Abstract
Acute ischemic stroke (AIS) is the leading cause of disability and one of the top causes of mortality worldwide. The current standard of care is reperfusion therapy including intravenous thrombolysis (IVT) and thrombectomy. However, these treatments have limitations as they have a limited therapeutic window. Hence, there is a vital need to develop neuroprotective agents to prevent brain injury, extend the reperfusion window, improve mortality, and reduce disability in AIS patients. Neuroprotective agents work by counteracting the detrimental biochemical and molecular events that result in irreversible ischemic damage. Numerous preclinical studies and clinical trials have been done on different agents. Thus far, all have been definitively unsuccessful in large trials. Currently, there are several challenges in translation from animal studies to human trials. It is important to understand the current evidence as well as past challenges in the development of neuroprotective strategies in AIS in order for a more strategic selection of agents to be studied, improve study designs and thus contribute to the development of effective neuroprotective agents. Newer agents have shown promise in neuroprotection, and human trials are ongoing. In this review, the mechanisms of action of different families of neuroprotective agents were discussed. The evidence for the efficacy of different drugs in each family of neuroprotective agents was also evaluated and the current research landscape in neuroprotection for AIS was summarized. The past challenges and limitations in clinical trials and proposed possible ways to address these issues were highlighted.
Keywords
Neuroprotection, acute ischemic stroke, neuron death, therapeutic, mechanismsIntroduction
Acute ischemic stroke (AIS) occurs as a consequence of vascular occlusion due to a thrombotic or embolic event which decreases perfusion to the brain. The current standard of care includes intravenous thrombolysis (IVT) and endovascular therapy (EVT) [1]. These treatments aim to restore blood supply to ischemic regions of the brain, thus halting the effects of the ischemic cascade and downstream cellular damage. Despite the advancements in reperfusion strategies, stroke remained the second leading cause of death and the third leading cause of combined death and disability globally in 2019 [2]. Neuroprotective therapy aims to counteract the detrimental biochemical and molecular events in the ischemic cascade which can inflict further insult and result in irreversible ischemic damage and post-ischemic inflammation [3]. Examples of neuroprotective therapy being studied include pharmacological compounds and non-pharmacological methods such as hypothermia, normobaric oxygen, or remote ischemic preconditioning [4]. Therefore, neuroprotective therapy may potentially reduce reperfusion injury, extend the therapeutic window and improve the outcomes of reperfusion therapy [4–6].
Over the past few decades, neuroprotective therapy has yielded positive results in experimental models and animal trials but has been unsuccessful in human trials [7]. Several reasons have been postulated to explain this. Firstly, the majority of animal studies were conducted in young animals and may fail to account for significant comorbidities present in older humans who experience AIS. Furthermore, many human clinical trials did not manage to administer neuroprotective therapy within the hyperacute phase of stroke, when neuroprotection is more effective [5]. However, a recent promising large randomized clinical trial, the efficacy and safety of nerinetide (NA-1) for the treatment of AIS (ESCAPE-NA1) trial conducted using NA-1 has renewed interest in the use of neuroprotective agents in AIS, as the non-tissue plasminogen activator (TPA) arm has shown positive results [8]. Hence, a new trial [efficacy and safety of NA-1 in participants with AIS undergoing endovascular thrombectomy excluding thrombolysis (ESCAPE-NEXT), NCT04462536] is being done to investigate the benefits of NA-1 in this subgroup of patients. Other newer agents investigated have also shown much promise. This review will summarise the evidence from human clinical trials for different families of neuroprotective agents in AIS, such as N-methyl-D-aspartate (NMDA) antagonists, free radical scavengers, calcium channel blockers, immune system modulators, antioxidants, and hypoglycemic agents.
Ischemic cascade in AIS
AIS leads to cerebral ischemia and depletion of energy stores, triggering multiple complex downstream pathways to result in acute neuronal injury and death (Figure 1). Due to lack of perfusion to brain tissue, ion pumps and channels fail and excitatory neurotransmitters reach toxic levels, resulting in damage and death of the neurovascular unit, which includes neurons, microglia, astrocytes, and endothelia. In particular, excessive release of glutamate causes over activation of NMDA and other ionotropic [α-amino-3- hydroxy-5-methyl-4-isoxazolepropionic acid (AMPA) and kainite] glutamatergic receptors [3]. Uncontrolled calcium entry through these receptors results in free radical generation and damage. Damage-associated molecular patterns (DAMPs) are released after neuron damage and cause amplification of the inflammatory cascade. Reperfusion following revascularisation therapy also accelerates damage caused by free radicals (oxidative/nitrosative stress injury) [9]. These eventually lead to neuronal death via necrosis, apoptosis, and autophagy. Free radicals may also damage the blood-brain barrier (BBB) and trigger the inflammatory response. Microglia are resident immune cells of the central nervous system (CNS), which release proinflammatory cytokines and generate free radicals, and further recruit peripheral immune cells into the CNS, leading to increasing neuronal damage [10]. Therefore, strategies to target this ischemic cascade may have neuroprotective effects. An overview of neuroprotective agents investigated in human trials for AIS is shown in Table 1 and Table 2.
