Abstract
The ubiquity of circadian rhythms in living organisms has generally been accepted by researchers over the last century. Indeed, morphology and molecular biology of the circadian clock were described during the last fifty years. This main biological clock is located in the suprachiasmatic nucleus of the hypothalamus. This nucleus is connected with the retina by the retinohypothalamic tract. This way, light regulates the functioning of the biological clock and biological rhythms such as the sleep-wake cycle and other cyclic functions by releasing melatonin from the pineal body (PB) into the general circulation. Melatonin reaches the retina via the bloodstream as humoral feedback. More than a hundred years ago a reverse neuronal connection between the central nervous system and the retina was hypothesized. This so-called centrifugal visual or retinopetal system has been explored in detail in birds, but less information is available in mammals. In this work, the morphology and physiology of mammalian centrifugal visual pathways are reviewed. It is generally accepted that the centrifugal (retinopetal) fibers terminate mainly on the amacrine cells of the retina. Histaminergic fibers terminate on dopaminergic amacrine cells. Serotoninergic synapses were identified on ganglion cells. In addition, serotoninergic fibers were also associated with photoreceptor terminals. Luteinizing hormone releasing hormone fibers have been observed in birds, but not in mammalian retinas. In summary, based on the data available in the literature, it seems that the retinopetal system has a mandatory role in lower vertebrates, but a modulatory role in mammals. There is currently no adequate way to eliminate the centrifugal visual system that would better explain its true function.
Keywords
Rat, hamster, pineal body, virus labeling, electron microscopy, immunostaining, double labelingIntroduction
The main visual pathway of most species is formed by the axons of ganglion cells, located in the ganglion cell layer (GCL) of the retina, which transmit image-forming information to the brain. In addition to this, non-image-forming photosensitive ganglion cells, located in the inner nuclear layer (INL) and GCL, are involved in the formation of the retinohypothalamic tract (RHT). This pathway transmits light information to the circadian pacemaker, i.e., to the suprachiasmatic nucleus (SCN), which is the main retinorecipient area of the hypothalamus. It is commonly referred to as the “biological clock” or the “mind’s clock” [1, 2]. This nucleus regulates the rhythmic functioning of the organs through a neuronal network expressing clock genes. The RHT also projects to the anterior hypothalamic, retrochiasmatic, and lateral hypothalamic areas. The anterior hypothalamic projections extend into the subparaventricular and perifornical regions, the dorsal hypothalamus, and zona incerta [3]. Photosensitive ganglion cells also send axons directly to the intergeniculate leaflet and olivary pretectal nucleus, where they contribute to the pupillary light reflex [4].
The internal circadian rhythm is a little longer or shorter than 24 h, and it is adjusted to the local environment by external cues originally named “Zeitgeber” (time giver) [5, 6]. Light is the most powerful synchronizing factor that sets the 24 h rhythm. The behavior and physiological functions of mammalian organisms are dictated by the rotation of Earth. The biological clock regulates the sleep-wake cycle by releasing melatonin from the pineal body (PB). In turn, the sleep-wake cycle affects eating habits, digestion, body temperature, hormone release, and behavior [7–10].
In the absence of the SCN, an endogenous “local clock” has been demonstrated in a variety of cell types (liver, pancreas, adrenal, muscle, heart, and adipose tissue [11] and in the photoreceptors of the retina [12]). Tosini and co-workers [12] concluded that the “local clocks are primarily responsible for integrating different timing signals to produce an appropriate response to external stimuli”. Melatonin supplements can also shift the timing of the body’s clock. Melatonin can be used as a sleep aid because it has a mild sleep-promoting effect.
The main neurotransmitter of the RHT is glutamate, but this pathway also contains pituitary adenylate cyclase activating polypeptide (PACAP) and melanopsin (MPS) [13]. Tsuji and co-workers [14] have shown that vasopressin (VP) is also present in some retinal ganglion cells which are found in both the GCL and INL. They have also shown that VP containing cells also use glutamate as a neurotramsmitter, and that 25% of large VP-glutamate immunoreactive cells were also immunoreactive for MPS, but it is still unknown whether all or only one subtype of these large cells participates in the formation of the RHT projecting to the SCN.
