation study indicated that melatonin blocked 17-β estradiol-mediated recruitment of CaM and p300 to the Cyc D1 promoter [25]. The absence of estrogenic activity shows the significant declining effects due to less recruitment of p300 to the Cyc D1 promoter. Reverse action had been observed within 1.5 h after administration of estrogen. The daily rhythm of estrogen peak arises in the early morning which is essential for the estrogenic activity [25]. Generally, plasma melatonin peak reaches 2 a.m. followed by the decreasing effect. Thus, estrogen levels begin to rise when the melatonin peak is in a downward step [56].
It was reported that dim light exposure at night (dLEN) hampers the melatonin functions and circadian signal. Circadian dysfunction induced the phosphorylation of the ER-α at Ser-118 and Ser-167 through the action of extracellular receptor kinase (ERK)-1 and ERK-2, cellular-Src (c-Src) kinase, and protein kinase B (Akt) [25, 49]. During dLEN, exogenous supplementation of melatonin significantly suppresses the phosphorylation of ER-α. This evidence strongly supports the role of melatonin on the regulation of gene expression in human breast cancer cells via the actions of the MT1-ER-α receptor-specific mechanism. However, contradictory findings in relation to night shift work and the incidence of cancer are also available. The meta-analysis study revealed that the development of cancer is not influenced by fixed time work or rotational night shift work. In this concern, the negative result had also been obtained from Asian people. The risk of cancer was low in Asians compared to Americans and Europeans due to their distinct food habits, lifestyles, and gene pools [57].
Expression of other NRs in human breast cancer cells by melatonin
The decrease of ER-α expression also regulates the transcriptional activity of other steroid hormones/NR super-family receptors. It is evident that melatonin can repress the ligand-induced expression of the glucocorticoid receptor (GR) and ROR-α in breast cancer cells [25, 58]. Alternatively, melatonin can enhance the expression of other NRs, including retinoic acid X receptor alpha (RXRα) and the retinoic acid receptor alpha (RARα) [25, 59].
Dauchy et al. [60] reported that melatonin administration during dLEN-mediated circadian disruption induced the expression and phosphorylation of many kinases (Akt, ERK1/2, FAK, PKA, Src, etc.) that regulate the phosphorylation of NRs and other transcriptional factors (Ap-1, Elk-1, NF-κB, STAT3, etc). This event clearly expresses the importance of melatonin in the regulation of gene expression in human breast cancer cells. Furthermore, administration of melatonin along with all-trans-retinoic acid (RXRα and RARα) in MCF-7 cells induced the rate of apoptosis, which is mediated by increased expression of pro-apoptotic protein Bax and downregulation of TGF-β1 and Bcl-2 (anti-apoptotic protein) expression [61]. In addition, the combination of 9-cis retinoic acid and melatonin can inhibit the progression of N-nitroso-N-methyl urea (NMU)-induced breast tumors in rats [25, 54]. In the presence of vitamin D, melatonin can promote vitamin D receptor (VDR) transcriptional activity and drive the MCF-7 breast tumor cells towards apoptosis [62, 63].
Melatonin induces cytotoxicity in breast cancer cells
The pharmacologic concentration of melatonin shows cytotoxic effects in breast cancer cells. The experimental reports revealed that physiologic concentrations of melatonin suppressed the in vitro proliferation of breast cancer cells by disrupting the cell cycle through the p21 or p53 pathway and induction of apoptosis [25]. Gurunathan et al. [64] reported that co-administration of melatonin and retinoic acid significantly increases the cytotoxicity and apoptosis in MCF-7 cells. The in vivo studies favor the melatonin-induced apoptotic rate in rat mammary tumors by increasing caspase-3 activity and DNA fragmentation [16, 54]. Treatment of 1 nmol/L melatonin showed a sharp reduction in mouse double minute 2 (MDM2) that promotes ubiquitin ligase-mediated breakdown of p53 [25]. Down-regulation of MDM2 enhanced acetylation of p53 and increases the expression of p21 levels, leading to inhibition of the cell cycle progression and induction of p53-mediated apoptosis [65].