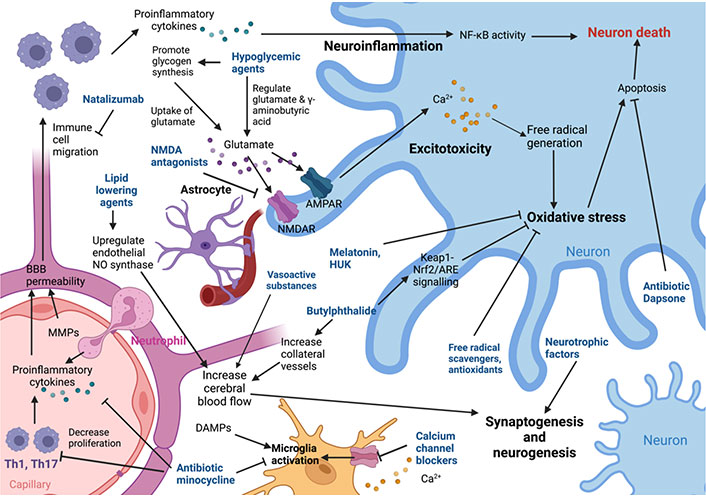
Diagram showing the mechanisms of action of various neuroprotective agents. MMP: matrix metalloproteinase; NMDAR: NMDA receptor; AMPAR: AMPA receptors; HUK: human urinary kallidinogenase; NF-κB: nuclear factor kappa B; Keap1-Nrf2: Kelch-like ECH-associated protein 1-nuclear factor erythroid 2-related factor 2 pathway; ARE: antioxidant response element; Th1: T helper type 1; NO: nitric oxide; Nrf2: nuclear factor erythroid 2-related factor 2. Created with BioRender.com (https://biorender.com/)
Note. Adapted from “Pharmacological brain cytoprotection in acute ischaemic stroke — renewed hope in the reperfusion era,” by Fisher M, Savitz SI. Nat Rev Neurol. 2022;18:193–202 (https://www.nature.com/articles/s41582-021-00605-6). CC BY.
Overview of human trials on neuroprotective agents in AIS
Mechanism | Drug | Trials | Study references | Overall effect |
---|---|---|---|---|
NMDAR antagonist | NA-1 | ESCAPE-NA1 | Hill et al. [8] 2020 | Negative overall, positive in patients without IV thrombolysis |
Memantine | - | Beladi Moghadam et al. [11] 2021 | Positive in a small RCT | |
Aptiganel hydrochloride | - | Albers et al. [12] 2001 | Negative | |
AR-R15896AR | - | Lees et al. [13] 2001, Diener et al. [14] 2002 | Negative | |
Selfotel | ASSIST | Davis et al. [15] 2000 | Negative | |
Lubeluzole | SRMA of 5 trials | Gandolfo et al. [16] 2002 | Negative | |
Gavestinel | - | Lees et al. [17] 2001 | Negative | |
GV150526 | - | Lees et al. [18] 2000, Dyker and Lees [19] 1999 | Negative | |
Licostinel | - | Albers et al. [20] 1999 | Negative | |
Neu2000 | SONIC (ongoing) | Hong et al. [21] 2018 | Ongoing | |
DAMPs antagonist | ZK 200775 | - | Walters et al. [22] 2005 | Negative |
GABA agonist | Clomethiazole | CLASS-I | Lyden et al. [23] 2002 | Negative |
Magnesium | Magnesium | IMAGES, FAST-MAG | IMAGES study investigators [24], Shkirkova [25] 2017, Afshari et al. [26] 2013 | Positive in 1 small RCT, negative in 2 larger RCTs |
Free radical scavengers | Edaravone | ECCS-AIS, EDO, TASTE | Zheng and Chen [27] 2016, Sharma et al. [28] 2011, Enomoto et al. [29] 2019, Mitta et al. [30] 2012, Shinohara et al. [31] 2009, Xu et al. [32] 2021 | Positive |
NXY-059 | SAINT I and II | Diener et al. [33] 2008, Shuaib et al. [34] 2007, Lees et al. [35] 2006 | Negative overall | |
Citicoline | - | Clark et al. [36] 1999, Agarwal et al. [37] 2022, Warach et al. [38] 2000, Dávalos et al. [39] 2002, Kuryata et al. [40] 2021 | Positive | |
Calcium channel blockers | Nimodipine | SRMA of 12 trials, The American Nimodipine Study | The American Nimodipine Study Group [41] 1992 | Negative overall, positive for 120 mg within 18 h in one trial |
Verapamil | SAVER-1 | Fraser et al. [42] 2017 | Safe and feasible, no efficacy data | |
Immune system modulation | Natalizumab | ACTION II | Elkind et al. [43] 2020 | Negative, reduced good outcome |
MAPC | MASTERS | Hess et al. [44] 2017 | Negative overall, positive < 36 h | |
Minocycline | SRMA of 7 small trials | Naderi et al. [45] 2020, Malhotra et al. [46] 2018 | Positive | |
Imatinib | - | Wahlgren et al. [47] 2017 | - | |
Antioxidants | NAC | - | Sabetghadam et al. [48] 2020 | Positive |
co-ultraPEALut | - | Caltagirone et al. [49] 2016 | Positive compared to baseline in an observational study | |
Butylphthalide | BAST | Zhang et al. [50] 2022 | Positive in observational study, ongoing RCT | |
HUK | RESK | Ni et al. [51] 2021 | Positive, not placebo-controlled | |
Melatonin | SRMA of 6 trials | Ramos et al. [52] 2020 | Negative | |
Uric acid | URICO-ICTUS | Chamorro et al. [53] 2014 | Negative | |
Albumin | ALIAS | Ginsberg et al. [54] 2013 | - | |
Hypoglycaemic agents | Insulin | SHINE | Johnston et al. [55] 2019 | Negative comparing intensive vs. standard glucose control |
Glibenclamide | - | Caffes et al. [56] 2015 | Positive in retrospective study | |
Glyburide | GAMES, GAMES-RP | Sheth et al. [57, 58] 2014, 2016 | Negative | |
