Abstract
Aim:
Neuroinflammation is a characteristic of multiple sclerosis (MS). Resveratrol (RSV) has potent antioxidant properties and has emerged as a promising therapeutic agent for various inflammatory diseases. This study investigated the effects of RSV on inflammatory responses via reactive oxygen species (ROS) production and leukocyte cytokine secretion in patients with MS and healthy controls.
Methods:
The effects of RSV on ROS production in resting and stimulated granulocytes (in the presence of opsonized particles) were assessed using luminol-dependent chemiluminescence. The cytokines interleukin (IL)-10, IL-1β, IL-6, and high mobility group box 1 (HMGB1) in the supernatant of peripheral blood mononuclear cells (PBMNCs) were quantified using enzyme-linked immunosorbent assay (ELISA).
Results:
RSV significantly downregulated ROS production in resting and stimulated granulocytes in patients with MS and healthy controls. In the control group, RSV reduced IL-6 levels by 49% in the PBMNC supernatant, whereas IL-6 levels remained unchanged in the MS group. Interestingly, higher levels of IL-10 were detected in PBMNC supernatants from patients with MS than in controls. No significant changes were observed in IL-1β and HMGB1 levels in the PBMNC supernatant.
Conclusions:
Controlling ROS production is a key target for treating inflammatory diseases. Our findings suggest that RSV can effectively modulate ROS production in MS, highlighting its potential as a promising adjunct therapy for controlling oxidative innate immune responses in MS.
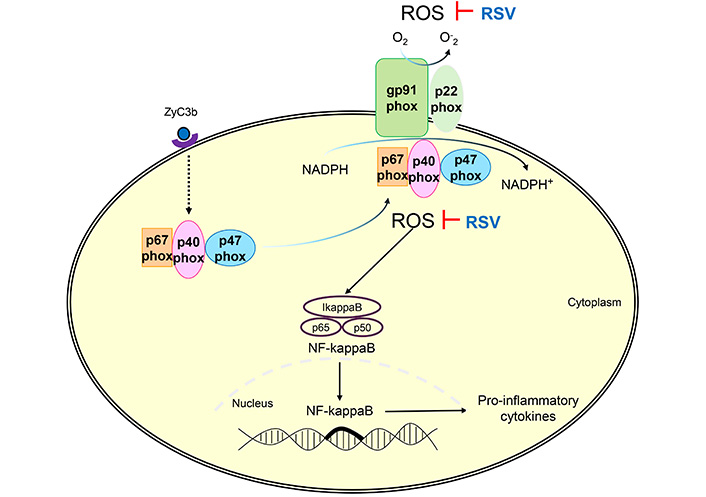
RSV inhibited the ROS production via NADPH-oxidase of patients with MS. ROS: reactive oxygen species; RSV: resveratrol; ZyC3b: zymosan recovered using C3b fragments (opsonized particles); MS: multiple sclerosis
Keywords
Resveratrol, multiple sclerosis, innate immunity, reactive oxygen species, inflammation, cytokineIntroduction
Multiple sclerosis (MS) is a chronic inflammatory demyelinating disease of the central nervous system (CNS), making it the second most prevalent cause of neurological disability among young adults, and impacting approximately 2.5 million individuals globally [1–4].
There is no consensus regarding the etiology of MS. Several theories have been proposed, including idiopathic loss of self-tolerance, altered blood-brain barrier (BBB) permeability due to unknown mechanisms, molecular mimicry, and chronic viral infection. These factors, combined with genetic predispositions and environmental influences, collectively contribute to the development of this disease [5–8].
Infiltration of immune cells into the CNS in MS is closely associated with producing reactive oxygen species (ROS). Immune cells such as autoreactive T and B lymphocytes, macrophages, and dendritic cells produce ROS, contributing to chronic inflammation and tissue damage in the CNS. Excessive ROS production can lead to lipid peroxidation, DNA damage, and cell death and plays a pivotal role in demyelination, axonal/neural injury, and modulation of BBB integrity. This process can result in partial or total disruption of neuronal signaling, contributing to disease progression and symptoms observed in MS, including sensory and visual disturbances, motor deficiencies, fatigue, pain, and cognitive deficits [9–14].