Numerous in vitro and in vivo studies on breast cancer reported that melatonin is less sensitive to the apoptotic effects when administered as a single agent. Applications of melatonin as an adjuvant with chemotherapeutic drugs potentially magnify the apoptotic effects [49, 66]. The pharmacologic concentration of melatonin activates both intrinsic and extrinsic apoptotic pathways in cancer cells, specifically by increasing the p53/MDM2 ratio and down-regulating the Sirt1, which is a potent metabolic regulator [25, 67].
Effects of melatonin on metabolism in tumor cells
Tumor cells exhibit robust uptake of circulating glucose that converted to lactate through the classical glycolytic pathway (Warburg effect). This effect meets the energy demand in the cancer cells to accommodate fast-growing tumor biomass [68–70]. HIF-1α, Akt, and cellular-master regulator of cell cycle entry and proliferative metabolism (c-MYC) are the important signal transducing and transcriptional networks, which operate the Warburg effect to reprogram the metabolism in cancer cells for continuous proliferation [71, 72]. A high rate of glycolysis increases cell proliferation and survival properties in tumor biomass. Besides these, uptake of LA is the most critical step for breast tumor growth and cancer cell proliferation [73]. The study of the xenograft model had revealed that LA is metabolized to 13-hydroxyoctadecadienoic acid (13-HODE), which favors the metabolic alteration in breast cancer cells. Melatonin shows pleiotropic effects. Night-time melatonin maintains the proliferation and repairing of normal cells but diminishes the rate of tumor growth.
The LAN and circadian disruption promote the condition of a melatonin-deficient state. Moreover, LAN increases circulating IGF-1 levels and stimulates continuous expression of proliferating cell nuclear antigen (PCNA) in nude rats. LAN also induces Akt-dependent activation of phosphoinositide-dependent protein kinase 1 (PDK1), which facilitates the expression of the IGF-1R. Collectively, these effects accelerate tumor growth in tumor-bearing nude rats through the activation of IGF-1R/PDK1 signaling [74]. Therefore, chronic disruption of circadian equilibrium increases metabolic and proliferative activities. Moreover, melatonin regulates the circadian oscillations of tumor signaling factors such as Akt, cAMP, HIF-1α, LA metabolism, and the Warburg effect. dLEN crucially interrupted the circadian oscillations, leading to metabolic fluctuations and deregulation of allosteric feedback activity followed by impaired metabolism in human breast cancer cells [25, 34].
Effects of light exposure at night on melatonin secretion and breast cancer
Epidemiological studies indicate a higher risk for certain types of cancer in night shift workers. The International Agency for Research on Cancer recommended night shift work probably carcinogenic (group 2A) to humans [57, 75]. LAN has an influencing effect on breast cancer. The incidence of breast cancer shows 73% higher in LAN exposed individuals compared to the unexposed group [76]. This finding is supported by the relationship between urinary melatonin excretion and the occurrence of breast cancer. An earlier study had indicated the low levels of urinary melatonin in human breast cancer patients [77]. Devore et al. [78] had indicated the relationship between urinary melatonin and the risk of breast cancer in postmenopausal women. They had included 1,354 postmenopausal women (nurses) and measured 6-sulfatoxymelatonin (excretory product of melatonin) from the first-morning spot urine. They reported that excretion of urinary melatonin had been associated with a lower risk of breast cancer in postmenopausal women. Elevated serum estradiol and low levels of urinary 6-sulfatoxymelatonin had been observed in postmenopausal women working at night that might be the pre-indicator of risk of breast cancer. However, this effect was not modified by the expression of the MT1 receptor in tumor cells [79–81].
The decreasing function of the pineal gland may amplify the risk of breast cancer by increasing exposure to circulating estrogens. Calcification of pinealocytes increases the incidence of breast cancer. Women who are taking chlorpromazine showed elevated levels of melatonin and lower rates of breast cancer [82]. Human ovarian cells express melatonin receptors that have a direct effect on ovarian estrogen synthesis and restrict hormone-sensitive breast cancer formation [17]. Richard Stevens [83] made a prognostic hypothesis that exposure to LAN in women had higher rates of breast cancer.