Others | Statins | SRMA of 22 observational studies, ASSORT | Montaner et al. [59] 2008, Yoshimura et al. [60] 2017, Guo et al. [61] 2021 | Negative in RCT, positive in SRMA of observational studies |
Cerebrolysin | SRMA of 6 trials | Wang et al. [62] 2017 | Negative | |
Piracetam | SRMA of 3 trials | Ricci et al. [63] 2012 | Negative | |
Theophylline | - | Modrau et al. [64] 2020 | Positive on NIHSS | |
GTN | SRMA of 5 trials, MR ASAP trial | Bath et al. [65] 2016, van den Berg et al. [66] 2022 | Negative overall | |
Epo | Epo stroke | Ehrenreich et al. [67] 2009 | Negative | |
DDFPe | - | Culp et al. [68] 2019 | Positive but small sample size | |
Otaplimastat | SAFE-TPA | Kim et al. [69] 2020 | Negative | |
3K3A-activated protein C | RHAPSODY | Lyden et al. [70] 2019 | Possible reduction in hemorrhagic rate | |
Dapsone | - | Nader-Kawachi et al. [71] 2007 | Positive in pilot trial |
IV: intravenous; RCT: randomized controlled trial; ASSIST: acute stroke studies involving selfotel treatment; SRMA: systematic review and meta-analysis; SONIC: safety and optimal neuroprotection of neu2000 in AIS; GABA: gamma-aminobutyric acid; CLASS-I: clomethiazole acute stroke study in ischemic stroke; IMAGES: IV magnesium efficacy in stroke; FAST-MAG: field administration of stroke therapy-magnesium; ECCS-AIS: edaravone-citicoline comparative study in AIS; EDO: edaravone versus sodium ozagrel in acute noncardioembolic ischemic stroke; TASTE: treatment of AIS with edaravone dexborneol; NXY: disodium 2,4-sulphophenyl-N-tert-butylnitrone; SAINT: stroke-acute ischemic NXY treatment trial; SAVER: surgical anterior ventricular restoration; ACTION: safety and efficacy of IV natalizumab in AIS; MAPC: multipotent adult progenitor cells; MASTERS: MAPC in AIS; co-ultraPEALut: co-ultramicronized palmitoylethanolamide/luteolin; NAC: N-acetylcysteine; BAST: butylphthalide for patients who had acute ischaemic stroke receiving IVT or EVT; RESK: re-evaluate the efficacy and safety of HUK; URICO-ICTUS: safety and efficacy of uric acid in patients with acute stroke; ALIAS: High-dose albumin treatment for acute ischaemic stroke; SHINE: the stroke hyperglycemia insulin network effort; GAMES: glyburide advantage in malignant edema and stroke; GAMES-RP: GAMES-remedy pharmaceuticals; ASSORT: administration of statin on AIS patient; NIHSS: National Institutes of Health Stroke Scale; GTN: glyceryl trinitrate; MR ASAP: multicentre randomised trial of acute stroke treatment in the ambulance with a nitroglycerin patch; Epo: erythropoietin; DDFPe: dodecafluoropentane; SAFE-TPA: safety and efficacy of otaplimastat in patients with AIS requiring TPA; RHAPSODY: safety evaluation of 3K3A-APC in ischemic stroke
Characteristics of human trials on neuroprotective agents in AIS
Class | Drug | Study reference | Year | Study type | Study size |
---|---|---|---|---|---|
NMDA antagonists | Nerinetide (ESCAPE-NA1 trial) | Hill et al. [8] | 2020 | RCT | 1,105 |
Nerinetide (ESCAPE-NEXT trial) | Hill et al. [8] | 2020 | RCT | 1,020 | |
Memantine | Beladi Moghadam et al. [11] | 2021 | RCT | 77 | |
Aptiganel hydro-chloride | Albers et al. [12] | 2001 | RCT | 628 | |
AR-R15896AR | Lees et al. [13] | 2001 | RCT | 174 | |
AR-R15896AR | Diener et al. [14] | 2002 | RCT | 103 | |
Selfotel | Davis et al. [15] | 2000 | RCT | 567 | |
Lubeluzole | Gandolfo et al. [16] | 2002 | SRMA | 5 RCTs, 3,510 | |
GV150526 | Lees et al. [17] | 2001 | RCT | 128 | |
Gavestinel | Lees et al. [18] | 2000 | RCT | 1,788 | |
GV150526 | Dyker et al. [19] | 1999 | RCT | 66 | |
Neu2000 (SONIC trial) | Hong et al. [21] | 2018 | RCT | 210 | |
Magnesium | Magnesium | Afshari et al. [26] | 2013 | RCT | 107 |
Magnesium (IMAGES trial) | IMAGES study investigators [24] | 2004 | RCT | 2,589 | |
Magnesium (FAST-MAG pilot trial) | Saver et al. [72] | 2004 | RCT | 20 | |
Magnesium (FAST-MAG trial) | Shkirkova et al. [25] | 2017 | RCT | 1,130 | |
