Abstract
Aim:
The aim of this work was to study the effects of extracellular vesicles (EVs) derived from mesenchymal stem cells (MSCs) on inflammation-impaired cognitive functions and the brain of mice.
Methods:
Young mice (~3-month-old) and aged mice (~18-month-old) were injected with bacterial lipopolysaccharide (LPS) and obtained intravenously donor 106 human umbilical cord MSCs, EVs isolated from a similar amount of MSCs or conditioned medium (CM) of MSCs. Subsequently, the mice were examined in behavioral tests and the mouse brains were analyzed for the levels of pro-inflammatory cytokines, α7 nicotinic acetylcholine receptors (α7 nAChRs) and amyloid beta 1-42 (Aβ1-42).
Results:
EVs prevented LPS-induced memory impairment in mice, whereas CM provided a weaker and temporal effect. Both EVs and MSCs injected once after regular injections of LPS stably improved memory of young mice. In contrast, both cells and EVs provided only transient effect in aged mice injected with LPS. The brains of aged LPS-treated mice contained elevated amounts of IL-1β and IL-6; both MSCs and EVs decreased them significantly. The brains of non-treated aged mice contained decreased levels of α7 nAChRs and increased levels of Aβ1-42 and α7-bound Aβ1-42 compared to the brains of young mice. LPS treatment decreased α7 nAChRs in both young and aged mice, while both MSCs and EVs restored them up to the control level. In young mice, LPS treatment increased the level of Aβ1-42 and α7-bound Aβ1-42, whereas MSCs and EVs decreased it. In contrast, neither LPS nor MSCs/EVs influenced the elevated level of Aβ1-42 but increased α7-bound Aβ1-42 in the brains of aged mice.
Conclusions:
Regenerative potential of MSCs and MSC-derived EVs is sufficient to support cognitive functions of LPS-treated young mice but is quite poor for aged animals, possibly, due to decreased levels of α7 nAChRs and accumulated Aβ1-42 in their brains.
Keywords
Mesenchymal stem cells, extracellular vesicles, young or aged mice, inflammation, memory, α7 nicotinic acetylcholine receptor, amyloid beta 1-42Introduction
Neuroinflammation is associated with many neurological and neurodegenerative disorders including Alzheimer’s and Parkinson’s diseases [1]. Neuroinflammation induced in mice by injections of bacterial lipopolysaccharide (LPS) results in memory impairment and accumulation of amyloid beta 1-42 (Aβ1-42) in the brain and is widely accepted as one of animal models of Alzheimer’s disease [2]. α7 nicotinic acetylcholine receptors (α7 nAChRs) are involved in regulating memory and cognition [3], mediate anti-inflammatory signaling [4] and interact with Aβ1-42 [5]; α7 nAChRs expression in the brain is decreased upon neuroinflammation [6].
Mesenchymal stem cells (MSCs) isolated from various sources are now extensively studied as potential therapeutic tools for many pathological states including neuroinflammation and neurodegenerative diseases [7–10]. The data obtained during the last decade demonstrate that intravenously injected MSCs penetrate the brain and stimulate homing and proliferation of neuronal precursor cells resulting in anti-inflammatory effect, inhibition of Aβ accumulation, stabilization of nAChRs in the brain and improvement of episodic memory [11]. It was also shown that therapeutic effects of MSCs are mainly dependent on soluble factors produced and secreted by these cells [12]. However, injecting MSCs-conditioned medium (CM) instead of the cells appeared to produce only temporal effect [11].
MSCs are known to express low level of major histocompatibility complex (MHC) class I molecules enabling researchers to use allogenic and even xenogeneic MSCs in experiments and clinical trials [13, 14]. However, the use of live cells may be circumvented by utilizing MSCs extracellular vesicles (EVs) to avoid issues of immunogenicity and simplifies transplantation tissue storage. In our study, we used EVs, which are up to 1 µm in diameter and can be classified as a mixture of exosomes and microvesicles (MVs) [15, 16]. They were shown to contain enzymes, signaling molecules, and cytokines, as well as miRNAs involved in maintaining cellular homeostasis [17]. They possess properties similar to the cells from which they are derived but have lower immunogenicity. They were shown to cross the blood-brain barrier and to stimulate neurogenesis and angiogenesis within the brain [18].