From the SCN, a multisynaptic pathway, composed of the hypothalamic paraventricular nucleus (PVN), the intermediolateral nucleus in the spinal cord (IML) and the superior cervical ganglion (SCG), reaches the PB. The final neurons of this chain, the sympathetic neurons in the SCG, send fibers to the PB and release noradrenalin to the pinealocytes, which in turn release melatonin into the general circulation [15]. The genetic elimination of displaced ganglion cells via expression of diphtheria toxin under the MPS promoter led to the complete loss of circadian entrainment and a severely impaired pupil light response [16–18].
Role of melatonin produced in the PB
In daylight the SCN is activated by light impulses, and the SCN inhibits the transmission of impulses to the PB through a gamma aminobutiric acid (GABA)-ergic mechanism located in the PVN. Thus, melatonin synthesis decreases, resulting in a significant decrease in the blood melatonin level. Melatonin is the chemical signal of darkness. Kalsbeek and co-workers [19] said “melatonin sees the light” and, as a consequence of this ability, melatonin level declines in the daytime. The human circadian system is sensitive to short wavelength light. Glutamate and similar agonists such as N-methyl-D-aspartate and PACAP mimic light-induced phase shifts. Possible targets for glutamate and PACAP are the “clock” genes Period1 (Per1) and Per2 [13]. Melatonin, as a humoral feed-back, affects the circadian clock.
In mammals, melatonin has two G protein coupled receptors, melatonin receptor 1 (MT1) and MT2, whereas the third, MT3 is an enzyme, quinone reductase 2 (NQO2). Melatonin also has nuclear receptors [retinoid-related orphan nuclear receptor family (ROR/RZR; RZRα/ROR, RZRβ/ROR)] [20–22]. Crystallographic evidence suggests that melatonin and its metabolites are not high-affinity ligands for ROR. Therefore, it is debated whether ROR is a nuclear receptor of melatonin [23]. The roles of both MT1 and MT2 are well established and both are identified in the SCN. MT2 activation has been shown to mediate the phase advances of the circadian rhythm [24].
Role of melatonin produced in the eye
In the last few years, it has become apparent that melatonin is also produced in the eye, and receptors for melatonin exist in the retina. The main sources of ocular melatonin are the photoreceptors [25], but non-pigmented ciliary and crystalline lens epithelia also produce melatonin [26, 27]. 5-Hydroxytryptophan is decarboxylated to serotonin, which is converted into melatonin by a chemical process catalyzed by 2 enzymes, arylalkylamine N-acetyltransferase (AANAT) and hydroxyindole-O-methyltransferase (HIOMT). The presence of both AANAT and HIOMT has been demonstrated in the retina, and these results strongly suggest that melatonin is not only present but it is produced in the retina [28–31]. Besides the enzymes, which are involved in the synthesis of melatonin, MT1 and MT2 receptor transcripts, studied by reverse transcription-polymerase chain reaction (RT-PCR), were demonstrated in human postmortem retina. The MT1 receptor was also identified by immunohistochemistry [32]. MT1 receptor protein was found on CA1 dopaminergic amacrine cells [positive for anti-tyrosine hydroxylase (TH) immunolabeling] along the inner border of the INL and inner plexiform layer (IPL) and GCL layers. The MT1 receptor was also present on horizontal cells, which were identified by colabeling with parvalbumin. Double labeling with either calbindin, a cone photoreceptor marker, or opsin, a rod cell marker, indicated that MT1 is also expressed on the inner segment of cones and rods [32].
The question arises as to the role of local melatonin in the retina. Because the available experiments are carried out using exogenous melatonin administration in cell or organ cultures, it is difficult to separate the effect of endogenous or exogeneous melatonin. The majority of researchers agree that melatonin maintains the integrity of the epithelium of choriocapillary vessels through its antiapoptotic and antioxidant effects. Melatonin deactivates the injured microglia, which would otherwise break down the barrier, and in this way, melatonin maintains the integrity of the blood-retina barrier [33]. In the retina, melatonin diffuses from the site of production to bind to specific receptors on a variety of inner retina neurons to modify their activity. In mice, immunocytochemistry using the anti-MT1 antibody revealed a strong and specific immunoreactivity in the photoreceptors, and a weak immunoreactivity was also observed in the outer plexiform layer (OPL). The immunoreactivity was completely abolished by pre-incubation of the antibody with the blocking peptide [34].