Magnesium | McKee et al. [73] | 2005 | RCT | 30 | |
AMPA antagonist | ZK 200775 | Walters et al. [22] | 2005 | RCT | 61 |
GABA agonist | Clomethiazole (CLASS-I) | Lyden et al. [23] | 2002 | RCT | 1,198 |
Free radical scavenger | Edaravone | Zheng et al. [27] | 2016 | RCT | 65 |
Edaravone | Sharma et al. [28] | 2011 | RCT | 50 | |
Edaravone | Enomoto et al. [29] | 2019 | Retrospective observational study | 11,508 | |
Edaravone vs. citicoline | Mitta et al. [30] | 2012 | RCT | 90 | |
Edaravone | Shinohara et al. [31] | 2009 | RCT | 386 | |
Edaravone dexborneol vs. edaravone | Xu et al. [32] | 2021 | RCT | 1,362 | |
Edaravone | Vdovychenko et al. [74] | 2021 | Prospective study | 48 | |
Edaravone | Naganuma et al. [75] | 2018 | Retrospective observational study | 1,114 | |
Edaravone | Ishibashi et al. [76] | 2013 | Retrospective observational study | 625 | |
NXY-059 (pooled SAINT I and II trials) | Diener et al. [33] | 2008 | RCT | 5,028 | |
NXY-059 (SAINT II trial) | Shuaib et al. [34] | 2007 | RCT | 3,306 | |
NXY-059 (SAINT I trial) | Lees et al. [35] | 2006 | RCT | 1,722 | |
Citicoline | Agarwal et al. [37] | 2022 | RCT | 99 | |
Citicoline | Warach et al. [38] | 2000 | RCT | 81 | |
Citicoline | Dávalos et al. [39] | 2002 | SRMA | 4 RCTS, 1,372 | |
Citicoline | Kuryata et al. [40] | 2021 | RCT | 96 | |
Calcium channel blocker | Nimodipine | Di Mascio [77] | 1994 | SRMA | 12 RCTs, 1,371 |
Nimodipine | The American Nimodipine Study Group [41] | 1992 | RCT | 1,064 | |
Verapamil (SAVER-1 trial) | Fraser et al. [42] | 2017 | RCT | 104 | |
Immune system modulation | Natalizumab (ACTION II trial) | Elkind et al. [43] | 2020 | RCT | 277 |
MAPC (MASTERS trial) | Hess et al. [44] | 2017 | RCT | 129 | |
Minocycline | Naderi et al. [45] | 2020 | Systematic review | 6 RCTs | |
Minocycline | Malhotra et al. [46] | 2018 | SRMA | 7 RCTs, 426 | |
Minocycline | Kohler et al. [78] | 2013 | RCT | 95 | |
Imatinib | Wahlgren et al. [47] | 2017 | RCT | ||
Antioxidant | NAC | Sabetghadam et al. [48] | 2020 | RCT | 68 |
Co-ultramicronized Palmitoylethanolamide/Luteolin | Caltagirone et al. [49] | 2016 | Prospective trial | 250 | |
Butylphthalide | Zhang et al. [50] | 2022 | Retrospective observational study | 127 | |
Butylphthalide (BAST trial) | Zhang et al. [79] | 2021 | RCT | 1,200 | |
HUK (RESK study) | Ni et al. [51] | 2021 | RCT | 1,206 | |
Melatonin | Ramos et al. [52] | 2020 | Systematic review | 6 RCTs | |
Uric acid | Chamorro et al. [53] | 2014 | RCT | 411 | |
Albumin | Ginsberg et al. [54] | 2013 | RCT | 841 | |
Hypoglycaemics | Insulin (SHINE trial) | Johnston et al. [55] | 2019 | RCT | 1,151 |
Glyburide (GAMES) | Sheth et al. [57] | 2014 | Pilot study | 10 | |
Glyburide (GAMES-RP) | Sheth et al. [58] | 2016 | RCT | 86 | |
Lipid lowering medication | Simvastatin | Montaner et al. [59] | 2008 | RCT | 60 |
Statins (ASSORT trial) | Yoshimura et al. [60] | 2017 | RCT | 277 | |
Statins | Guo et al. [61] | 2021 | SRMA | 22 observational studies, 17,554 | |
Low molecular weight peptides from porcine brain | Cerebrolysin | Wang et al. [62] | 2017 | SRMA | 6 RCTs, 1,649 |
Nootropic | Piracetam | Ricci et al. [63] | 2012 | SRMA | 3 RCTs, 1,002 |
Phosphodiesterase inhibitor | Theophylline | Modrau et al. [64] | 2020 | RCT | 64 |
BBB preservation | Epo | Ehrenreich et al. [67] | 2009 | RCT | 522 |
Oxygen transporter | DDFPe | Culp et al. [68] | 2019 | RCT | 24 |
MMP inhibitor | Otaplimastat (SAFE-TPA) | Kim et al. [69] | 2020 | RCT | 69 |
Activated protein C variant | 3K3A-activated protein C | Lyden et al. [70] | 2019 | RCT | 110 |
Apoptosis inhibition | Dapsone | Nader-Kawachi et al. [71] | 2007 | RCT | 30 |
Excitotoxicity glutamate receptor antagonists
Brain ischemia results in the excessive release of neurotransmitters such as glutamate and activation of NMDAR and AMPAR, and blockade of their effects may protect against apoptotic and excitotoxic insults, which may be neuroprotective after AIS.