There is no cure for MS. Treatment is based on immunosuppression intended to modulate the inflammatory component of the disease. Still, most immunosuppressants have limited efficacy and serious side effects, and some patients are non-responsive or develop tolerance [15–18]. Adjunctive therapies, such as vitamins and dietary supplements, are being explored and are considered acceptable for patients with MS [19].
Resveratrol (trans-3,4’,5-trihydroxystilbene, RSV), a naturally derived polyphenol found in grapes, seeds, and red wine, has the potential to affect human diseases, including neurodegenerative diseases, ischemia/reperfusion, and depression [20–23]. RSV has recently emerged as a potential therapeutic agent for treating various inflammatory diseases, owing to its anti-inflammatory and antioxidant properties [24]. RSV is commonly addressed in several pathologies, and its effects are evident in cardioprotection, neuroprotection, improving remyelination, immune modulation, and malignant neoplastic cells. It is water-soluble and can cross the BBB [25–38]. Despite its promising therapeutic potential, RSV may cause side effects, especially when administered at high doses or for extended periods, including gastrointestinal discomfort, allergic reactions, and interactions with other medications [39–44]. The mechanism of action of RSV involves its ability to activate various cellular pathways, including sirtuin activation, AMP-activated protein kinase (AMPK) activation, and modulation of nuclear factor-kappa B (NF-κB) signaling, which is involved in the production of pro-inflammatory cytokines [23, 45, 46]. RSV can also modulate oxidative stress by downregulating ROS production through the NADPH-oxidase (NOX) complex and mitochondria [37, 47–49]. ROS and pro-inflammatory cytokines play essential roles in the pathogenesis of MS by inducing severe CNS damage [50, 51]. Therapeutic targeting based on the inflammatory response represents an exciting approach to developing novel neuroprotective strategies [52, 53]. Several studies have shown that RSV may be beneficial for treating chronic diseases involving oxidative stress and inflammation. Therefore, we hypothesized that RSV prevents inflammation via ROS production and cytokine secretion in the leukocytes of patients with MS.
Materials and methods
Study population
This comparative cross-sectional parallel-group study was approved by Dr. Francisco das Chagas Lima and Silva Ethics Committee of Santa Casa Hospital of Belo Horizonte, Brazil (approval number 69385917.7.0000.5138). Informed consent was obtained from all participants. Peripheral venous blood was collected from twenty-four MS patients and twenty-two healthy individuals (control group) aged 21 to 60. Table 1 displays the detailed profiles of the study population, including disease duration, disease course, and degree of disability, as measured by the expanded disability status scale (EDSS) and categorized as mild (EDSS 0–2.5), moderate (EDSS 3–5), or severe (EDSS 5.5–10). The EDSS, which ranges from 0 to 10, quantifies disability progression in patients with MS based on a neurologist’s examination, with higher scores indicating worse disability [54]. All patients in the MS group were non-smokers and had received immunotherapy. The exclusion criteria were pregnancy, dementia, inflammation, malignant disease, infection, and tobacco or alcohol dependence.