One of the most popular and evident purposes of therapeutic MSC application is to slow the age-dependent neurodegeneration and to improve (rejuvenate) the state of the aging brain. The promising data in this regard have already appeared in the literature [19]; however, no final conclusion has been published. The aim of our study was to compare the effects of MSCs and associated EVs on the brains of young and aged mice. The results obtained demonstrate that a single injection of either MSCs or EVs stably improved the state of young mice chronically treated with LPS. In contrast, even repeated injections of either MSCs or EVs in aged mice, which had obtained a single injection of LPS, provided only a transient effect, lasting for about a week.
Materials and methods
Materials
Most of reagents were purchased from Sigma-Aldrich (Saint Louis, USA). Antibodies against α7(179-190) or α7(1-208) nAChR fragments were generated and biotinylated by us previously as described [20, 21]. The kits for IL-1β and IL-6 determination (Invitrogen, Waltham, USA) and Neutravidin-peroxidase conjugate (Molecular Probes) were purchased from Thermo Fisher Scientific. Antibody against Aβ1-42 (Cat. #ab2539) was purchased from Abcam.
Animals
We used C57BL/6J female mice 3-month-old and 18-month-old mice from the Institute of Molecular Biology vivarium mice breeding. The mice were kept in the animal facility of Palladin Institute of Biochemistry NAS of Ukraine in quiet, temperature-controlled rooms, and were provided with water and food pellets ad libitum. All procedures were carried out in accordance with the Directive 2010/63/EU for animal experiments and were approved by the Animal Care and Use Committee of Palladin Institute of Biochemistry (Protocol 1/7-421). Cervical dislocation procedure was carried out by skilled operators.
MSC isolation, propagation, and characterization
Umbilical cord MSCs isolation and culture were performed as described previously [12, 22]. At the second passage in culture, they were characterized for the presence of surface marker proteins CD34, CD45, CD90, CD73, and CD105 by flow cytometry (BD FACS Aria) [23] and were used for transplantation into LPS-treated mice. The cell-CM was obtained by culturing the second passage MSCs in serum-free medium for 2 days.
EVs isolation and characterization
The most widely used methods for EVs isolation traditionally include differential ultracentrifugation, size-based isolation, and polymer-based precipitation [24]. In our experiments, isolation of EVs from MSCs CM was performed according to the protocol described in details in [25]. Shortly, MSCs at the 2nd passage were grown up to the 80% confluence, the growth medium containing FBS was changed for DMED/F12 medium without serum, and cell monolayer was left for 24 h in the CO2-incubator. The CM was centrifuged at 3,000× g for 20 min at 4°C and filtrated through 0.22 μm pore filter membranes to remove cell debris and apoptotic bodies. The resulting cell-free supernatant was ultra-centrifuged at 100,000× g for 1 h, 4°C and the pellet containing EVs was re-suspended with freezing medium and frozen at –80°C for later use. The size of the EVs was determined by nanoparticle tracking analysis according to [26] and isolated EVs were further characterized by transmission electron microscopy (TEM, JEM 1230, JEOL, Japan). Ten microliters of vesicle suspension were placed onto formvar-coated TEM grids and dried. Vesicles were stained with 2% (w/v) uranyl oxalate, pH 7.0 for 10 min and then washed with PBS. TEM was performed on a JEM 1200 EX device (Figure 1A).