Industrialization, electrification, exposure to LAN and night shift work in the USA and other Western countries have decreased the pineal melatonin production and increased the risk of breast cancer [25, 49]. Melatonin production in the pineal gland is an extremely trustable output signal of the circadian clock. This signal is depressed by the duration, intensity, and wavelength of LAN [49, 84]. Rotating night-shift work advances the light exposure-induced circadian disruption and increases the risk of breast cancer [85]. Blask et al. [49] reported that isolated breast cancer cells increased cAMP levels, the expression of mitogen-activated protein kinase (MAPK), uptake of LA and its conversion to 13-HODE when the cells were perfused with blood taken from women exposed to dim light (0.2 lux) at night. Reverse results appeared with the perfusion of blood taken from women at dark night. The inhibitory effect of melatonin is receptor-mediated, and the blocking of receptors shows antagonistic effects [74]. Mao et al. [86] reported that the phosphorylation of the GSK3β enzyme is a crucial factor for breast cancer cell metabolism, proliferation, and survival in the tumor xenograft model. LAN depresses nocturnal pineal functions, followed by inhibition of GSK3β activation through phosphorylation of serine-473 and serine-9 [30].
Impaired melatonin levels at night activate PI3K/Akt pathway, epidermal growth factor receptor (EGFR)/HER2 pathway, RAS/MAPK/ERK pathway, the PAK-1, PI3K/Akt/pyruvate dehydrogenase kinase-1 (PDK-1), and ribosomal S6-kinase (RSK). Collectively, these enzymatic pathways can promote cancer cell proliferation, survival, metastasis, and drug resistance [25, 87]. Other signaling pathways, including cAMP, PKA, PK-Cα and δ, c-Src, FAK, CREB, STAT3, and NF-κB were activated in dLEN-induced low melatonin state [88]. Supplementation of melatonin in isolated tumor exhibits inhibition of these signaling pathways to suppress tumor cell proliferation and drug resistance properties [49].
Peripheral tissues, including the breast, also bear circadian clocks that are synchronized with the SCN. The normal circadian rhythm regulates the expression of clock genes (PER, Cry, BMAL1, Clock). Impaired expression of clock genes influences cancer development [89]. The products of clock genes are involved in the expression of p21, Cyc D, c-Myc, and Wee1 for the regulation of the cell cycle [90–92]. The period 2 (PER2) has been reported to be a tumor suppressor gene whose expression reduces the formation of a variety of tumors like breast, prostate, and lymphoma [89, 93]. Loss of PER2 may induce breast cancer. Decreasing PER2 gene expression by methylation or degradation of PER2 by casein-kinase-1ɛ promotes the development of breast cancer. Circadian dysfunction alters DNA methylation and expression of clock genes, including PER2 [89, 94]. Audia and Campbell [94] reported that melatonin may regulate epigenetic-induced gene expression and resist the progression of the tumor. Several reports indicated that clock genes are involved in the regulation of the cell cycle, DNA repair, cellular metabolism, oxidative stress, apoptotic response, inflammatory activity, and epithelial-mesenchymal transition. Circadian dysfunction and impaired melatonin alter the expression of clock genes, leading to the progression of cancer [46, 95, 96].
