Abstract
Myasthenia gravis (MG) is a rare auto-immune neuromuscular junction (NMJ) disorder which is caused by formation of autoantibodies and destruction of NMJ components. The MG diagnosis is based on the symptoms, autoantibodies detection and paraclinical tests. Given that MG patients have so many differential diagnosis and various medication responses, choosing an accurate diagnosis and the therapy plan in MG is challenging. According to the studies, there are the immunologic, genetic, microRNAs, gut microbiome, and other established or newly proposed biomarkers for diagnosis and prognosis of MG. More studies are needed to provide better collection of biomarkers in MG patients and evaluate their role in MG pathology.
Keywords
Myasthenia gravis, biomarker, autoimmune disease, neuromuscular junctionIntroduction
Myasthenia gravis (MG) is a rare auto-immune neuromuscular junction (NMJ) disorder that is caused by the formation of autoantibodies and the destruction of NMJ components [1]. MG is the most common NMJ disorder with a prevalence of 150–300 per million population [2].
There are several autoantibodies against NMJ components that are sensitive and specific diagnostic markers in MG: acetylcholine receptor (AChR) antibodies [which are produced in 80% of patients and are from immunoglobulin G1 (IgG1)/IgG3/IgG2/IgG4 subclass], muscle-specific kinase (MuSK) antibodies (which are produced in 7–10% of patients and are mainly from IgG4 subclass), and lipoprotein-receptor related protein 4 (LRP4) antibodies (mostly are from IgG1/IgG2 subclass) [3]. Agrin is a presynaptic protein secretion from NMJ, which activates the agrin/LRP4/MuSK/downstream of kinase 7 (Dok-7) signaling pathway. These pathways lead to the clustering of AChR on the postsynaptic membrane [4]. Destruction of each part of this pathway by autoantibodies could impair neuromuscular transmission. These pathologic mechanisms are seen as a decremental response during repetitive nerve stimulation (RNS) in MG patients. Although serum autoantibody detection is the most specific diagnostic tool for MG diagnosis, electromyography, RNS, and clinical response to cholinesterase inhibitors are useful in patients who are suspected to have other NMJ disorders. Currently, the treatment of MG is based on cholinesterase inhibitors, corticosteroids, biological medications (belimumab, rituximab, etc.), thymectomy in some patients, plasmapheresis, and Igs [5]. The role of genetic predisposing factors, consuming some medications [statins, penicillamine, interferons (IFNs), etc.], cytokines production, T-helper 1 (Th1)/Th2 ratio alternation, B cell activation, and thymic hyperplasia are the underline possible mechanisms and triggers in MG [6].
The main MG clinical manifestations are fluctuations of muscle weakness in ocular muscles (which is presented by double vision and ptosis), bulbar muscles involvement (in patients who have dysarthria, dysphagia, and facial or jaw muscle weakness), axial muscles weakness (which causes neck flexion impairment and head) and respiratory muscles involvement. Within two years after the ocular onset of MG, up to 80% of patients will develop generalized symptoms. Alongside paraneoplastic disorders associated with thymomas such as myositis, Morvan syndrome, and pure red aplasia, MG is the most common paraneoplastic disorder in thymoma [6, 7].
MG occurs in all ages [< 50 years is early-onset MG (EOMG) and > 50 years is late-onset MG (LOMG)], even children. The symptoms of MG are sometimes unspecific for the diagnosis and some patients are seronegative which means they do not have serum auto-antibodies specific to MG. Early diagnosis of MG, prevention of progression to generalized MG, predicting the response to the treatments, and distinguishing MG from other diseases associated with muscle weakness (as well as other NMJ diseases) are challenging issues. This is why biomarkers are promising tools to help us diagnose and find the best treatment plan. Here, the known biomarkers in MG disease are described.
Immunologic biomarkers of MG
Detection of the autoantibodies against every five subunits of muscle AChR by laboratory tests is the first step of MG diagnosis [1, 8]. The AChR antibodies mostly are from IgG1 or IgG3 subclasses and radioimmunoprecipitation assay (RIPA) is a widely used method to detect them [with a specificity of 99%, a sensitivity of 85% in generalized MG, and 50% in ocular MG (OMG)] [9, 10]. Although the titer of AChR antibodies does not relate to MG severity (unlike MuSK antibodies [11]), some studies evaluate that there is a correlation between the titer of the IgG1 subclass and MG severity. Since the AChR antibody was detected up to 2 years before the onset of MG symptoms, it could be used to diagnose MG earlier [12].