Characteristics of study subjects
Parameters | Control group | Multiple sclerosis | P |
---|---|---|---|
N | 22 | 24 | Ns |
Sex, N (%) Female b | 16 (72.7%) | 16 (66.6%) | Ns |
Age in years a | 38.4 ± 14 | 35.5 ± 10 | Ns |
Disease duration in years a | Na | 15.9 ± 12.5 | - |
EDSS | |||
Mild, N (%) | Na | 17 (70.8%) | - |
Moderate, N (%) | Na | 4 (16.7%) | - |
Severe, N (%) | Na | 3 (12.5%) | - |
Disease course | |||
Relapsing-remitting, N (%) | Na | 20 (83.3%) | - |
Primary progressive, N (%) | Na | 4 (16.7%) | - |
a Values expressed in mean ± standard deviation, student’s t-test; b values expressed in percentage, χ2 test. Na: not applicable; Ns: non-significant (P > 0.05). Expanded disability status scale (EDSS) scores were categorized as mild, no to minimal disability (EDSS 0–2.5), moderate (EDSS 3–5), and severe (EDSS 5.5–10)
Preparation of leukocytes
Granulocytes and peripheral blood mononuclear cells (PBMNCs) were purified using a modified version of the Ficoll-Hypaque density gradient method as described by Bicalho et al. [55]. Cells were identified and counted based on their morphology, granulation, and size in a Neubauer chamber. The final concentration of the suspension was adjusted to 1 × 106 cells/mL. The viability of the cell suspensions was determined using the trypan blue exclusion test, and cell viability exceeded 90% for all samples.
Reactive oxygen species assay
ROS levels in the granulocytes were determined using a luminol-based chemiluminescence method. Aliquots (100 µL) containing a suspension of granulocytes in phosphate-buffered saline (PBS) at a concentration of 1 × 106 cells/mL were mixed with luminol (0.4 M dissolved in DMSO) in the presence or absence of RSV (10 μM, 3,4’,5-tri-hidroxi-trans-estilbeno, 5-[(1E)-2-(4-hidroxifenil)etenil]-1,3-benzenodiol, 3,5,4’-trihidroxiestil-beno, cat. #5010 Merck KGaA) and opsonized particles [13.6 mg/mL zymosan-C3b suspension, zymosan recovered using C3b fragments (opsonized particles) (ZyC3b), zymosan-A from saccharomyces cerevisiae, cat. #Z4250 Merck KGaA]. Sequential reactions were conducted over 30 min and monitored using a Turner BioSystems (Promega, Madison, WI, USA) model 20/20n luminometer [56–59].
Cytokine detection in PBMNC supernatant
Aliquots (100 µL) containing a suspension of PBMNCs in Dulbecco’s modified Eagle’s medium (DMEM) supplemented with 10% fetal bovine serum (FBS) at a concentration of 1 × 106 cells/mL from both patients and controls were distributed in triplicate in the presence or absence of RSV (10 μM) among the wells of 96-well flat-bottomed culture plates (Corning Inc., New York, USA). The plates were incubated for 72 h at 37℃ under 5% CO2. Following incubation, PBMNC culture supernatants were collected, and the concentrations of interleukin (IL)-10, IL-1β, IL-6, and high mobility group box 1 (HMGB1) were determined using enzyme-linked immunosorbent assay (ELISA) kits. IL-10, IL-1β, and IL-6 were obtained from BioLegend, Inc., San Diego, USA. The HMGB1 cells were obtained from FineTest (Wuhan Fine Biotech Co., China).
Statistical analysis
Statistical analyses were performed using GraphPad Prism version 5 (GraphPad Software, Inc.). Data are presented as the mean ± standard error (SE) or median (minimum-maximum) as appropriate. An unpaired student’s t-test or the Whitney U-test was used to compare samples. In certain cases, the χ2 test was used. P < 0.05 was considered significant.
Results
Table 1 shows a detailed profile of the study population. This study included 22 healthy individuals (control group), with a mean age of 38.4 ± 14 years, and 24 MS subjects, with a mean age of mean age 35.5 ± 10 years and disease duration of 15.9 ± 12.5 years, were included in this study. There were no significant differences in age or sex between the two groups. According to the International Advisory Committee on Clinical Trials of MS 20 with MS were diagnosed with a relapsing-remitting course, and 4 with primary progressive [60, 61]. According to the EDSS scale, 17 MS patients with no-minimal disability were classified in the EDSS 0–2.5, 4 patients in the EDSS 3–5, with moderate disability, and 3 patients in the EDSS 5.5–10, with severe disability.