Most researchers accept that the potential target cells of melatonin in the inner retina are photoreceptors, amacrine cells, bipolar cells, horizontal cells, and ganglion cells. MT subtypes show differential, cell-specific patterns of expression that are likely to underlie differential functional modulation of specific retinal pathways. Melatonin inhibits the release of dopamine from amacrine cells and increases the sensitivity of horizontal cells. Melatonin potentiates rod signals to ON-type bipolar cells, via activation of the melatonin MT2 receptor, suggesting that melatonin, based on the differential distribution of its receptors, modulates the function of specific retinal circuits. The cell-specific expression of the MT is the essential factor that determines the influence of melatonin on retinal function. One of the potentially important roles of melatonin in the normal retina is the modulation of the daily renewal of the photoreceptor outer segment membrane. Horizontal cell axons expressing MT1 receptors form synaptic contacts with cone terminals that synapse with MT2 receptor-positive OFF-cone bipolar cell dendrites [35].
In the last few decades, more and more research groups have confirmed the beneficial effect of exogenous melatonin on inflammatory diseases of the eye [32–34]. In guinea pigs, uveitis was induced by intravitreal administration of bovine serum albumin and 3 days later melatonin, vitamin E, or aprotinin were administered intraperitoneally. It was experienced that the histopathological alterations and edema were decreased [36]. Other researchers injected lipopolysaccharide (LPS) intravitreally in golden hamsters to induce uveitis. Two hours before LPS injection, a melatonin pellet was implanted subcutaneously. Clinical and histopathological parameters of uveitis showed improvement [37, 38]. It is also worth mentioning that most researchers emphasize that melatonin exerts an immunomodulatory effect via immunocompetent cells. Indeed, melatonin supplementation can be used to treat oxidative stress and inflammation-related disorders (obesity, cardiovascular diseases, immune disorders, infectious diseases, cancer, neurodegenerative diseases, osteoporosis, and infertility) [39, 40].
Atacak and co-workers [41] induced diabetes with intraperitoneal streptozotocin in aged female rats. The retinal damage was assessed by measuring glutathione (GSH) and malondialdehyde (MDA) levels by enzyme linked immunosorbent assay (ELISA), as well as sirtuin1 (SIRT1, a neuroprotective molecule) messenger RNA (mRNA) by PCR in retinal tissue. GSH and MDA are biomarkers of oxidative stress. SIRT1 is a nicotinamide adenine dinucleotide (NAD+)-dependent protein deacetylase that governs many physiological pathways. Intravitreal melatonin supplementation increased the activation of antioxidant molecules and the expression of SIRT1. These molecules have antiapoptotic and retinoprotective effects. This experiment described the mechanism by which melatonin exerts beneficial effects in the treatment of diabetic retinopathy. In the following experiments the authors studied the beneficial effect of long-term oral administration (90 days) of melatonin on the retina in glaucoma patients. It was found that melatonin alleviated the disturbance of the circadian rhythms caused by glaucoma with different effects of melatonin on systemic vs. local circadian rhythms [42].