The majority of MuSK antibodies are produced against the extracellular domains which are cysteine-rich Ig-like regions [13]. MuSK antibodies are detected by RIPA in 6% of all MG patients, 40% in the AChR antibody-negative patients, and 0.5–12.5% in the AChR antibody-positive (AChR+) MG patients [13]. MuSK antibodies are from the IgG4 subclass which binds to the first Ig-like domain of MuSK and restrains the agrin-induced and agrin-independent AChR clustering pathways through inhibition of interactions between MuSK and collagen Q or LRP4; interestingly, MuSK antibodies do not activate complement cascade [14, 15]. One of the best examples of indicating the role of biomarkers to predict medication response is in the MuSK antibody-positive (MuSK+) MG patients who usually progress high severity of MG, generalized MG, and are prone to adverse effects with pyridostigmine, less usefulness of thymectomy, excellent treatment response to rituximab, and getting more plasma exchange benefits [16–18].
LRP4 is a transmembrane protein, containing several low-density lipoprotein domains. LRP4 acts as a muscle receptor for agrin-induced AChR clustering at the NMJ, by passing the signal to MuSK [19]. The autoantibodies against LRP4 are mostly from the IgG1 subclass and have complement activation features to play a role in MG pathology. As well as MG, LRP4 has been detected in the cerebrospinal fluid of amyotrophic lateral sclerosis patients [20]. Based on the used method and the source of antigen, and the ethnicity of the patients the prevalence of LRP4 antibody-positive (LRP4+) MG samples in MG patients varies from 2% to 45% [1]. LRP4 antibody positivity has been found in 15–20% and 7.5% of MuSK+ MG and AChR+ MG patients, respectively [21, 22].
The other precious prognostic biomarkers are autoantibodies against titin and ryanodine receptor (RyR). Titin is a huge abundant filamentous protein located in the striated muscles which is one of the sarcomere proteins and RyR is a calcium (Ca2+) channel located in the sarcoplasmic reticulum membrane which plays a role in Ca2+ release from the sarcolemma to the cytoplasm to launch excitation-contraction coupling [23, 24]. Among the AChR+ MG patients, 20–40% of them also have a positive test for titin antibodies. This prevalence shifts from 6% in EOMG to 50–80% in non-thymomatous LOMG patients [25]. RyR antibodies are not detected in the EOMG but 40% of LOMG have a positive result for it. Titin and RyR antibodies are worthy biomarkers of having thymoma in EOMG patients since 50–95% of them have a positive test for titin antibodies and 75% for RyR antibodies [26–28]. Therefore, the positivity of titin and RyR antibodies is correlated with having more severe disease and thymoma.
By using RIPA, 14.6% of the MuSK+ MG and 16.4% of the LRP4+ MG patients have also positive results for titin antibodies. Although enzyme-linked immunosorbent assay (ELISA) did not detect any positive result for titin antibody in the seronegative MG (SNMG) patients, RIPA has been found in 13.4% of SNMG patients [29]. So it could be an invaluable biomarker for detecting MG in SNMG patients.
As mentioned before, agrin is a key proteoglycan involved in the signaling cascade activation resulting in AChR clustering on the postsynaptic membrane. Most MG patients who produce antibodies against AChR, MuSK, or LRP4, also have a positive result for agrin antibody and it has been detected in 2–15% of all MG patients who develop mild to severe MG and who have a mild response to medications [22, 30]. Although the prevalence of agrin antibodies in MG patients is low, the lack of agrin antibodies in other neurologic diseases or healthy people is one of the strong reasons to use agrin antibodies as a specific biomarker for MG.
There are some obstacles to interpreting the level of biomarkers in different populations. One of the pieces of evidence for this statement is the evaluation of the antibody production against Kv1.4, a subunit of voltage-gated potassium (K+) channels, which has a function to regulate presynaptic acetylcholine release. In Japanese MG patients, 11% to 18% of patients showed a positive result of antibodies against Kv1.4, and most of them had severe symptoms, myasthenic crises, myocarditis, abnormal electrocardiogram (ECG), and thymoma [31, 32]. In contrast, 17% of Caucasian MG people showed Kv1.4 antibody positive result which was mostly found in females, LOMG, patients with mild symptoms, and OMG [33].