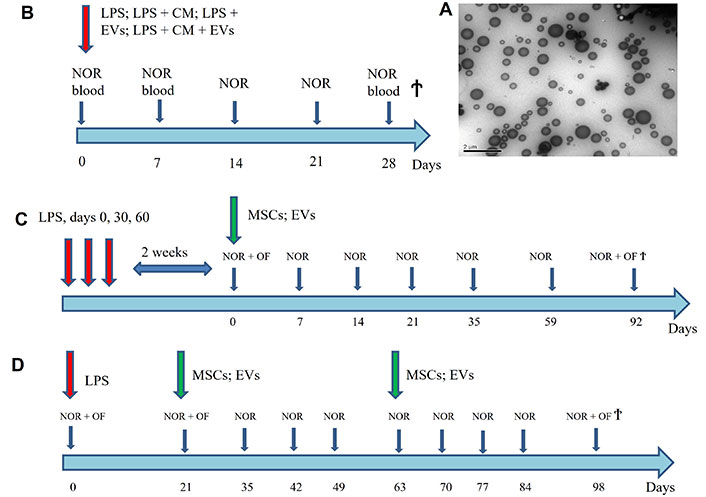
The scheme of experiments showing the time points of LPS, MSCs and EVs injections and those of blood collection, Novel Object Recognition (NOR), Open Field (OF) tests and mice sacrifice (Ϯ) (B–D) and the TEM image of isolated EVs (A). LPS: lipopolysaccharide; MSCs: mesenchymal stem cells; EVs: extracellular vesicles; CM: conditioned medium; TEM: transmission electron microscopy
Animal treatment and brain preparations
In the 1st set of experiments (Figure 1B), we used five groups of 3-month-old C57Bl/6 mice, 5 animals per group. Four groups of mice were intraperitoneally injected with 2 mg kg–1 LPS (E. coli strain 055:B5) in 0.1 mL of saline. The first group obtained a single intravenous injection of EVs obtained from 106 MSCs in 0.1 mL of incubation medium in the tail vein. The second group was injected intraperitoneally with 0.3 mL of MSC-CM. The third group obtained both EVs and CM. All EV or CM injections were performed the same day with LPS injection. The fourth group of mice obtained LPS only and the fifth group was the untreated control. All mice were examined with behavioral testing every week and the blood was taken from the tail vein on days 0, 7, and 28. The mice were sacrificed on day 28 and their brains were removed, homogenized and characterized for the presence of α7 nAChRs as described below. The blood sera, obtained by the standard procedure, were analyzed by ELISA for the presence of antibodies specific to CM components.
In the 2nd set of experiments (Figure 1C), we used four groups of 3-month-old mice, 5 animals in each. Three groups of mice were injected with 2 mg kg–1 LPS (E. coli strain 055:B5) in 0.1 mL of saline on days 0, 30, and 60, the 4th group remained intact. Two weeks after the last LPS injection (qualified further as day 0), one group of LPS-treated mice obtained a single intravenous injection of 0.1 mL of 106 MSCs and another group obtained intravenously 0.1 mL of EVs derived from 106 MSCs. The third group obtained LPS injections only. The mice were examined with behavioral tests every one or two weeks up to the day 35 and then on days 59 and 92 after the MSC/EV injection. Then they were sacrificed, the brains were removed, homogenized in detergent-containing buffer as described [6] and used for determination of cytokines, α7 nAChRs and Aβ1-42 by Sandwich ELISA.
In the 3rd set of experiments (Figure 1D), we used four groups of 18-month-old mice, 5 animals in each. One group remained intact, while other three ones were injected once with 2 mg kg–1 LPS (E. coli strain 055:B5) in 0.1 mL of saline. On days 21 and 56 after the LPS injection, one group obtained an intravenous injection of 106 MSCs and another one obtained 0.1 mL of EVs derived from 106 MSCs; the third group obtained LPS only. The mice were examined with behavioral tests every one or two weeks and were sacrificed on day 98 after LPS injection. Their brains were homogenized in detergent-containing buffer as described [6] and used for determination of cytokines, α7 nAChRs and Aβ1-42 by Sandwich ELISA.
ELISA assays
The levels of α7 nAChR subunits, Aβ1-42 and Aβ1-42 bound to α7 nAChRs in the brain preparations were determined by Sandwich ELISA as described previously [6, 12]. Briefly, Nunc Maxisorp immunoplates were coated with either rabbit α7(1-208)-specific antibody or Aβ1-42-specific antibody (20 µg/mL). The detergent lysates of brain tissue (1 µg of protein per 0.05 mL per well) were applied for 2 h at 37°C and the bound α7 subunits or Aβ1-42 were detected with the second biotinylated α7(179-190)-specific or Aβ1-42-specific antibody, Neutravidin-peroxidase conjugate and o-phenylendiamine-containing substrate solution.