RSV inhibited ROS production in resting and phagocytosis-stimulated granulocytes
Figure 1 presents the oxidative response in non- and stimulated granulocytes across the studied groups, values expressed as the mean ± SE. The findings indicate comparable levels of ROS in resting granulocytes from patients with MS (198.4 ± 19) and controls (187.1 ± 19). However, upon stimulation with opsonized particles, ROS generation was significantly higher (P < 0.05) in granulocytes from patients with MS (883.6 ± 109.4) than those from healthy individuals (420.8 ± 30.1). Additionally, the addition of RSV inhibited ROS generation in both resting cells (controls: 84.1 ± 5.4, MS: 85 ± 3.3) and phagocytosis-stimulated granulocytes (controls: 111.9 ± 113.2, MS: 159.3 ± 22.3) from both groups. These findings demonstrate that RSV effectively suppresses ROS production in granulocytes when exposed to opsonized particles, indicating inhibition during phagocytosis.
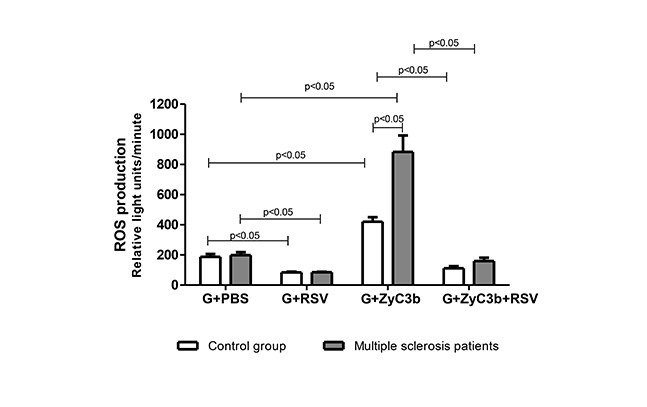
The effect of RSV (10 μM) on ROS production during phagocytosis of opsonized ZyC3b particles in granulocytes obtained from multiple sclerosis patients and control group. Values expressed in mean ± standard error; student’s t-test; n = 12 for each group. G: granulocytes; PBS: phosphate-buffered saline; ROS: reactive oxygen species; RSV: resveratrol; ZyC3b: zymosan recovered using C3b fragments (opsonized particles)
Cytokine levels in the absence and presence of RSV
To investigate whether the observed RSV-mediated downregulation of ROS production in granulocytes from patients with MS was associated with modulation of anti- or pro-inflammatory cytokines production, supernatants from PBMNC cultures treated with or without RSV were analyzed for IL-10, IL-1β, IL-6, and HMGB1 levels. As shown in Figure 2, PBMNCs from patients with MS exhibited a significantly higher (P < 0.05) IL-10 secretion (162.3 ± 13.2 pg/mL) compared with those from the control group (71 ± 9.5 pg/mL) (Figure 2A). Although IL-6 levels were not significantly different between the two study groups, the presence of RSV led to a significant inhibition (49%) of IL-6 secretion in PBMNCs from the control group. In contrast, no alteration was observed in patients with MS (Figure 2C). The levels of IL-1β and HMGB1 in PBMNCs from both groups showed no significant differences and were not modulated by the presence of RSV (Figure 2B and D).