To find out whether a biomarker is suitable to be used to identify patients or not, one of the important factors is its specificity and sensitivity. Rapsyn is an intracellular scaffold protein located in the muscle cells, which connects the AChR to the cytoskeleton inside the cell. Although 15% of MG patients had Rapsyn antibodies in their serum, they could be present in the serum of patients with other diseases [34–36]. Similar to this, the antibody against cortactin is found in 23.7% of SNMG patients, 9.5% of seropositive MG, 12.5% of patients with other autoimmune diseases, 7.6–26% of skin disorders (polymyositis, dermatomyositis, and immune-mediated necrotizing myopathy), and 5.2% of healthy controls [37–40]. Cortactin is one of the NMJ proteins located in the cytoplasm which has a role in actin assembly and AChR clustering. The detection of cortactin autoantibodies in other patients and healthy controls diminish its role as a specific MG biomarker.
Some studies evaluated the presence of antibodies against collagen Q, an extracellular matrix protein at the NMJ which has interactions with MuSK, collagen XIII, and acetylcholinesterase in the serum of MG patients. These antibodies had no significant value for being MG biomarkers and also they have been detected in other disorders [41–44].
In addition to autoantibodies, subgroups of lymphocytes in the blood and thymus of MG patients could be interesting biomarkers. Follicular regulatory T (Tfr) cells are a subset of regulatory T cells (Tregs) that inhibit the high activation of follicular helper T (Tfh) cells and B cells in germinal centers. The Tfr/Tfh ratio is negatively related to the severity of MG. The depletion of CD4+ C-X-C chemokine receptor type 5 (CXCR5)+ forkhead box P3 (FOXP3)+ Tfr-like cells and an increased number of CD4+CXCR5+FOXP3− Tfh-like cells are seen in MG patients. This means that B cells and T cell activation control are disrupted by Treg cell imbalance in MG [45].
Th1-like Th17 [Th1/17; IFN-γ+ interleukin-17 (IL-17)+ CD4+CD3+] cells are pro-inflammatory trigger cells of Th17 group cells which are increased in the AChR+ MG patients and are decreased faster than Th17 cells in the good responder’s immunosuppression treatment as in the non-responders [46].
MG patients had significantly fewer granulocyte-monocyte colony-stimulating factor (GM-CSF)-expressing cells or GM-CSF-expressing Th cells and ThCD103 than controls in the blood and these cells are also collected in the thymus of the AChR+ MG patients. These subgroups of T cells could be potential biomarkers for the disease severity of MG patients since they are correlated inversely with the MG severity [47]. This study also suggests the tumor necrosis factor (TNF)-producing ThCD103 cell subset could be a valuable promising candidate as a biomarker of MG severity.
The surface lymphocyte molecules are another biomarker in MG. Inducible costimulator (ICOS)/ICOS ligand (ICOSL) are expressed on the activated or memory T cells and in the primary and secondary follicles of lymph nodes, respectively. These markers are responsible for the conversion of types of antibodies and B cell activation [48]. Programmed death protein-1 (PD-1)/PD-1 ligand (PD-L1) are inhibitors of B cell proliferation which are expressed on the surface of CD4+/CD8+ T cells, B cells, dendritic cells (DCs), activated T cells, and non-hematopoietic cells [49]. The PD-1/PD-L1 suppression and ICOS/ICOSL activation lead to inflammatory pathology in autoimmune diseases like MG [50]. This means that these molecules are involved in MG pathology and could be used as therapeutic/diagnostic markers. In addition to addressing T cell surface molecules, we take a glance at the B cell surface molecules. T cell Ig and mucin domain-1 (Tim-1) is expressed on the B cells and has a role in keeping immune tolerance. Tim-1 is down-regulated in B cells in MG patients and inversely correlates with MG severity [51]. Another study measured the CD72 expression on the B cells in MG, multiple sclerosis (MS), and healthy controls [52]. CD72 showed low expression in MG and MS patients. Intercellular adhesion molecule 1 (ICAM-1) and CD25 were introduced as new biomarkers in MG patients but in the same study, soluble forms of CD28, CD80, CD86, and CD152 were not significantly different in MG vs. controls [53].
ILs are renowned biomarkers that let us diagnose and foresee the prognosis of disease precisely. The increased levels of IL-17A, IFN-γ, and IL-21 are mainly the feature of MuSK+ MG, and IL-17A, IL-21, IL-4, and IL-10 are increased in AChR+ MG. After immunosuppressive therapy, IL-10 increases but IFN-γ decreases in AChR+ MG patients [54, 55]. In addition, another more recent study showed that in the generalized AChR+ MG patients, IL-4, IL-5, IL-2, and IL-12-P70 increased [56]. IL-6 is associated with MG severity in AChR+ MG patients and the use of anti-IL-6 therapy, like tocilizumab, causes a decreased level of IL-6 [57]. IL-33, proliferation-inducing ligand, IL-19, IL-20, IL-28A, IL-35, IL-27, and IL-36γ are other novel biomarkers that are increased in MG patients [58–60]. In addition, other inflammatory proteins increase in MG and could be used as biomarkers alongside other specific MG biomarkers: metalloproteinase 10, transforming growth factor-α (TGFα), S100 Ca2+-binding protein A12 (S100A12), IL-6, IL-8, C-C motif ligand 19, and C-X-C motif ligand 1 [61].