The CM-specific antibodies in the blood sera of mice were determined by direct ELISA. The immunoplates were coated with CM (nondiluted, 2 h, 37°C), blocked with 1% BSA, and the sera (diluted 1:50) were applied for additional 2 h at 37°C followed by peroxidase-labeled goat anti-mouse IgG (Fab-specific) and o-phenylendiamine-containing substrate solution.
The cytokine levels (IL-1β and IL-6) were measured by Sandwich ELISA according to recommendations of kits manufacturer (Invitrogen, Waltham, USA).
The optical density was read at 490 nm for o-phenylendiamine and at 450 nm for tetramethyl benzidine using Stat-Fax 2000 ELISA Reader (Awareness Technologies, USA).
Behavioral experiments
Mice were tested in the “Novel Object Recognition” (NOR) and “Open Field” (OF) behavioral tests as described [27, 28]. In the NOR test, the mice at first explored two identical objects for 10 min followed by a 10 min break. In the second session, one object was replaced by a novel one of similar size but different shape or color and the time of explorations of the two objects was registered in subsequent 10 min session. The results of NOR test are presented as discrimination index (DI) calculated as the difference in the time (in seconds) of “novel” and “familiar” object explorations divided by the total time of explorations of two identical objects.
In the NOR test, mice first explored two identical objects (right and left) for 10 min, followed by a 10-min break. During the second session, one of the two identical objects (hereafter “familiar”) was replaced by another one of the similar size but different shape or color (hereafter “novel”), and the exploration time of each of the two different objects was recorded during the next 10-min sessions. The results of the NOR test are presented as a DI, which is calculated as the difference in the study time of the “novel” and “familiar” objects in seconds of the session, divided by the total time spent studying the two identical objects (in the first session). The lack of preference for a new object, expressed as a decrease in DI, was qualified as episodic memory impairment.
In the OF test, the animals were individually placed in a rectangular box and were observed during 10 min. Total locomotor activity was evaluated as the time (in seconds) of running, rearing and climbing activity. The anxiety index (IA) was calculated according to [28] by the following formula: IA = (TA + cr)/ha, where TA is total anxiety calculated as a total number of vertical rears, grooming, boluses, and urinations during examination period, cr is the number of center runs and ha (horizontal activity) is the sum of center and wall runs.
Subsequently, mice were offered two identical objects and total explorative activity was expressed as the time spent in examination of each object plus the time of rearings/climbings.
Statistical analysis
ELISA experiments, as well as behavioral experiments were performed in triplicates for each mouse and mean values for individual mice were used for statistical analysis using one-way ANOVA and Origin 9.0 software. The data are presented as mean ± SD. Differences between groups were considered significant at P < 0.05.
Results
EVs characterization
According to nanoparticle tracking analysis, the peak size value of isolated EVs was about 100 nm that corresponded to exosomes [15]. However, according to TEM, the particles were quite heterogeneous (Figure 1A) and, most probably, there was a mixture of exosomes and MVs [15]. The specific markers are still lacking to distinguish MVs from exosomes [16] and their biological functions seem to be quite similar [15]. We will further classify the particles used in our experiments as EVs.
Comparative effects of MSC-derived EVs and MSC-CM on behavior and brains of young mice
In the first set of experiments, we compared the effect of EVs isolated from MSCs CM with that of CM itself in young (3-month-old) mice. As shown in Figure 2A, LPS injection resulted in significant impairment of episodic memory during two weeks followed by a partial improvement on weeks 3 and 4. EVs injected simultaneously with LPS prevented memory impairment caused by LPS. In contrast, CM injection provided a weaker effect which lasted two weeks only. Combination of EVs with CM affected mice similarly to EVs alone. The brains of mice injected with LPS contained decreased amounts of α7 nAChRs (Figure 2B).