Centrifugal visual system
It is generally accepted that there are reverse neuronal connections to the retina from the central nervous system, called the centrifugal visual system [43–45]. Centrifugal visual fibers were first observed 130 years ago by Ramon y Cajal [46]. Based on the silver impregnation technique, he assumed that the fibers coming from the central nervous system terminate on the amacrine cells. After the introduction of modern tract tracing methods, several structures were identified as the origin of centrifugal visual fibers, and non-transsynaptic tracers have been used to identify the origin of these fibers in mammals. Tracers injected into the vitreous body can be taken up by retinal neurons. This phenomenon was utilized in the following experiments. After injection of horseradish peroxidase (HRP) into the vitreous body of rats, retrogradely labelled neuronal perikarya appeared in the pretectal area, periaqueductal gray matter [47], caudal mesencephalon, oculomotor nucleus [48] and in the ventral hypothalamus of dogs [49]. With the use of fast blue, Bons and Petter [50] found labelled neurons in the arcuate nucleus of monkeys. Villar and co-workers [51], using HRP as a tracer, observed labelled neurons in the serotoninergic dorsal raphe nucleus of rats, and Labandeira-Garcia and co-workers [52] confirmed these data in monkeys, cats and guinea pigs. In rats and rabbits, they also observed labeling in the tegmentum and trochlear nucleus. In 1999, Gastinger and co-workers [53] described a dense pattern of histamine-immunoreactive fiber labeling in the retina; however, cell body labeling was not observed, suggesting that these fibers may originate from the brain where histaminergic neurons reside, i.e., from the tuberomammilary nucleus of the posterior hypothalamus. In weaver mice, Simon and co-workers [54] described retinopetal TH-immunoreactive fibers in the optic nerve and retina. The dopaminergic fibers appeared first in the optic nerve (postnatal day 12) and a day later in the retina as well.
Ample evidence indicates that the centrifugal visual fibers terminate on amacrine cells as hypothesized by Ramon y Cajal [46]. In the retina amacrine cells form synaptic connections with other retinal cells. Histamine receptor (HR) mRNA (HR1, HR2, HR3) was identified in the mouse retina by RT-PCR. HR1 immunoreactivity was localized on dopaminergic amacrine cells and calretinin-positive ganglion cells, indicating that histaminergic fiber (HIST) terminate on these cells. HR2 immunoreactivity was observed on the processes and cell bodies of the primary glial cells (Müller cells) of the mouse retina. It was also demonstrated that amacrine cells form synaptic connections with displaced ganglion cells, but there was no evidence of a direct connection between the retinopetal fibers and displaced ganglion cells located in the INL [55]. With the use of electron microscopy, Lindstrom and co-workers [56] identified axon terminals on amacrine cell somata and dendrites in the avian retina (leghorn chicken). These fibers, labelled by anterograde tracer, originated from the isthmo-optic nucleus.
Vesselkin and co-workers [57] filled both retinofugal and retinopetal fibers with HRP through either the cut optic nerve in isolated lamprey retina preparations or after intracerebral HRP injections. The electron microscopic analysis revealed that the centrifugal terminals synapse either upon unlabelled somata of profiles containing synaptic vesicles. In many cases, these boutons appear to establish synaptic contacts on ganglion cell dendrites. The target cell bodies were located within the inner part of the INL. This localization strongly supports the view that the ganglion cells were a displaced type, at least in the lamprey. The morphological and physiological evidence indicate that postsynaptic potential generating cells are activated synaptically by centrifugal fibers and that in the lamprey retina these fibers make contacts either with dendrites or somata of amacrine cells and with ganglion cell dendrites.
It is interesting that the direct connection between the retinopetal fibers and the displaced ganglion cells are not discussed in the literature, even in a very recent paper by Raja and co-workers in 2023 [58] and by Kolb in 2011 [59]. In mammals, direct electron microscopic evidence is not available.
Limbo-retinal and hypothalamo-retinal system
Using biotinylated dextran amine (BDA, 10,000 MW), a tracer that cannot pass through gap junctions or synapses, and is not endocytosed from the blood was injected into the vitreous body of adult Sprague-Dawley male rat eyes in vivo [44]. The tracer was taken up by the retina and transported both antero- and retrogradely. Dense parallel bands of labelled fibers were observed in the optic nerve and optic tract. In the SCN and the lateral geniculate body, where the retinofugal fibers (RF) terminate, a densely labelled fiber-network was observed. In addition to the known RF (main visual and retinohypothalamic pathways), labelled nerve cell bodies can also be seen in several limbic structures such as the dentate gyrus, CA1 and CA3 regions of the hippocampus, the indusium griseum, habenula and olfactory tubercle. Labelled cells were also observed in the PVN and supraoptic nucleus (SON). Some labelled cells in the dentate gyrus also showed vasoactive intestinal polypeptide (VIP), PACAP or luteinizing hormone releasing hormone (LHRH) immunoreactivity. There was colocalization between BDA and LHRH in the olfactory tubercle and indusium griseum, and colocalization between BDA and PACAP in the SON and habenula. When another tract tracer, fluorogold, was administered iontophoretically into the dentate gyrus, where the majority of labelled neuronal cell bodies were found, labelled fibers appeared in the optic nerve at both sides. The labelled fibers entered the retina through the optic nerve layer and reached the INL. Here the fibers formed loops around unlabeled cells. Labelled cell bodies were never observed in the retina, indicating that the labelled fibers originate from the structures mentioned above. It was accepted that these fibers are retinopetal, and we named this system the limbo-retinal and hypothalamo-retinal pathways. Such a hypothalamo-retinal connection was also described in lower vertebrates by Repérant and co-workers [60].