Free light chains (FLC) of autoantibodies, were introduced as novel biomarkers in MG. Recently, an interesting study showed that κ FLC, but not λ FLC, increases in the SNMG and OMG. The authors concluded the κ/λ ratio could be used as a novel biomarker for MG patients who do not have another condition associated with FLC alternation [62].
The summary of unveiled novel immunologic biomarkers of MG is illustrated in Figure 1 and listed in Table 1.
The immunologic biomarkers of MG
Auto-antibodies | Immune cells and cytokines | Molecules |
---|---|---|
AChR antibodies | Th1/17 | PD-1/PD-L1 suppression |
LRP4 antibodies | Tfr/Tfh ratio | ICOS/ICOSL activation |
MuSK antibodies | CD4+CXCR5+FOXP3+ Tfr-like | Metalloproteinase 10 |
FLC of autoantibodies | CD4+CXCR5+FOXP3− Tfh-like | TGFα |
Collagen Q antibodies | GM-CSF-expressing Th | S100A12 |
Collagen XIII antibodies | TNF-producing Th CD103 | C-C motif ligand 19 |
Acetylcholinesterase antibodies | IL-17A, IL-21, IL-10, IL-14, IL-2, IL-5, IL-12, IL-P70, IL-33, IL-6, IL-8, IL-19, IL-20, IL-28A, IL-35, IL-27, IL-36γ | C-X-C motif ligand 1 |
Rapsyn antibodies | IFN-γ | ICAM-1 |
Cortactin antibodies | - | CD25 and CD72 |
Titin antibodies | - | Tim-1 |
Kv1.4 antibodies | - | - |
Agrin antibodies | - | - |
RyR antibodies | - | - |
-: blank cells
Genetic factors in MG
The role of genetic factors associated with MG is influenced by population study. Cytotoxic T-lymphocyte antigen-4 (CTLA-4) is expressed on the immune cells including T cells and B cells and represses inflammation. In the Chinese cohort, MG patients showed higher rs733618*C allele frequency of this gene than healthy controls. In addition, in OMG rs231775*A allele frequency was lower than in controls and generalized MG. The rs3087243*A allele also was less frequent in OMGs than in generalized MG. The combination of rs733618*C, rs231775*G, and rs3087243*G alleles increased the risk of MG and OMG type in this cohort [63]. In another study in China, CTLA-4 methylation and CTLA-4 expression in the peripheral blood of MG patients were higher and lower than in controls, respectively and these results were the possible reasons for related cytokines secretion in MG [64].
Genetic factors could be used as prognosis factors in thymoma-associated MG (TAMG). CXCR4, is expressed highly in cancerous tissues, unlike healthy ones [65]. The overexpression of CXCR4 is an independent biomarker of TAMG’s poor prognosis and low survival [66].
LOMG is a major subgroup of MG and its prevalence is growing. A meta-analysis concluded human leukocyte antigen DRB1 (HLA-DRB1) is the most related genotype with LOMG. Increased risk of LOMG was related to DRB1 07 and 0403 alleles. DRB1*0301 and 1301 alleles were introduced as protective factors [67]. Another study measured DQA1*0103 with lower frequency in the OMG group compared to the control group. The MG patients with thyroid-associated ophthalmopathy had higher DQA1*0301 and lower DQB1*0601 frequency. Also, DQB1*0501 was more found in the OMG and OMG+ thyroid-associated ophthalmopathy compared to the control group [66].
The analysis of 598,375 single nucleotide polymorphisms (SNPs) in MG and normal controls, suggested three genes have value to be as MG biomarkers: Ca2+ voltage-gated channel subunit alpha1 S (CACNA1S), signaling lymphocytic activation molecule family 1 (SLAMF1), and RyR [68].