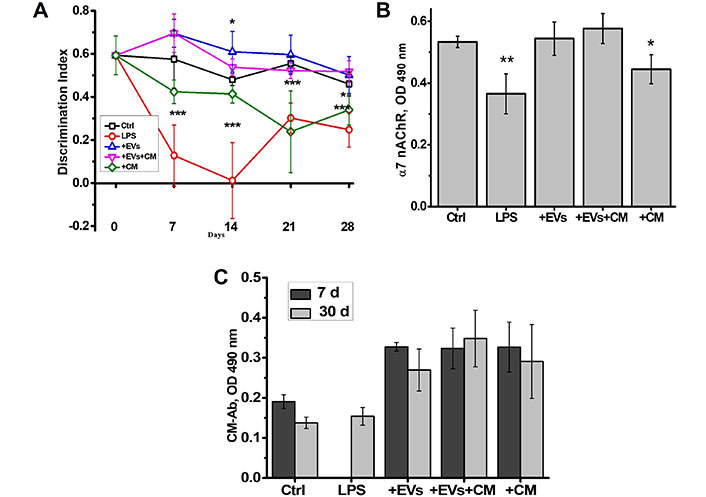
The EVs isolated from the MSCs-conditioned culture medium are efficient similarly to MSCs and are more efficient compared to MSC-CM. The effects of MSC-derived EVs, MSC-CM or their combination (EVs + CM) on episodic memory of LPS-injected young mice (A); the level of α7 nAChRs in their brains (B); and the level of CM-specific antibodies (Ab) in the blood (C). LPS (ip), MSCs (iv) or CM (ip) were injected on day 0. Each column or point corresponds to Mean ± SD, n = 5. * P < 0.05; ** P < 0.01; *** P < 0.001 compared to Ctrl. CM: conditioned medium; Ctrl: control; LPS: lipopolysaccharide; EVs: extracellular vesicles; α7 nAChRs: α7 nicotinic acetylcholine receptors; MSCs: mesenchymal stem cells
EVs prevented such decrease, CM was less efficient and combination of EVs and CM provided an effect similar to EVs alone. These data indicated that EVs were more efficient than CM and the latter did not contain any additional factors to improve EV effect.
Previously, we reported that injections of CM in mice stimulated production of antibodies against the CM components [11]. It was suggested that these are the antibodies, which diminish the CM effect. Here, we observed that both CM and EVs stimulated the appearance of CM-specific antibodies on day 7 after injection already (Figure 1C). Given the stronger and longer effect of EVs compared to that of CM, it was concluded that the CM-specific antibodies did not influence the EV efficiency.
The weak signal for the antibodies against MSC-CM in the blood of control and LPS-treated mice shown in Figure 2C most probably relates to “natural” or polyreactive antibodies capable of recognizing the MSC-released metabolites. Injections of CM, EVs, or their combination clearly stimulated such antibodies production. The fast increase of the antibody level in the blood (day 7) confirms that B lymphocytes of corresponding specificity are already present in control mice.
Behavioral effects of either MSCs or MSC-derived EVs in the young and aged mice
In this set of experiments, young mice (3-month-old) were subjected to chronic LPS treatment by way of three intraperitoneal LPS injections over two months. Either MSCs or MSC- derived EVs were injected once on day 14 after the last LPS injection (qualified as day 0 in Figure 3A). The behavioral tests were conducted every one or two weeks up to the day 35 and then on days 59 and 92. Figure 3A shows that the memory was improved in two weeks during the first 35 days after MSC/EV injection and Figure 3B illustrates that the memory of LPS + MSCs and LPS + EVs - injected mice was effective up to the day 92, while memory of LPS only-injected mice remained impaired during the three months after the last LPS injection.