NA-1
NA-1 is a peptide that interferes with the binding of postsynaptic density protein 95 to the NMDAR and to neuronal NO synthase. This dissociates NMDAR from downstream neurotoxic cascades, which include excessive neuronal calcium influx and neurotoxic NO production. The ESCAPE-NA1 phase III trial investigated the effect of a single IV dose of NA-1 before thrombectomy in patients with ischaemic stroke, with moderate-to-good filling of collateral vessels on computed tomography (CT) angiography and no evidence of an extensive region of early infarction on CT brain. The ESCAPE-NA1 trial [8] showed that NA-1 did not improve the proportion of overall stroke patients achieving good clinical outcomes [modified Rankin score (mRS) 0–2 at 90 days] or incidence of serious adverse events compared with placebo [adjusted risk ratio (RR) 1.04, 95% confidence interval (CI) 0.96–1.14] [8]. However, in a pre-defined subgroup of patients who did not receive IV thrombolysis, NA-1 significantly increased favorable outcomes compared to placebo (59.3% vs. 49.8%). The study proposed that this could be due to enzymatic cleavage of NA-1 by plasmin, a serine protease generated from plasminogen by tissue-plasminogen activators such as alteplase, thus leading to subtherapeutic concentrations of NA-1. This hypothesis was supported by pharmacokinetic data from a subset of trial participants [80]. Therefore, further investigation is needed to identify subgroups that may potentially benefit from NA-1. Currently, there is an ongoing trial (ESCAPE-NEXT, NCT04462536) investigating the efficacy of NA-1 in patients who do not receive thrombolysis.
Memantine
Memantine antagonizes overstimulated NMDAR, and preclinical studies had proposed that memantine improves outcomes through increased brain-derived neurotrophic factor signalling, reduced reactive astrogliosis, and improved vascularization [81]. In a recent human trial involving 77 participants with mild to moderate AIS (NIHSS score 16 and below), patients were given 20 mg memantine every 8 h for five days and then 20 mg daily for three months [11]. Memantine significantly improved the neurologic function of the patients, with NIHSS change after 5 days being significantly higher in the intervention group compared to the control group (−2.96 ± 0.10 in the intervention group and −1.24 ± 0.96 in the control group P < 0.0001). Memantine also significantly increased the Barthel Index (BI) scores after 3 months (6.00 ± 2.62 in the intervention group and 3.96 ± 1.76 in the control group P = 0.002) [11]. Although there is preliminary evidence that memantine may be beneficial, further larger trials are needed to better characterize its effects on AIS patients.
Novel NMDAR antagonists
Novel small molecules that bind and inhibit the NMDAR and NMDA glycine site antagonists generally showed no improvement in stroke outcomes in human RCTs. This included aptiganel hydrochloride [12], AR-R15896AR [13, 14], selfotel [15], and lubeluzole [16]. The glycine site antagonists, gavestinel, GV150526, and licostinel, showed no significant differences in serious side-effects, outcome, or mortality [17–20]. Neu2000, a sulfasalazine derivative, selectively blocks NMDARs and acts as a free radical scavenger. The ongoing SONIC phase-II clinical trial has randomized AIS patients who underwent EVT into control, low-dose (2.75 g), and high-dose (5.25 g) Neu2000KWL over 5 days, and the results are yet to be published [21].
AMPA antagonists
Excessive activation of AMPAR induces the postsynaptic depolarisation of glutamate and is a marker of excitotoxicity-related diseases like AIS [82]. However, in a phase II trial, AMPA antagonist ZK 200775 was found to reversibly worsen the neurological condition in patients with AIS. Furthermore, ZK 200775 exerted significant sedative effects in patients which precluded its further development as a neuroprotective agent [22].
GABA agonist inhibition of excitotoxicity
GABA is the main inhibitory neurotransmitter in the CNS, and it reduces depolarization-induced and ischemia-induced glutamate release. Furthermore, activation of GABA reduces respiratory rate, preserves glucose, and reduces acidosis, improving cerebral blood flow [83]. Clomethiazole is a thiazole derivative that potentiates GABA activity at low concentrations, and at high concentrations, directly activates the GABA receptor by a different binding site. In the CLASS-I trial involving 1,198 patients, it was shown that clomethiazole did not improve the proportion of AIS patients with BI ≥ 60, functional outcome, or neurological recovery [23].