Recently, genome-wide and transcriptome-wide association studies on 1,873 AChR+ MG patients and 36,370 healthy controls were done [69]. This study proved there is a genetic overlap between MG and other autoimmune diseases such as thyroid-related diseases, rheumatoid arthritis, and MS. Cholinergic receptor nicotinic alpha 1 (CHRNA1) and cholinergic receptor nicotinic beta 1 (CHRNB1) signals unlike epsilon and delta subunits, were related with MG since they are targets of AChR antibodies. In this evaluation, MG-associated signals were found on chromosomes 2q31.1, 10p14, and 11q2, and the major histocompatibility complex locus was also forcefully associated with an increased risk of LOMG. In addition, the genes of protein tyrosine phosphatase non-receptor type 22 protein (PTPN22), TNF receptor superfamily member 11a protein (TNFRSF11A), and HLA-DQA1/HLA-B showed MG-associated signals. A genome-wide association study including 1,401 MG patients and 3,508 healthy controls, also showed an association between HLA-DRB1/HLA-B and TNFRSF11A gene [70]. Like the previously discussed study, autoimmune diseases like type 1 diabetes, rheumatoid arthritis, late-onset vitiligo, and autoimmune thyroid disease were related to MG in this study. Interestingly, they also suggested that the agrin gene (AGRN), is a novel MG susceptibility gene. A list of genetic factors associated with MG is shown in Table 2.
Genetic factors associated with MG
The association | Study |
---|---|
CTLA-4 gene: rs733618*C allele frequency was higher in MG than controls, rs231775*A allele frequency was lower in OMG than controls and generalized MG, rs3087243*A allele frequency was lower in OMG than generalized MG. The combination of rs733618*C, rs231775*G, and rs3087243*G alleles was more frequent in MG and OMG than in other patients. | Cai et al. [63] |
CTLA-4 methylation and CTLA-4 expression were higher in MG than in controls. | Fang et al. [64] |
Overexpression of CXCR4 in poor prognosis TAMG. | Yang et al. [66] |
DRB1 07 and 0403 alleles as risk factors in LOMG, DRB1*0301 and 1301 alleles as protective factors in LOMG. | Ling et al. [67] |
Lower DQA1*0103 frequency in OMG than in the control group. Higher DQA1*0301 and lower DQB1*0601 frequency in MG patients with thyroid-associated ophthalmopathy. Higher DQB1*0501 frequency in the OMG and OMG+ thyroid-associated ophthalmopathy than in the control group. | Yang et al. [66] |
RyR, CACNA1S, and SLAMF1 as MG biomarkers. | Na et al. [68] |
CHRNA1, CHRNB1, chromosomes 2q31.1, 10p14, and 11q2, PTPN22, TNFRSF11A, and HLA-DQA1/HLA-B were associated with MG. | Chia et al. [69] |
HLA-DRB1/HLA-B, TNFRSF11A, and AGRN were associated with MG. | Topaloudi et al. [70] |
MicroRNAs as MG biomarker
MicroRNAs (miRNAs) are small and non-coding endogenous RNA molecules that regulate gene expression via various mechanisms [71]. Recently, researchers evaluated the role of miRNAs as valuable biomarkers for detecting diseases or their complications and forecasting medication response. In addition, miRNAs were found in the extra-cellular space, blood plasma, serum, amniotic fluid, cerebrospinal fluid, peritoneal/pleural fluids, breast milk, urine, and tear called circulating miRNAs [72].
Although the comparison of gene expression profiles between MG subgroups has revealed many differences in the expression of miRNAs (Table 3), we should evaluate which ones are more specific for detecting subgroups of MG or medication response. Quantitative reverse transcription polymerase chain reaction (PCR) is a worthy and widely used method to determine miRNA profiles in the samples.
miRNA markers for MG diagnosis
EOMG | TAMG | MuSK+ MG | AChR+ MG | LOMG |
---|---|---|---|---|
Down-regulation:miR-7-5p, miR-29, miR-548k, miR-145, miR-24, and miR-143, miR-146 | Up-regulation:let-7a-5p, let-7f-5p, miR-125a-5p | Down-regulation:miR-210-3p, miR-324-3p | Down-regulation:miR-15b, miR-122, miR-140-3p, miR-185, miR-192, miR-20b, and miR-885-5p, miR-27a-3p | Up-regulation:miR-30e-5p, miR-150-5p, miR-21-5p, miR-106b-3p, miR-223-5p, miR-140-5p, miR-19b-3p |
Up-regulation:miR-150-5p, miR-21-5p | Up-regulation:let-7a-5p, let-7f-5p, miR-125a-5p | Up-regulation:miR-151a-3p, let-7f-5p, let-7a-5p, miR-423-5p | Up-regulation:miR-150-5p and miR-21-5p | - |
Up-regulation in PBMCs:miR-612, miR-3654, miR-3651, and precursor miR-3651 | - | - | - | - |
PMBC: peripheral blood mononuclear blood cell; -: blank cells
It has been shown that miR-150-5p is the most up-regulated one in the serum of AChR+ MG patients and it will be reduced after thymectomy or immunosuppressive treatment [73]. The functions of miR-150-5p are regulation of proliferation, apoptosis, and differentiation of natural killer (NK) cells, T, and B cells [74]. miR-21-5p up-regulates in AChR+ MG patients and decreases after immunosuppressive therapy. Other immunologic disorders such as systemic lupus erythematosus, MS, and type1 diabetes mellitus also deregulate miR-21 [75, 76]. These two miRNAs are modulators for Treg maturation [77]. AChR+ MG has different clinical phenotypes: EOMG, LOMG, and TAMG. Some reports investigated the miRNAs profile in these subgroups.