In another experiment, using double virus labeling and oxytocin immunostaining in rats, it was found that the same magnocellular neurons send axon collaterals to both the posterior pituitary and the retina [61]. A retrograde-specific virus (Ka-gEI-memGFP-RV) that expressed green fluorescent protein (GFP), was injected into the vitreous body of the eye. A second, non-direction-selective virus (Ka-Vhs-CHR-memTomato-A-RV), which expressed a red fluorescent protein (Tomato), was injected into the posterior pituitary at the same time. The latter virus was only retrogradely transported from the posterior pituitary, as cell bodies are not present in the posterior pituitary. A few double labelled neurons were observed in the SON and the magnocellular part of the PVN. Some double virus labelled cells also showed oxytocin immunoreactivity. These cells were also seen in sympathectomized rats. Experiments where the optic nerve had been previously transected showed nolabeling in the SON. It was concluded that the same magnocellular oxytocinergic neurons send axon collaterals to the posterior pituitary and also to the retina. This experiment supports our previous observation that there is a hypothalamo-retinal system.
Pinealo-to-retinal connection
The centrifugal visual pathways discussed above consist of direct neuronal connections. However, we found another retinopetal system connecting the PB and the retina [62]. In non-mammalian vertebrates, both photoreceptors and neurons exist in the PB. During evolution the PB lost its photosensory role, but neurons in the PB continue to exist in various mammalian species. These neurons are relatively difficult to locate, requiring very meticulous work—the number of these cells is relatively low, and the whole organ should be sectioned and each slide examined.
Twenty-nine years ago, Ekström and his co-workers [63] discussed that in lower vertebrates the pineal neurons are typically postsynaptic to photoreceptors, and their axons form the pineal tract that primarily innervates forebrain cell groups in the pretectum and thalamus, while other neurons project to the habenula, hypothalamus and preoptic region. Some of these targets of the pineal tract also receive direct retinal innervation. The above-mentioned system is reduced during phylogenesis, but further survives in mammals.
Ichimura and co-workers [64] demonstrated the presence of such neurons in monkey (Macaca fuscata) PB using electron microscopy. Approximately 70 nerve cell bodies were detected in the central part of one half of the total PB. Vígh-Teichman and co-workers [65] found neurons in cat PB using postembedding GABA immunocytochemistry and electron microscopy. The positive neurons were located in the proximal part of the PB, and positive fibers were found near the habenular commissure. The pinealocytic axons formed ribbon-containing synapses on the dendrites of GABA-negative primary neurons and synaptic contacts were found between negative and positive neurons. Positive neurons were located near the posterior and habenular commissures, and their axons were found to join the commissural fibers; they may represent a pinealofugal pathway collecting information from the pinealocytes. In cotton rat, substance-P (SP) immunoreactive nerve cells were found in the PB [66].