Magnesium
Magnesium may antagonise some NMDARs and has been studied in several clinical trials. Magnesium showed a significant neuroprotective effect in one small RCT of 107 patients [26]. Patients receiving IV magnesium within 12 h of stroke onset showed significant improvements in the NIHSS score and better functional outcomes as measured by the mRS at 90 days compared with placebo [26]. However, two large randomised controlled trials have shown that magnesium has no overall benefit on AIS patients [odds ratio (OR) 0.95, 95% CI 0.80–1.13] [24, 25, 72]. In the IMAGES trial, IV magnesium given within 12 h of acute stroke did not reduce death (hazard ratio 1.18, 95% CI 0.97–1.42) significantly [24]. This study was limited as the mean time to initiate treatment was 7 h, and only 3% of patients were treated in the 3 h window which increases the chances of optimal recovery. However, the FAST-MAG phase III trial that involved 1,700 participants also reported no significant differences with respect to mortality or all serious adverse events in patients treated with IV magnesium within 2 h of stroke onset compared to placebo (15.4% in the magnesium group and 15.5% in the placebo group, P = 0.95) [72]. Magnesium level achieved in the treatment group was not associated with clinical outcomes including disability at 90 days, symptomatic hemorrhage, or death [25]. One reason for the limited effect of IV magnesium in neuroprotection could possibly be related to the fact the brain bioavailability of magnesium sulfate is limited, and the neuroprotective threshold of magnesium in the injured brain is uncertain [73]. Furthermore, as magnesium takes time to travel across the BBB, the concentration in cerebrospinal fluid (CSF) peaks 4 h after IV administration. Hence, magnesium may not have accumulated in brain tissues quickly enough to yield a benefit despite achieving increased serum levels [25].
Reduction of oxidative stress by free radical scavengers
In brain ischemia, excessive calcium entry through neurotransmitter receptors results in a free radical generation. Free radicals exacerbate membrane damage through the peroxidation of unsaturated fatty acids in the cell membrane, protein dysfunction, and deoxyribonucleic acid (DNA) damage, contributing to ischemic brain injury [9].
Edaravone
Edaravone is an amphiphilic low-molecular-weight drug that scavenges both lipid- and water-soluble peroxyl radicals, thus preventing lipid oxidation in animal models [84]. It also inhibits oxidation, increases endothelial NO synthase (eNOS), enhances NO production, and decreases neuronal NO synthase (nNOS) and inducible NO synthase (iNOS), which are detrimental NO synthase (NOS). This may improve cerebral blood flow without peroxynitrite generation during reperfusion [85]. An RCT by Zheng and Chen [27], 2016 that recruited 60 diabetic stroke patients showed that mean NIHSS scores were lower (60%), and BI scores were 1.7-fold higher in edaravone-treated patients compared to control on day 14. Another RCT by Sharma et al. [28] 2011 involving 50 patients also found that edaravone effectively improved functional outcomes (mRS ≤ 2) in patients after AIS. This was also supported by a few retrospective observational studies [29, 30], which reported that the use of edaravone is associated with better functional independence after discharge (adjusted OR, 1.21; 95% CI 1.03–1.41) and lower hospital mortality (adjusted OR, 0.52; 95% CI 0.43–0.62). Although edaravone was the first drug to be approved for clinical treatment of AIS in Japan in 2009 [31], its benefit requires further validation in larger studies.
An RCT showed that edaravone was not inferior to ozagrel sodium (thromboxane A2 synthase inhibitor) for the treatment of acute noncardioembolic ischemic stroke in 401 patients [31], and edaravone could be more cost-effective than ozagrel [86]. Novel neurotherapeutic agents with a combination of edaravone and other active ingredients have also been developed. For instance, edaravone dexborneol, which comprises 2 active ingredients edaravone and (+)-borneol, has shown synergistic effects of antioxidant and anti-inflammatory in animal models. In a phase III RCT of 1,165 patients, the edaravone dexborneol group showed a significantly higher proportion of patients experiencing good functional outcomes on day 90 after randomization, compared with the edaravone group (mRS ≤ 1, OR 1.42, 95% CI 1.12–1.81) [32].
NXY-059
NXY-059 is a nitrone-based IV drug which has a free radical trapping action in animal models [87]. Although NXY-059 was consistently reported to be well-tolerated in AIS patients, NXY-059 did not improve mortality in several RCTs [33–35]. While the administration of NXY-059 within 6 h after the onset of AIS showed a reduction in terms of disability at 90 days, it did not show benefits in reducing the rates of severe and non-severe adverse events including neurologic status in a trial of 1,722 patients [35]. In the SAINT I and II RCTs, where NXY-059 was also administered within 6 h of stroke onset, an intention-to-treat analysis based on 5,028 patients from these trials confirmed an absence of benefit by comparison at each level of the mRS, as well as no difference in mortality in the treatment and control groups [33].
Citicoline
Citicoline may reduce free radical generation through a reversible synthetic pathway leading from choline to phosphatidylcholine, which reduces the breakdown from phosphatidylcholine into free fatty acids that are used to generate free radicals in animal models [36]. In AIS, a few RCTs reported that the administration of citicoline is associated with a significant decrease in infarct volume compared to placebo [37, 38]. In addition to infarct volume, oral administration of citicoline (500 mg/day) for 6 weeks improved NIHSS by seven or more points at week 12 [38]. In a systematic review by Dávalos et al. [39] 2002, oral citicoline within the first 24 h of onset in moderate and severe stroke could increase the chance of full recovery at 3 months (25.2% in citicoline, 20.2% in placebo, OR 1.33; 95% CI 1.10–1.62). The dose showing the largest difference with placebo was 2,000 mg, with 27.9% of patients achieving recovery. A randomised controlled study by Kuryata et al. [40] 2021 found that additional citicoline treatment could result in a decrease in the levels of glial fibrillary acidic protein (GFAP), neurofilament light subunit (NF-L) and myelin basic protein (MBP) compared to the control group. As such, citicoline could have a therapeutic effect on patients with AIS by protecting them from axonal injury, lowering levels of astrogliosis, decreasing demyelination, and protecting the function of microglia.