MuSK+ MG patients have different miRNA profiles. Among them, miR-let-7 through stimulation of the Toll-like receptor 7 and thereby activation of T cells lead to MG in MuSK+ patients [78]. Other circulating RNAs were detected in MG patients. For example, peripheral blood hsa-circRNA5333-4 could be a promising biomarker for the early detection of MG [79]. Long non-coding RNAs (lncRNAs) are other circulating RNAs with more than 200 nucleotides. XLOC_003810, SNHG16, IFNG-AS1, and MALAT-1 are lncRNAs that were found in the serum of MG patients and were associated with T cell activation and PD-1/PD-L1 signaling suppression [80]. Five lncRNAs (NR_104677.1, ENST00000583253.1, NR_046098.1, NR_022008.1, and ENST00000581362.1) were significantly elevated in the serum exosome of MG patients [81].
The advantages of using circulating RNAs are their resistance to changes in temperature and PH changes because of encapsulation by a membrane [82]. The easy identification of miRNAs in the body fluids is a good tool for the early detection and subtyping of MG. In addition, the medication response in MG patients could be monitored by using circulating RNAs.
Gut microbiomes and biomarkers of MG
The human gut microbiome is a huge ecological community of microorganisms in the intestine which makes an important role in our health. Dysbiosis defines by changes in the composition of microbiota that could affect the host/microbe interaction which could increase disease susceptibility [83].
Like many autoimmune diseases, MG is associated with dysbiosis by decreasing the intestinal FOXP3+CD4+ Treg cells. FOXP3+CD4+ Treg cells inhibit B cell production and AChR antibody synthesis. Analysis of the fecal metabolites showed that some bacteria increase in MG: Bacteroidaceae, Lachnospiraceae, Prevotellaceae, and Veillonellaceae, while these bacteria population decrease in MG: Lachnospiraceae, Ruminococcaceae, Erysipelotrichaceae, Clostridiaceae, and Peptostreptococcaceae [84, 85]. MG subjects had significant depletion of Clostridium and Lactobacillus populations. Several studies confirmed that Bacteroidetes and Actinobacteria are increased in MG patients. The Firmicutes/Bacteroidetes (F/B) ratio is the indicator of a pro-inflammatory environment and is lower in MG patients [86].
The significant reduction in the Clostridium spp. maybe has a role in the pathology of MG. The Clostridium spp. affects the intestinal epithelium through up-regulation of 2, 3-dioxygenase and TGFβ-1 which are necessary to FOXP3+CD4+ Treg cells differentiation and restrain AChR antibody proliferation [87, 88].
The analysis of the fecal metabolites of MG patients shows short-chain fatty acids (SCFAs) are decreased compared to healthy controls. SCFAs play a role in the FOXP3+CD4+ Treg cell differentiation. Because the main source of SCFAs production is Clostridium spp. and these bacteria are depleted in MG patients, thereby it could be the second mechanism of MG pathology [89].
Streptococcus salivarius is another bacterium that increases significantly in MG patients. Streptococcus salivarius inhibits the transcriptional activity of peroxisome proliferator-activated receptor γ (PPAR γ). PPAR γ inhibition leads to FOXP3+CD4+ Treg cells decrease and antibody production [90].
The serum levels of lipopolysaccharide secretion soluble CD14 (LPS-sCD14) and endotoxin core antibody IgM (Endo-CAb-IgM) are biomarkers of the translocated microbe and alongside IL-6, secretory IgA (SIgA) and TNF-α were used to determine chronic inflammation from the inflated gut in MG. LPS-sCD14 and Endo-CAb-IgM are decreased but IL-6, SIgA, and TNF-α are increased in the MG patient’s serum. Unlike other autoimmune diseases such as inflammatory bowel disease (IBD)/irritable bowel syndrome (IBS), in which the level of LPS-sCD14 and Endo-CAb-IgM is the reason for chronic inflammation, it seems that this is not the mechanism of inflammation in MG [85, 86].