With the use of transneural tract tracing methods, we also found neurons in the PB of adult golden hamsters [62]. A retrograde transporting virus expressing GFP (Ka-gEI-memGFP-RV) was injected into the vitreous body of the eye. The virus was taken up by the retina and it was transported to the brain. Four days later the animals were sacrificed and frozen sections were made using a cryotome. The whole organ was sectioned and nerve cells were counted. The total number of neurons ranged from 5 to 36 per animal. However, in adult rats using a similar approach only 1–2 cells were found in the whole organ. In our next experiment [67] we used Sprague-Dawley prepubertal albino rats of both sexes at different ages. A similar retrograde virus (Ka-gEI-memGFP-RV) was injected into the vitreous body of the eye. The virus labeling revealed that the number of labelled neurons is very high in neonatal rats (250–300/animal) in both sexes, but there was a tremendous decrease between the 6th and 8th postnatal days. After that, the number of neurons slowly decreased and practically disappeared by the time of puberty, 5–6 days later in males compared to females. With the use of electron microscopy, we confirmed that the labelled cells are neurons. Pinealocytes were not infected by the virus, always appearing negative [68]. Injection of an anterograde spreading virus (Ka-Vhs-CHR-memTomato-A-RV) in the PB of adult golden hamsters or six-day-old Sprague-Dawley rats induced labeling in the retina. Ganglion cells, bipolar cells, rods and cones were also labelled [62, 67]. It is interesting to note that photoreceptors can transmit the virus, but that the pinealocytes have lost this ability [68].
Characterization of neurons in six-day-old rats by immunohistochemistry revealed that the pineal neurons synthesize neuropeptides and neurotransmitters [69]. We have tested 16 various antibodies such as dopamine β-hydroxylase (DBH), neuropeptide-Y (NPY), vesicular acethylcholine transporter (VAChT), vesicular glutamate transporter 1 (VGluT1), calcinonin gene related peptide (CGRP), SP, oxytocin (OT), VP, leu-enkephalin (L-ENK), glutamic acid decarboxylase (GAD, indicates the presence of GABA), neuronal nuclear antigen (NeuN), calbindin, LHRH, somatostatin, nesfatin (NES) or orexin (ORX). We found that SP, OT, VP, L-ENK and GAD immunoreactive neurons were located in the PB and these cells showed colocalization with a neuron marker, NeuN.
How does the centrifugal visual system influence the retinal functions during the circadian cycle?
Morphological considerations
Centrifugal visual fibers establish synaptic connections mainly with amacrine and ganglion cells. The number of these fibers is limited; however, they form a rich arborization in the retina, suggesting a central control of retinal functions [46, 70, 71]. Electron microscopic investigation confirmed synaptic connections between amacrine cells and centrifugal visual fibers in non-mammalian vertebrates [72].
Gastinger and co-workers [73] reviewed the morphological and physiological data concerning the retinopetal system. The first important information is what neurotransmitters and neuropeptides are present in the retinopetal fibers. (1) HIST derive from the tuberomammillary region of the posterior hypothalamus, the only place in the brain where histaminergic neurons reside. HIST establish a synaptic contact with dopaminergic amacrine neurons, but histaminergic receptors were also localized to ON bipolar cell dendrites. This suggests that HIST may terminate on these cells as well. (2) The serotoninergic fibers (SER) are derived from the midbrain dorsal raphe nucleus. Overall, the number of neurons projecting to the retina is relatively limited (5–300) and varies from species to species. These fibers terminate on ganglion cells and associate with choriocapillary vessels. A very limited amount of serotonin is also synthesized locally by photoreceptors as a precursor of melatonin, and some amacrine cells also use serotonin as a transmitter. (3) LHRH was shown in rats, voles and monkeys in the optic nerve, but not in their retina. However, LHRH fibers and additionally LHRH cell bodies were also detected in birds [74]. As also mentioned above, after BDA was injected into the vitreous body, retrogradely labelled neurons were identified in the indusium griseum, hippocampus, dentate gyrus, habenula, olfactory bulb, SON, and PVN. A colocalization study revealed that VIP, PACAP, and LHRH may also be present in BDA labelled neurons. These results suggest that VIP, PACAP, LHRH fibers originating from the central nervous system may be present in the retina as well. Both PACAP (ganglion cell) and VIP (amacrine cell) immunoreactive neurons are also locally present in the retina. Currently, there is no direct evidence to indicate the origin of the PACAP or VIP fibers detected in the retina, a question that requires further experiments.