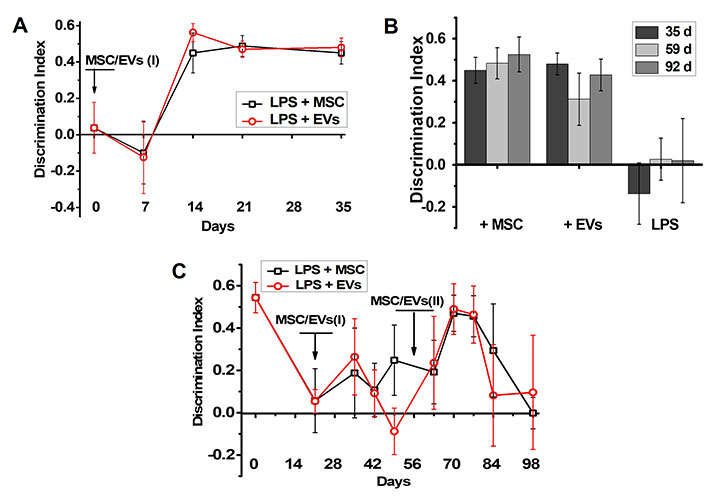
MSCs and MSC-derived EVs improve memory of the young but not old LPS-treated mice. The effect of MSCs or MSC-derived EVs on episodic memory of either young (A, B) or aged mice (C) treated with LPS. Arrows indicate the time points of MSC/EV injections. Figure 3A shows how fast the memory of young mice was improved during the first 35 days after MSC/EV injection and Figure 3B illustrates how long the memory of LPS + MSCs, LPS + EVs and LPS-only-injected young mice was maintained. Discrimination index of non-treated mice was 0.6 ± 0.09. LPS: lipopolysaccharide; MSC: mesenchymal stem cell; EVs: extracellular vesicles
The aged mice (18-month-old) obtained a single LPS injection, which decreased their episodic memory on day 21 (Figure 3C). Either MSC or EV injection slightly improved their memory after two weeks (day 35), but the effect was transient and diminished one week thereafter. Repeated injection of either MSCs or EVs performed five weeks after the first injection (day 56) improved the mice memory again in two weeks (day 70) and, again, the effect lasted for one week only (Figure 3C).
According to the OF behavioral studies performed at the final stage of experiment, before sacrifice, the young mice demonstrated higher locomotor activity compared to aged mice. LPS injections decreased the higher locomotive activity in the young mice, whereas either MSCs or EVs restored the activity up to the control level. LPS treatment did not change significantly the locomotor activity of aged mice, while either MSCs or EVs augmented it up to the level of young mice (Figure 4A, left part). The explorative activity of young mice also decreased under the effect of LPS and was not significantly affected by MSC or EV injections. In contrast, neither LPS nor MSCs/EVs affected explorative activity of aged mice, which was even higher than that of young mice under the effect of MSCs/EVs (Figure 4A, right part). The IA of aged mice was initially higher than that of young mice and was not affected by either LPS or MSC/EV injections. The IA of young mice increased under the effect of LPS and was decreased by MSC/EV injections (Figure 4B).
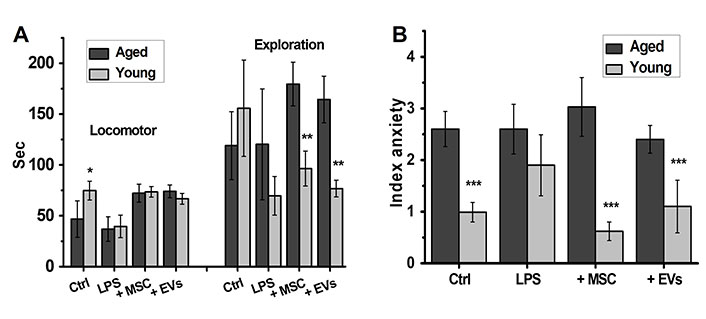
MSCs and EVs improve the locomotor activity of both young and aged mice and decrease anxiety in young mice only. Locomotor, explorative activity (A) and anxiety (B) of either young or aged LPS-treated mice injected with MSCs or EVs. * P < 0.05; ** P < 0.01; *** P < 0.001 compared to aged mice. LPS: lipopolysaccharide; MSC: mesenchymal stem cell; EVs: extracellular vesicles
The effects of either MSCs or MSC-derived EVs in the brains of young and aged mice
At the end of behavioral experiments, the mice were sacrificed and their brains were examined for the levels of pro-inflammatory cytokines, α7 nAChRs and Aβ1-42. As shown in Figure 5, the brains of LPS-treated young mice did not contain elevated amounts of IL-1β or IL-6 at the end of experiment (more than 100 days after LPS injection); however, both MSCs and EVs decreased IL-6 levels in their brains. In contrast, the brains of some aged mice treated with LPS still contained elevated amounts of both IL-1β and IL-6 and either MSCs or EVs decreased them significantly.