The gene expression changes by the gut microbiome and pathways which lead to MG pathology are illustrated in Figure 2. There is still a need for studies on the microbiome changes in MG subgroups and treatment response.
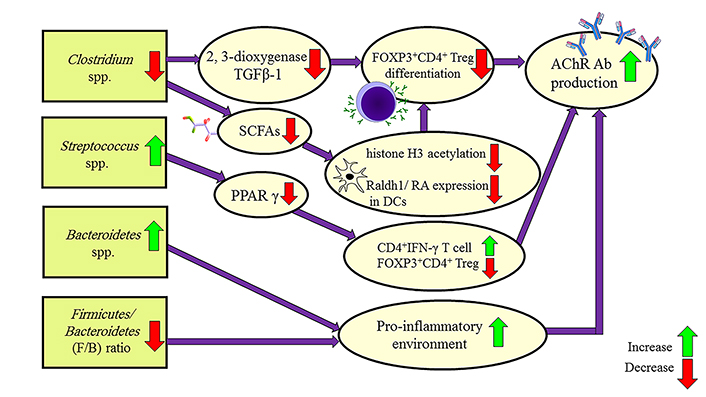
Mechanisms leading to MG pathology by the gut microbiome of MG patients. Raldh1: retinal dehydrogenase isoform-1; RA: retinoic acid; Ab: antibody
Other promising biomarkers
As mentioned before, the dysbiosis of gut microbiota in MG patients plays a crucial role in MG pathology. Calprotectin (CLP) is a Ca2+-binding protein of the S100 family secreted by various immune cells and interacts with Toll-like receptor 4 resulting in inflammatory processes activation [91]. Since CLP previously has been shown to have a relation with dysbiosis in inflammatory diseases, a high level in MG patients exhibits this relation [92, 93].
Some studies focused on the metabolite profile in MG patients. One study demonstrated 12 specific metabolites for MG: two short-chain keto acids, two hydroxy acids, one benzenoid, five structural lipids, one organooxygen compound, and one bile acid. In this study, there were common biomarkers in MS and rheumatoid arthritis with MG [94]. Also, aspartic acid and glutamic acid show elevated levels in MG patients [95].
The receptor for advanced glycation end products (RAGE) is a membranous receptor on the various immune cells which connects to several ligands [like high-mobility group box 1 (HMGB1) and S100 family members] and proceeds inflammatory pathways resulting in impaired self-tolerance. RAGE and S100B both up-regulated in the experimental model of MG resulting in increased Th1/Th17 and decreased FOXP3+ Tregs. AChR antibody production also is the other effect of high RAGE/S100B expression [96]. On the other hand, in the clinical studies, S100B had no significant different level in the MG patients but soluble form of RAGE (sRAGE), and endogenous secretory RAGE were reduced in MG [96, 97]. These forms of RAGE act as an inhibitor for RAGE and ligand interactions. Although there is a contrast between experimental MG and human MG studies, some studies demonstrated S100B increases in the peak of clinical MG presentation in rats and decreases in humans after the remission phase [97, 98]. However, HMGB1 is correlated with AChR antibody production, generalized MG, and thymoma in a study [99], but it had no significant different level in MG vs. controls in the other studies [97, 100]. Studies suggest maybe S100A12 is the responsible ligand for RAGE in MG in the human body [96].
Adipokines are a group of secreted peptides from adipose tissue which have a role in appetite and satiety regulation, lipid metabolism, and insulin sensitivity [101]. Leptin, resistin, and adiponectin are evaluated biomarkers in MG patients. Leptin increased in MG patients due to glucocorticoid use, resistin increased in one study but decreased in another study, and adiponectin showed no significant different level in MG patients vs. controls [102, 103]. However adipokines are utile biomarkers to demonstrate the insulin sensitivity and metabolic status of MG patients, but they could be very changeful by various conditions and medications.
The urokinase plasminogen activator receptor is a fibrinolysis factor that acts by binding to a urokinase-type plasminogen activator. Recently, it has been shown that soluble urokinase plasminogen activator receptor (suPAR) increases systematic chronic inflammation [104]. In the MG patients, suPAR was correlated with disease severity and could be a potential biomarker to precipitate MG clinical course in the patients, but not detecting them from healthy ones, since there was no difference in suPAR level between MG and controls [105].