Functional considerations
About 50 years ago, Marchiafava [75] carried out electrophysiological experiments on turtle retina. This species was selected because the amacrine cells are large and intracellular current can be easily recorded. The ganglion cells responded to optic nerve stimulation with antidromic action potentials, and the amacrine cells generated excitatory postsynaptic potentials. According to Marchiafava [75] this finding clearly indicates that “the excitatory postsynaptic potential is due to a single afferent fiber terminating in the proximity of the cell soma. This fiber traveled through the optic nerve to the retina from the central nervous system”.
Histaminergic neurons are called “waking up” neurons located in the tuberomammillary region. The histamine release in the retina varies depending on the sleep/wake cycle of the animals. It is high during daytime and low at night in diurnal animals, and is reversed in nocturnal species. Histamine induces blood vessel relaxation, increases the blood flow of the optic nerve in daytime and enhances the permeability of the blood-retinal barrier. In summary, “histamine optimizes retinal function and contributes to light adaptation” [53].
SER act on ganglion cells; however, serotonin receptors are also localized to photoreceptor terminals. Serotoninergic retinopetal axons, similarly to histaminergic ones, release more serotonin during the day and less at the night in diurnal animals, and the reverse is true for nocturnal species. Based on the results of various animal experiments it seems that serotonin “improves the performance of neural circuits in the retina under the given conditions when the animals wake up (scotopic vision). Acting via 5-hydroxytriptamine receptors (5-HT1), serotonin is expected to increase the absolute light sensitivity of rabbit retinal ganglion cells by decreasing surround inhibition [73]”. In summary, the serotoninergic centrifugal visual fibers optimize the function of the retina at the given ambient light intensity during the waking period of the animal. In some species, dopaminergic amacrine cells are also targets of retinopetal fibers, and because dopamine influences so many types of retinal neurons, this morphological situation multiplies the effects of the retinopetal axons. Retinopetal axons play a role in dark adaptation in these species. In nocturnal animals, the retinopetal fibers inhibit dopamine release from amacrine cells, and this decreased nocturnal dopamine release may facilitate dark adaptation [73].
The presence of LHRH in the retina of adult mammals has not been demonstrated. The role of LHRH in the retina was only studied in non-mammalian species, mainly in birds. Amacrine and displaced ganglion cells and fibers were demonstrated. It has been thought that LHRH fibers may influence retinal cell responses to hormonal cycles [74].
Wilson and Lindstrom [76] proposed that the centrifugal visual system in birds acts “as an early warning sign that allows the presence of a moving shadow to trigger a very rapid and parallel search of the regions of sky most likely to contain an aerial predator. Once an association between shadow and object is established, the system locks on through positive feedback and continues to track shadow and object together. Several previously suggested ideas are incorporated into this new view of function, for example, the idea of highlighting, predator detection, and static scanning of the sky” [76].
In mammals, RF terminate in limbic structures, the thalamus, and the bed nucleus of stria terminalis [76]. We have shown that the limbic structures, on the other hand, send fibers to the retina. This means that there is a retino-limbic-retinal circuit [60]. It has been hypothesized that light influences behavior, mood, and hormonal conditions and, as a feedback mechanism, the limbic structures affect the visual signal transmission.
There is considerable evidence that retinopetal fibers can influence eye development in chicks [77], and intensive work is underway to explore the role of retinopetal fibers in mammals [78].
Both the retino-pineal and the pinealo-to-retinal pathway differ from other identified connections in that they are multisynaptic systems [15, 62]. It is well established that the light information, transmitted through the SCN, regulates the function of the pinealocytes. In diurnal species, pinealocytes release melatonin at night and the melatonin release is inhibited by light during the day. The function of pineal neurons is not known at this time. We assume that this neuronal system plays a role in the onset of puberty, as was observed a long time ago. This theory is plausible because the number of pineal neurons in peripubertal rats is dramatically reduced, and by the time of puberty the neurons have disappeared. We have also shown that this process takes place by apoptosis [67]. It is also well known that continuous illumination interrupts the ovarian cycle. It would be interesting to know whether continuous illumination in peripubertal rats prevents the disappearance of the pineal neurons.