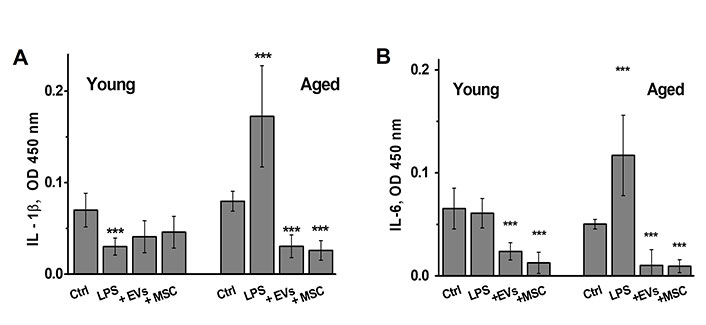
The brains of aged LPS-treated mice contain elevated amounts of IL-1β and IL-6, which are decreased by MSCs or EVs injections. The levels of IL-1β (A) and IL-6 (B) in the brains of either young or aged mice treated with LPS or LPS + MSCs/EVs. * P < 0.05; ** P < 0.01; *** P < 0.001 compared to control mice. LPS: lipopolysaccharide; MSC: mesenchymal stem cell; EVs: extracellular vesicles
The level of α7 nAChRs in the brains of young non-treated mice was higher than that in aged mice (Figure 6A). LPS decreased it in both young and aged mice, while both MSCs and EVs restored it up to the control level; the amplitude of these changes was higher in the brains of young compared to aged mice.
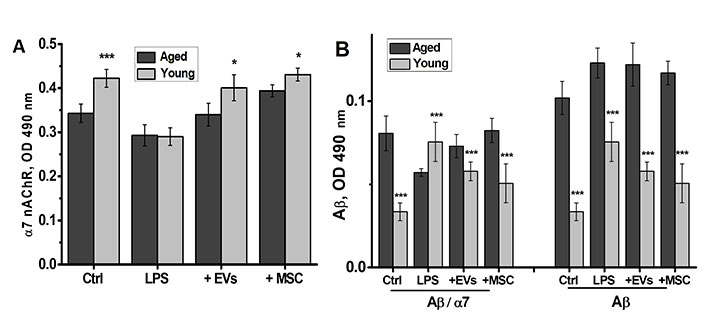
MSCs and EVs restore α7 nAChR levels and tend to decrease the levels of Aβ1-42 in the brains of LPS-treated young but not aged mice. The levels of α7 nAChR (A) and Aβ1-42, either free or α7-bound (B), in the brains of young and aged mice treated with LPS or LPS + MSCs/EVs. * P < 0.05; ** P < 0.01; *** P < 0.001 compared to aged mice. MSC: mesenchymal stem cell; EVs: extracellular vesicles; α7 nAChR: α7 nicotinic acetylcholine receptor; Aβ1-42: amyloid beta 1-42; LPS: lipopolysaccharide
The level of Aβ1-42 in the brains of aged mice was much higher than that in young mice (Figure 6B, right panel). In young mice, LPS treatment increased the level of Aβ1-42, whereas either MSCs or EVs decreased it. In contrast, neither LPS nor MSCs/EVs influenced the elevated level of Aβ1-42 in the brains of aged mice. The brains of aged mice contained more α7-bound Aβ1-42 compared to that in young mice (Figure 6B, left panel). LPS treatment increased α7-bound Aβ1-42, whereas both MSCs and EVs decreased it in young mice. In contrast, LPS treatment decreased the level of α7-bound Aβ1-42 and MSCs/EVs restored it in the brains of aged mice.
Discussion
In this paper, we show that EVs secreted by human umbilical cord MSCs efficaciously mitigate the consequences of neuroinflammation in young but not in aged mice. The EVs isolated from the MSCs-conditioned culture medium (and concentrated to correspond the quantity of MSCs) appeared to be efficacious similarly to MSCs used in the same experiment and much more efficacious compared to MSC-CM. Therefore, MSC-secreted EVs contained complete set of growth/nutrition factors sufficient to overcome the consequences of neuroinflammation. Taking into account that EVs are less immunogenic than the cells, from which they derive, and can be easily accumulated and stored before transplantation, their use in regenerative medicine looks preferable compared to MSCs.