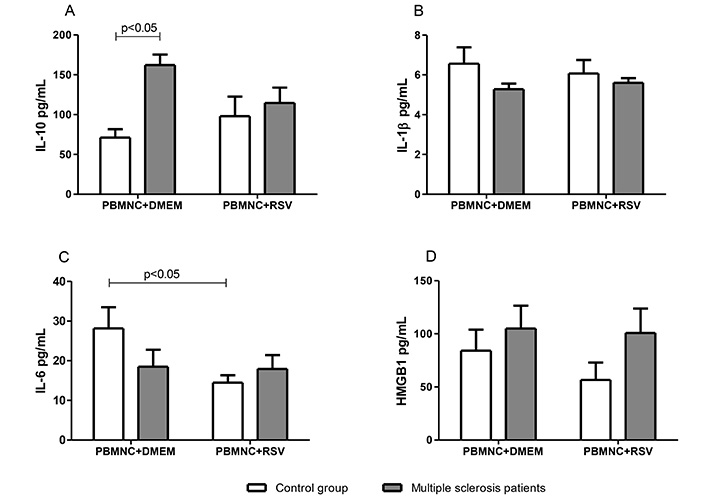
The modulation by RSV (10 μM) of cytokine production in PBMNC from multiple sclerosis patients and controls. (A) Levels of IL-10; (B) levels of IL-1β; (C) levels of IL-6; (D) levels of HMGB1. Values expressed in mean ± standard error; n = 12 for each group. In panels B and D, no significant differences were observed in any of the analyses. DMEM: Dulbecco’s modified Eagle’s medium; HMGB1: high mobility group box 1; PBMNC: peripheral blood mononuclear cell; RSV: resveratrol
Discussion
This study examined the potential immunomodulatory effects of RSV on leukocytes from patients with MS. Our results demonstrated that RSV inhibited inflammation-associated ROS production in unstimulated and stimulated granulocytes from patients with MS and healthy controls (Figure 1). RSV did not affect cytokine secretion in cells from patients with MS; however, this antioxidant decreased IL-6 levels in mononuclear cells from the control group (Figure 2). Furthermore, our results showed high levels of IL-10 secreted by cells from patients with MS (Figure 2A).
The pathophysiology of MS remains unclear; nonetheless, neuroinflammation is a hallmark of the disease, characterized by the influx of leukocytes into the CNS and the loss of BBB integrity [11, 52, 53]. Immune cells in the CNS are a major source of ROS production and secretion of pro-inflammatory cytokines, leading to demyelination, neuronal damage, and functional disabilities [25–35]. Antioxidant therapy may be a good option for controlling the inflammatory processes in neurodegenerative pathologies [62–68].
An oral pharmaceutical formulation of RSV, SRT501, prevented neuronal damage and associated long-term neurological dysfunction in a mouse model of MS [40, 69]. The neuroprotective effects of RSV are associated with sirtuin 1 (SIRT1), an NAD-dependent deacetylase that regulates various cellular functions, including energy metabolism and cellular stress responses [70]. Reduced SIRT1 levels have been observed in several chronic inflammatory diseases, such as arterial inflammation, obesity, and Alzheimer’s disease [71]. Additionally, low levels of SIRT1 contribute to the upregulation of IL-1β levels in chronic inflammation and aging microglia of experimental models [72]. RSV’s neuroprotective effects of RSV may be linked to its ability to ameliorate oxidative stress, inflammation, and mitochondrial dysfunction [38]. Oxidative stress is pivotal in neurodegenerative diseases such as MS, causing demyelination, neuronal damage, and BBB disruption [73–79]. ROS, primarily produced by NOX isoforms in response to growth factors or cytokines, exacerbates these conditions [80, 81]. In parallel with previous studies implicating the antioxidant action of RSV through its inhibitory effects on NOX activity, our experiments also indicated that RSV significantly attenuated ROS production in non-stimulated and stimulated granulocytes from patients with MS and controls (Figure 1). In aortic smooth muscle cells (SMCs), RSV stimulates the expression of antioxidant genes and acts as a ROS scavenger [82–84].
RSV has also been identified as a negative regulator of NF-κB signaling by improving oxidative stress, given that NF-κB can be activated by a wide variety of signals, including oxidative stress [85]. Although our results indicated that RSV downregulated ROS production in cells from patients with MS and controls, peripheral immune cells from patients with MS remained unaltered upon RSV treatment (Figure 2). This could be because i) in this study, the molecular mechanisms of MS influence the activity of these antioxidants, or ii) assessment of the cytokine profile in these cells is not possible since it is common to observe the inflammatory response in loco in the CNS [86–88]. Only IL-6 levels were affected by RSV in mononuclear cells of the control group (Figure 2C). Studies have reported that RSV alters cytokine production either positively or negatively. Upon RSV treatment, the secretion of anti-inflammatory interleukins, IL-4 and IL-10 was enhanced, and that of nitric oxide, interferon-γ, tumor necrosis factor (TNF)-α, IL-1β, IL-6, monocyte chemoattractant protein-1 (MCP-1), and C reactive protein was suppressed [89–92]. The expression of anti- and pro-inflammatory genes in the brains of experimental autoimmune encephalomyelitis (EAE) mice, an MS model, was also observed in a dose-dependent RSV model. The gene expression of arginase 1 and IL-10 was upregulated, whereas that of iNOS and IL-1β was downregulated in EAE mice after RSV treatment [93]. In contrast, RSV treatment has been reported to increase the expression of IL-17 in murine EAE and significantly exacerbate demyelination and inflammation without neuroprotection in the CNS in autoimmune and viral models of MS [94].