In summary, based on the data available in the literature, it seems that the retinopetal system has a mandatory role in lower vertebrates, but a modulatory role in mammals. There is currently no adequate way to eliminate the centrifugal visual system that would better explain its true function. Interruption of the entire centrifugal visual system is impossible because it is not a single homogeneous unit, but the cells of origin are located in different places.
Conclusions
The internal neuronal circuit of the retina integrates external light impulses and information arriving via the centrifugal visual (retinopetal) fibers from various brain structures. MPS immunoreactive glutamatergic-PACAP-ergic and MPS immunoreactive VP-ergic ganglion cells further transmit the processed impulses to the biological clock (and other retinorecipient areas) which drive the circadian rhythms. There is no direct evidence for the termination of retinopetal PACAP, VIP, and LHRH fibers in mammals. We have summarized the aforementioned hypothesis in Figure 1.
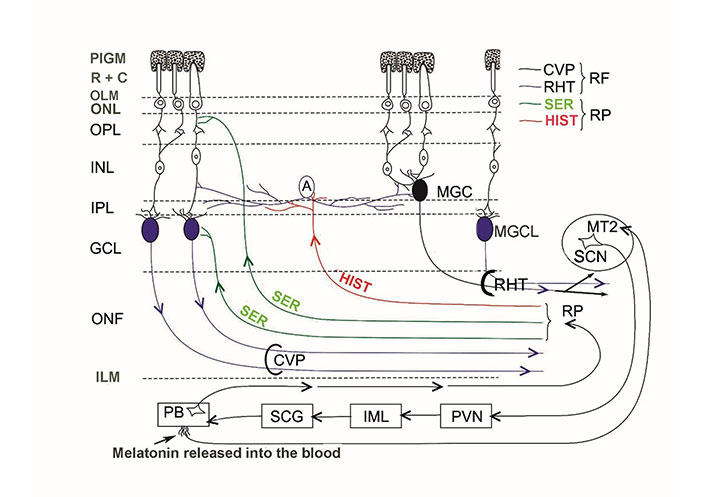
Schematic illustration of the internal neuronal circuit of the retina. A: amacrine cell; CVP: classical visual pathway; ILM: inner limiting membrane; MGC: displaced MPS immunoreactive ganglion cell; MGCL: MPS immunoreactive ganglion cell in the GCL; OLM: outer limiting membrane; ONF: optic nerve fibers; ONL: outer nuclear layer; PIGM: pigment cell layer; R + C: rods and cones; RP: retinopetal fibers
Abbreviations
BDA: |
biotinylated dextran amine |
GABA: |
gamma aminobutiric acid |
GCL: |
ganglion cell layer |
HIST: |
histaminergic fiber |
HR: |
histamine receptor |
HRP: |
horseradish peroxidase |
INL: |
inner nuclear layer |
LHRH: |
luteinizing hormone releasing hormone |
MPS: |
melanopsin |
MT1: |
melatonin receptor 1 |
PACAP: |
pituitary adenylate cyclase activating polypeptide |
PB: |
pineal body |
PVN: |
paraventricular nucleus |
RF: |
retinofugal fibers |
RHT: |
retinohypothalamic tract |
ROR/RZR: |
retinoid-related orphan nuclear receptor family |
SCG: |
superior cervical ganglion |
SCN: |
suprachiasmatic nucleus |
SER: |
serotoninergic fibers |
SON: |
supraoptic nucleus |
SP: |
substance-P |
VIP: |
vasoactive intestinal polypeptide |
VP: |
vasopressin |
Declarations
Author contributions
VV: Conceptualization, Investigation, Writing—original draft, Writing—review & editing. KK: Conceptualization, Investigation, Writing—original draft, Writing—review & editing, Supervision. ÁC: Investigation. All authors read and approved the submitted version.
Conflicts of interest
The authors declare that they have no conflicts of interest.
Ethical approval
Not applicable.
Consent to participate
Not applicable.
Consent to publication
Not applicable.
Availability of data and materials
Not applicable.
Funding
Not applicable.
Copyright
© The Author(s) 2024.