Calcium channel blockers: inhibition of cell death signalling
Increased calcium in cells may cause free radical generation through the peroxidation of membrane bound lipids and activation of proteases that lyse structural proteins and NO synthase. By inhibiting calcium entry, calcium channel blockers reduce free radical damage and may exhibit neuroprotective effects in animal models. However, human trials on calcium channel blockers in AIS have generally yielded disappointing results. A meta-analysis of 12 trials on nimodipine showed that overall, nimodipine had no neuroprotective effect on AIS, although it reduced mortality and delayed cerebral ischemia after subarachnoid hemorrhage (SAH) [77]. In a study of 1,064 AIS patients conducted by the American Nimodipine Study Group, there was no difference in mortality or neurological outcome found with placebo, 60 mg, 120 mg, and 240 mg of nimodipine for 21 days [41]. However, planned subgroup analysis showed a reduction in the frequency of worsening on day four as measured by the Toronto scale, compared with placebo and significantly better outcome scores with 120 mg nimodipine daily started within 18 h of stroke (P < 0.005) which requires further validation [41]. The SAVER-1 phase I clinical trial on 11 subjects showed that intraarterial verapamil administration in humans is safe and feasible, but its neuroprotective efficacy is not yet proven in humans [42]. Based on current evidence, calcium channel blockers do not appear to be neuroprotective in AIS, but studies on IV calcium channel blockers are lacking.
Modulation of the immune system and neuroinflammation
Activation of the immune system and migration of peripheral immune cells in the ischemia cascade may further increase free radical generation and neuronal damage. Several medications with immune modulatory effects have been investigated in AIS.
Natalizumab
Natalizumab is a monoclonal immunoglobulin G4 (IgG4) antibody that binds to integrin to inhibit immune cell migration, and the main application it is approved for is the treatment of relapsing-remitting multiple sclerosis [88]. In the multicentre ACTION II trial on AIS patients with NIHSS scores of 5–23, one dose of 300 mg or 600 mg IV natalizumab was given to patients [43]. However, results showed that the composite of excellent outcome (mRS ≤ 1, BI sore ≥ 95 at 90 days) was less likely with natalizumab than with placebo (OR 0.6, 95% CI 0.39–0.93), with no significant difference in mortality or adverse events [43].
MAPC
MAPC are stem cells derived from the bone marrow with immunomodulatory properties that are anti-inflammatory and has shown benefits in animal models of AIS [89]. In the phase II MASTERS RCT, 129 patients with moderately severe AIS (NIHSS 8–20) were randomised to IV MAPC or placebo between 12–48 h after symptom onset [44]. There were no differences in adverse events and global stroke recovery at 90 days, although post-hoc analysis showed efficacy in the earlier time window of 24 to 36 h. An analysis of the subgroup with stroke onset < 36 h showed the MAPC group had a higher chance of full or nearly full recovery at 1 year, and further trials evaluating the efficacy of MAPC in the earlier time window (< 36 h) are planned.
Minocycline
Minocycline is a tetracycline that inhibits microglial activation and causes decreased production of inflammatory cytokines including tumor necrosis factor-alpha (TNF-α) and interleukin-1 beta (IL-1β). In addition, minocycline decreases the production of inflammatory mediators like cyclooxygenase-2 (COX-2) and iNOS by inhibiting NF-κB activity. Furthermore, through decreasing T-cell proliferation, fewer inflammatory cytokines including TNF-α and interferon-γ are produced in animal studies [45]. A systematic review on minocycline reviewed 6 small, randomised trials in AIS patients, which have not demonstrated a clear neurological benefit but also were not sufficiently powered to draw definitive conclusions [45]. In another systematic review that included 7 RCTs on 426 patients, minocycline was associated with higher rates of 3-month functional independence (mRS-0-2) and BI in AIS patients [46]. The doses used ranged from 200 mg to 400 mg daily and were administered 6–24 h from the onset of symptoms. However, these trials were open-label, and larger double-blinded randomised trials are needed to confirm these results.
Imatinib
Imatinib is an anti-cancer drug that inhibits tyrosine kinase and specifically targets several proteins such as tyrosine kinases, Abelson (Abl)-related gene, c-Kit, platelet-derived growth factor receptor (PDGF-R), and breakpoint cluster region-Abl gene (BCR-ABL). It has been shown to have anti-inflammatory activity in reducing pro-inflammatory mediators such as TNF-α and IL-6. In a phase II RCT in 60 patients with AIS treated with thrombolysis, imatinib improved neurological outcomes by 0.6 NIHSS points per 100 mg of imatinib [47], and a larger RCT is ongoing to confirm these findings (NCT03639922).