Role of melatonin in therapeutic strategies of breast cancer
Resistance to chemotherapy and endocrine therapy is the most important obstacle for the successful treatment of breast cancer [97, 98]. Experimental evidence showed the link between activation of different types of pro-oncogenic tyrosine kinases and the development of resistance power against chemotherapeutic drugs as well as anti-estrogenic agents in breast cancer cells [99, 100]. About 60–75% of breast cancer cells express progesterone receptor (PR) and ER-α that are used as a marker for the endocrine therapies, including ER-α modulators, such as tamoxifen [101, 102]. However, 40% of patients with ER-α-positive breast tumors show inherent resistance to tamoxifen [103]. Anthracycline, such as doxorubicin, is commonly used as a chemotherapeutic drug for patients whose breast tumors are in metastatic stage or endocrine-resistant [104]. There are various types of mechanisms that make the cancerous cell to resist drugs or chemotherapies. The activation of numerous signaling pathways, including EGFR/HER2/MAPK/ERK and PI3K/Akt enhances drug resistance capacity [105, 106]. It was reported that the expression of various transcription factors and kinases are elevated in human breast cancer cell lines and in experimental breast tumor biopsy samples. These observations indicate the inherent capacity of resistance to anti-endocrine and chemotherapeutic agents [107]. The drug resistance properties are linked with the up-regulation of adenosine triphosphate (ATP)-binding cassette (ABC) transporter such as ABCB1, ABCC1, and ABCG2 as well as drug-metabolizing enzymes; the results are decreased levels of active drug within the cancer cells [108]. It had found that exposure to dLEN enhances the expression of the ABC transporter-like ABCG2 that is termed as the breast cancer resistance protein. Breast cancer resistance protein involves the efflux of 4-hydroxy-tamoxifen (4OH-Tam), and endoxifen [25]. Several in vitro studies proved that melatonin may increase the efficacy of tamoxifen and doxorubicin [54, 64]. Melatonin alters the activity of ER-α through inhibition of kinase signaling pathways and increases the tamoxifen-mediated antagonistic effects on ER-α. It also reduces the efflux of tamoxifen from breast tumor cells [54]. The tumor xenografts were extremely sensitive to the synergistic actions of melatonin and doxorubicin. Supplementation of melatonin suppresses the growth of the doxorubicin-resistance cancer cells [25, 109].
Application of melatonin in the clinical trials for breast cancer treatment
Melatonin has potential anti-cancer properties. But the results of clinical trials in human breast cancer therapy are insufficient, and it is not included in the treatment regimen [110]. However, some reports are available in the field of the clinical trial of melatonin. The studies indicated that the cancer patients taking 20 mg of melatonin gave better clinical outcomes. The beneficial effects were remission of tumor volume and decreased rate of mortality [111–113]. It was observed that sleep problem is a great challenge in breast cancer survivors, even after the completion of anti-cancer therapy. Li et al. [114] reported that the first cycle of breast cancer chemotherapy disrupted the sleep-wake cycle, sleep quality, cognitive functions, and melatonin secretion. These are appeared as the side effects of breast cancer treatment. Application of 20 mg melatonin as an adjuvant prior to first cycle chemotherapy of breast cancer in a randomized, double-blinded, placebo-controlled trial with 36 participants showed improvement of cognitive performance, immediate and delayed episodic memory, sleep quality, and depressive symptoms [115]. Previously, a randomized, double-blind, and placebo-controlled trial of melatonin had also been conducted on the patients undergoing breast cancer surgery. The application of 6 mg oral melatonin for 3 months significantly reduced the risk of depressive symptoms [116].
Estradiol levels, IGF-1 concentration, insulin-like growth factor-binding protein 3 (IGFBP-3) expression, and the IGF-1/IGFBP-3 ratio are regarded as the biomarkers of breast cancer. Schernhammer et al. [117] had evaluated whether melatonin has an effect on biomarkers or not. They performed a randomized, double-blind, and placebo-controlled study on 95 postmenopausal women who had a prior history of breast cancer report in different sage (0-III). These patients had completed the anti-cancer treatment (including hormonal therapy). A course of 3 mg of melatonin per day for 4 months had been given orally to the 48 patients and a placebo had been provided to the rest of the members (47 patients). The outcome of the study had revealed that melatonin did not influence circulating estradiol, IGF-1, IGFBP-3 levels, and IGF-1/insulin-like growth factor-binding protein (IGBP) ratio after the short-term course of melatonin treatment. Chen et al. [118] had conducted the same study as performed by Schernhammer et al. [117] to establish the role of melatonin on the improvement of sleep quality. Finally, they reported that melatonin-experienced patients showed significant improvement in sleep quality and related parameters. A good result had been observed in Pittsburgh sleep quality index (PSQI) score compared to placebo-controlled patients.