Interestingly, IL-10 secretion was significantly higher in cells from the MS group than in the control group (Figure 2A). IL-10 is important in protection against neuroinflammation due to its anti-inflammatory and immunosuppressive effects [95, 96]. Several studies have demonstrated that IL-10 deficiency is a more severe form of neuroinflammatory pathology [97–100]. Our results suggest that high IL-10 levels modulate the immune response in patients with MS and may be important for the intermittence of the disease.
This study had some limitations, including the evaluation of the effects of RSV on other signaling pathways, such as mitochondrial function and LPS-induced secretion of cytokines (in vitro conditions of neuroinflammatory injury).
Overall, our results indicate that RSV downregulates ROS production in non-stimulated and stimulated granulocytes; however, it is not effective in modulating the production of anti- or pro-inflammatory cytokines in the peripheral immune cells of patients with MS. Controlling ROS production is a key target for the inflammatory process, in which oxidative stress precedes the inflammatory response [101]. These findings highlight the potential utility of RSV as a complementary therapeutic option for patients with MS.
In conclusion, owing to its anti-inflammatory and antioxidant actions, we suggest that RSV can be considered an adjunctive therapy for MS. Further investigations are necessary to elucidate the precise activity of RSV in modulating inflammatory processes.
Abbreviations
BBB: | blood-brain barrier |
CNS: | central nervous system |
EAE: | encephalomyelitis |
EDSS: | expanded disability status scale |
HMGB1: | high mobility group box 1 |
IL: | interleukin |
MS: | multiple sclerosis |
NF-κB: | nuclear factor-kappa B |
NOX: | NADPH-oxidase |
PBMNCs: | peripheral blood mononuclear cells |
ROS: | reactive oxygen species |
RSV: | resveratrol |
SIRT1: | sirtuin 1 |
Declarations
Acknowledgments
We would like to thank Editage (www.editage.com.br) for English language editing.
Author contributions
PHVD: Investigation, Data curation, Writing—original draft. RPS: Investigation. PPC: Investigation, Conceptualization. JANM: Investigation, Conceptualization. CMOV: Conceptualization, Validation, Writing—review & editing, Supervision.
Conflicts of interest
The authors declare that they have no conflict of interest.
Ethical approval
All procedures involving human participants performed in this study were in accordance with the ethical standards of the institutional and national research committee, were approved by Dr. Francisco das Chagas Lima and Silva Ethics Committee of Santa Casa Hospital of Belo Horizonte, Brazil (approval number 69385917.7.0000.5138), and complied with the 1964 Helsinki Declaration and its later amendments or comparable ethical standards.
Consent to participate
The informed consent to participate in the study was obtained from all participants.
Consent to publication
Not applicable.
Availability of data and materials
Some datasets generated and analyzed in this study are not publicly available but are available from the corresponding author upon reasonable request.
Funding
This study was supported by CAPES (Coordenação de Aperfeiçoamento de Pessoal de Nível Superio), CNPq (Conselho Nacional de Desenvolvimento Científico) and FAPEMIG (Fundação de Amparo à Pesquisa do Estado de Minas Gerais). The funders had no role in study design, data collection and analysis, decision to publish, or preparation of the manuscript.
Copyright
© The Author(s) 2024.