Mitochondrial proteins as energy expenditure markers could be helpful in MG diagnosis. Mitofusin 1, and 2, optic atrophy type 1, dynamin-related protein 1 and fission 1, adenosine monophosphate (AMP)-activated protein kinase, PPAR γ co-activator-1α, nuclear respiratory factor-1, and mitochondrial transcription factor A are reduced in MG patients [106]. Low plasma vitronectin level is another diagnostic biomarker in MG. Vitronectin is a glycoprotein in blood and the extracellular matrix which induces complement-dependent immune response and clot regulation. The unidentified role of this reduction in MG patients is still unknown [107].
Beyond the diagnosis, subtyping, precipitating medication response, and MG severity classification, we could apply biomarkers as prognostic factors in patients with thymoma. For example, heat shock protein 90α (HSP90α) increases in the serum in non-thymomatous MG, thymic carcinomas, thymomas, and thymic neuroendocrine tumors MG compared to those experienced thymectomy. HSP90α high level was associated with a low recurrence-free rate in patients with thymoma [108].
Conclusions
The use of various biomarkers to diagnose, treat and determine the MG prognosis could differentiate MG from its differential diagnoses and make the treatment challenges of these patients easier. Although many studies have been designed to determine new biomarkers, there are many challenges to achieving the profile of MG biomarkers. The existing limitations are the method of measuring biomarkers, the ethnicity under investigation, the time of measurement (before or after treatment), and unknown confounding factors which alter the levels of these molecules.
In this study, we gave a brief overview of immunological biomarkers, genetic biomarkers, RNAs, and other new biomarkers in MG. More studies are needed to determine the exact role of these biomarkers in the pathology of MG disease and the profile of biomarkers in different subgroups of MG, and investigate the biomarker changes after applying therapeutic plans.
Abbreviations
AChR: | acetylcholine receptor |
AChR+: | acetylcholine receptor antibody-positive |
Ca2+: | calcium |
CTLA-4: | cytotoxic T-lymphocyte antigen-4 |
CXCR5: | C-X-C chemokine receptor type 5 |
Endo-CAb-IgM: | endotoxin core antibody immunoglobulin M |
EOMG: | early-onset myasthenia gravis |
FLC: | free light chains |
FOXP3: | forkhead box P3 |
GM-CSF: | granulocyte-monocyte colony-stimulating factor |
HLA: | human leukocyte antigen |
HLA-DRB1: | human leukocyte antigen DRB1 |
ICOS: | inducible costimulator |
ICOSL: | inducible costimulator ligand |
IFNs: | interferons |
IgG1: | immunoglobulin G1 |
IL-17: | interleukin-17 |
lncRNAs: | long non-coding RNAs |
LOMG: | late-onset myasthenia gravis |
LPS-sCD14: | lipopolysaccharide secretion soluble CD14 |
LRP4: | lipoprotein-receptor related protein 4 |
MG: | myasthenia gravis |
miRNA: | microRNA |
MS: | multiple sclerosis |
MuSK: | muscle-specific kinase |
MuSK+: | muscle-specific kinase antibody-positive |
NMJ: | neuromuscular junction |
OMG: | ocular myasthenia gravis |
PD-1: | programmed death protein-1 |
PD-L1: | programmed death protein-1 ligand |
PPAR γ: | peroxisome proliferator-activated receptor γ |
RAGE: | receptor for advanced glycation end products |
RIPA: | radioimmunoprecipitation assay |
RyR: | ryanodine receptor |
S100A12: | S100 calcium-binding protein A12 |
SCFAs: | short-chain fatty acids |
SNMG: | seronegative myasthenia gravis |
suPAR: | soluble urokinase plasminogen activator receptor |
TAMG: | thymoma-associated myasthenia gravis |
Tfh: | follicular helper T |
Th1: | T-helper 1 |
Tim-1: | T cell immunoglobulin and mucin domain-1 |
TNF: | tumor necrosis factor |
TNFRSF11A: | tumor necrosis factor receptor superfamily member 11a protein |
Treg: | regulatory T cells |
Declarations
Author contributions
FA: Conceptualization, Writing—immunologic, gut microbiome, and other biomarkers section. RR: Conceptualization, Writing—genetic factors and miRNA biomarkers section. Both of the authors contributed to manuscript revision, read and approved the submitted version.
Conflicts of interest
The authors declare that they have no conflicts of interest.
Ethical approval
Not applicable.
Consent to participate
Not applicable.
Consent to publication
Not applicable.
Availability of data and materials
Not applicable.
Funding
Not applicable.
Copyright
© The Author(s) 2022.