A single MSC/EV injection performed after repeated LPS treatment stably restored episodic memory, decreased anxiety and improved locomotor and explorative activity of young mice. This was not the case with aged mice that obtained only one LPS injection. The aged mice initially demonstrated higher anxiety and lower locomotor activity compared to young mice. In aged mice, even repeated injections of MSCs/EVs only transiently improved episodic memory impaired by LPS and did not influence anxiety or explorative activity. However, MSCs/EVs-treated aged mice looked healthier than their LPS-only-treated counterparts and demonstrated better locomotor activity. Therefore, MSC/EV treatment was beneficial for aged mice as well but did not influence their cognitive capacity. Our experiments were performed in female mice because they are calmer and less aggressive compared to males; this is especially important in long lasting experiments. We do not think that estrogen variations between young and aged female mice are more significant compared to individual testosterone levels in male mice within the group.
The study of mouse brains supported and partly explained the data obtained in behavioral experiments. Thus, it was shown that at least some of aged LPS-treated mice maintained elevated levels of pro-inflammatory cytokines in the brain for a long time; therefore, they were initially less capable to resolve neuroinflammation compared to young mice. This can be due to low level of α7 nAChRs known to mediate anti-inflammatory signaling [4]. MSCs/EVs evidently helped resolving inflammation (and stimulated α7 nAChR increase) that could result in better health and locomotor activity of MSC/EVs-treated mice. However, this did not improve memory of LPS-treated aged mice, probably, because of high level of Aβ1-42 in their brains, which was not affected by either LPS or MSC/EVs treatment. Of interest is also the fact that MSCs/EVs prevented Aβ1-42 interaction with α7 nAChR in young mice but favored it in aged mice. Previously, we reported that neuroinflammation caused by LPS injection is usually accompanied by the decrease of α7 nAChRs, accumulation of soluble Aβ1-42 and elevation of α7-Aβ1-42 complexes in the brains of mice, whereas therapeutic interventions, which improved memory of LPS-treated mice (PNU292097, NSE, MSCs), resulted in restoration of α7 nAChR levels and the decrease of α7-Aβ1-42 complexes [6, 11, 29]. This data suggests that complexing α7 nAChRs with Aβ1-42 is disadvantageous for the brain functioning. Possibly, high level of Aβ1-42 accumulated in the brains of aged mice and minor increase of α7 nAChRs under the effect of MSCs/EVs favored α7-Aβ1-42 interaction. However, this may not be the only reason for inefficacy of MSCs/EVs in aged mice, which may be less sensitive to MSC-derived growth/nutrition factors due to altered expression of corresponding receptors or impairment of their signaling pathways. Previously we reported that injected MSCs stimulated migration of neuronal progenitor cells to the brain of LPS- treated young mice supporting their cognitive functions [11]. The aged mice have significantly less neural progenitor cell proliferation, neuronal differentiation, and newborn neuron survival [30] leading to lower regenerative potential compared to young animals. Therefore, MSCs and MSC-derived EVs are efficacious in supporting cognitive functions of young mice and are quite poor for aged animals.
Abbreviations
Aβ1-42: | amyloid beta 1-42 |
CM: | conditioned medium |
DI: | discrimination index |
EVs: | extracellular vesicles |
IA: | anxiety index |
LPS: | lipopolysaccharide |
MSCs: | mesenchymal stem cells |
MVs: | microvesicles |
NOR: | Novel Object Recognition |
OF: | Open Field |
TEM: | transmission electron microscopy |
α7 nAChRs: | α7 nicotinic acetylcholine receptors |
Declarations
Acknowledgments
Thanks are due to Dr. Raymond Sullivan for language revision. Professor Maryna Skok, one of the main authors, passed away on April 11th, 2024.
Author contributions
OL: Investigation, Writing—review & editing. OK: Investigation, Writing—review & editing. MS: Conceptualization, Writing—original draft, Writing—review & editing. OD: Conceptualization, Writing—original draft, Writing—review & editing. OG: Investigation. OT: Investigation. IP: Investigation. VK: Conceptualization, Validation, Writing—review & editing, Supervision.
Conflicts of interest
The authors declare that they have no conflicts of interest.
Ethical approval
All procedures with animals were approved by the Animal Care and Use Committee of Palladin Institute of Biochemistry, Protocol 1/7-421.
Consent to participate
Not applicable.
Consent to publication
Not applicable.
Availability of data and materials
All data can be obtained from authors upon request.
Funding
Not applicable.
Copyright
© The Author(s) 2024.