A clinical study had been conducted on 167 patients with TNBC. The outcome of the study had indicated that 48% of the TNBC patients showed MT1-negative while only 11% TNBC exhibited MT1-positive. The MT1-positive TNBC patients were in the early stage of breast cancer with a smaller volume of tumor size. The MT1-negative TNBC patients were at high risk of cancer progression. The progression-free survival (PFS) rate was also low. The overall survival ratio compared to PFS was significantly decreased in MT1-negative TNBC [38]. Simultaneous use of somatostatin, melatonin, retinoid, vitamin D3, and cyclophosphamide (prolactin inhibitor) has been made on 20 women who have breast cancer. The rate of response of the treatment is about 75% (55% complete response and 20% partial response) while 71% of metastatic patients exhibit overall survival capacity [119].
Conclusion
Melatonin acts as a potent anti-cancer agent. The actions of melatonin in breast cancer are driven by regulating the different molecular pathways related to growth inhibition and pro-apoptotic effects. Exposure to LAN, shift/might work, chronic jet lag, and sleep disorder hamper melatonin synthesis and lower the night-time melatonin peak. Epidemiological studies regarding breast cancer indicated that women working at night or swing shifts were vulnerable to the development of breast cancer. The prospective studies indicated the association between a low level of urinary melatonin and the incidence of breast cancer. Melatonin exerts anti-estrogenic and anti-inflammatory, immune-modulating, and anti-mitogenic actions. Supplemental melatonin shows oncostatic effects in breast cancer. MT1 receptors are the key factor for cytostatic and pro-apoptotic activity. Several possibilities regarding melatonin’s actions as an anti-cancer agent in breast treatment are showing positive impacts and have a prospective future as a therapeutic agent in breast cancer treatment. Melatonin increases the efficacy of chemotherapeutic drugs in the treatment of breast cancer. On the other hand, supplementation of melatonin at nontoxic pharmacological doses in breast cancer patients gives financial benefit due to its low cost and wide accessibility. Finally, it can be said that further research and prospective clinical trials will open a new avenue for the use of melatonin in the treatment of breast cancer.
Abbreviations
ABC: | adenosine triphosphate-binding cassette |
Akt: | protein kinase B |
Bax: | B-cell lymphoma-2 associated X protein |
Bcl: | B-cell lymphoma |
CaM: | calmodulin |
cAMP: | cyclic adenosine monophosphate |
CREB: | cyclic adenosine monophosphate-responsive element-binding protein |
Cyc D1: | cyclin D1 |
dLEN: | dim light exposure at night |
ER-α: | estrogen receptor-α |
ERK: | extracellular receptor kinase |
G-protein: | guanosine triphosphate-binding protein |
GSK3: | glycogen synthase kinase 3 |
HER2: | human epidermal growth factor receptor 2 |
HIF-1α: | hypoxia-inducible factor 1α |
IGF-1R: | insulin like growth factor 1 receptor |
IGFBP-3: | insulin-like growth factor-binding protein 3 |
LA: | linoleic acid |
LAN: | light-at-night |
MAPK: | mitogen-activated protein kinase |
MDM2: | mouse double minute 2 |
MT1: | melatonin receptor 1 |
NRs: | nuclear receptors |
PER2: | period 2 |
PI3K: | phosphoinositide 3-kinase |
PKA: | protein kinase A |
ROR: | retinoid-related orphan receptor |
Src: | sarcoma |
TGF: | transforming growth factor |
TNBC: | triple-negative breast cancers |
Declarations
Acknowledgments
The authors are grateful to Midnapore College, Midnapore, West Bengal, India, for providing all kinds of facilities (infrastructural, computer and internet) to prepare this manuscript.
Author contributions
Dr. NKD started literature survey and collected the relevant papers. He had prepared the draft of the manuscript. Dr. SS had supervised the work. He had involved in the writing and correction of the manuscript. At the finishing stage, he had drawn all the pictures and given the final shape of the manuscript.
Conflicts of interest
The authors declare that they are no conflicts of interest.
Ethical approval
Not applicable.
Consent to participate
Not applicable.
Consent to publication
Not applicable.
Availability of data and materials
Not applicable.
Funding
Not applicable.
Copyright
© The Author(s) 2022.