Reduction of oxidative stress by antioxidants
NAC
According to Sabetghadam et al. [48] 2020, patients treated with NAC showed significantly lower mean NIHSS scores at day 90 compared to placebo. NAC treatment also reduced the blood levels of proinflammatory factors including IL-6, soluble intercellular cell adhesion molecule-1 (sICAM-1), NO, malondialdehyde (MDA), and neuron-specific enolase (NSE) and significantly increased serum levels of antioxidant biomarkers such as superoxide dismutase (SOD), glutathione peroxidase (GPx), and total thiol groups (TTG). Thus, NAC has good antioxidant and anti-inflammatory effects on patients after a stroke.
Co-ultramicronized PEA/Lut
Tissue injury and stress activate endogenous mechanisms to restore homeostatic balance and prevent further damage by upregulating the synthesis of lipid signalling molecules, including palmitoylethanolamide (PEA), which has positive neuroprotective effects and reduces inflammatory events due to ischemia reperfusion injury. In an observational study, 250 stroke patients undergoing neurorehabilitation were treated for 60 days with a pharmaceutical preparation of co-ultraPEALut (Glialia), a combination of PEA, and the antioxidant flavonoid luteolin [49]. Patients showed significant improvement in neurological status, impairment of cognitive abilities, the degree of spasticity, pain, and independence in daily living activities after 30 days of treatment compared to baseline. Further RCTs are needed to confirm the neuroprotective efficacy of co-ultraPEALut in AIS.
Butylphthalide
Butylphthalide activates the Keap1-Nrf2/ARE signalling pathway which is important in maintaining the redox equilibrium state in neurons. Butylphthalide can also potentially improve the cerebrovascular reserve of patients, and enhance the establishment of collateral compensatory vessels. A retrospective study by Zhang et al. [50] showed that butylphthalide injection for 2 weeks in AIS patients improved patients’ neurological function and activities of daily living. Compared to routine treatment, the treatment group had a superior overall response rate (ORR), showed a significantly higher BI score, lower NIHSS score, higher cerebrovascular vascular reserve function, and lower pulsation index (all P < 0.05). Additionally, in the observational group, the serum level of Keap 1 was remarkably higher (P < 0.05), while the serum levels of nicotinamide adenine dinucleotide phosphate [NAD(P)H] quinone dehydrogenase 1 (NQO1), Nrf2, and ARE were evidently compared to the control (P < 0.05). As no significant adverse reactions were observed, butylphthalide may be promising as an adjuvant therapy of AIS, and there is an RCT on the efficacy of butylphthalide in AIS which is ongoing [79].
HUK
HUK is a glycoprotein that treats stroke by positive regulation of the kallikrein-kinin, which through the kinin B2 receptor acts as an antioxidant and anti-inflammatory agent in protection against stroke [90]. RESK is a phase IV study which demonstrated that the single IV dose of HUK over 50 min every day for 14–21 continuous days had a favorable safety profile, and improvements in neurological and functional outcomes were observed in 1,202 AIS patients from baseline [51]. However, as this was not placebo-controlled, its efficacy compared to conventional treatment requires further study.
Melatonin
Melatonin reduces oxidative stress, neuroinflammation, and apoptosis induced by an ischemic stroke. A systematic review of 6 RCTs on melatonin showed it was generally safe, but no benefits were reported regarding the quality of life despite efficacy in animal models [52]. However, the doses used in animal studies were substantially higher than those administered in the human RCTs, and this may have contributed to the lack of efficacy seen.
Uric acid
Uric acid is an antioxidant in animal models, and its mechanism for neuroprotection in AIS is multifaceted [91]. In an animal study, the neuroprotective property of uric acid was associated with IL-6/signal transducers and activators of transcription 3 (STAT3) signaling pathway activation, attenuation of edematogenic vascular endothelial growth factor A (VEGF-A)/MMP-9 signaling, and modulation of oxidative stress, neuroinflammation, and apoptosis [92]. In an RCT involving 411 participants by Chamorro et al. [53], it was found that the addition of uric acid to thrombolytic therapy did not increase the proportion of patients who achieved excellent outcomes (mRS 0–1) after stroke compared with placebo.
Albumin
Albumin has antioxidant properties and modulates intracellular signalling of neuronal or glial cells to exert neuroprotective effects [93]. In the ALIAS RCTs I and II, treatment with 25% IV albumin did not show overall clinical benefit in AIS patients [54]. However, when albumin was initiated within 2 h, AIS patients with large cardioembolic stroke (NIHSS ≥ 15) showed a trend for benefit, suggesting that certain AIS patient subgroups most representative of preclinical settings may benefit.
Epigallocatechin gallate
Epigallocatechin gallate (EGCG) is a natural polyphenol that exerts antioxidant and anti-inflammatory effects. EGCG also induces the proliferation and differentiation of neural progenitor cells and promotes neurogenesis after AIS. In a study involving adult rats, after inducing middle cerebral artery occlusion injury, it was shown that EGCG exerted a neuroprotective effect by regulating caspase-3 and poly(adenosine diphosphate-ribose) polymerases (PARP) proteins during cerebral ischemia [94]. Another animal study confirmed that EGCG inhibited autophagy by modulating the protein kinase B (AKT)/5’adenosine monophosphate-activated protein kinase (AMPK)/mammalian target of rapamycin (mTOR) phosphorylation pathway in both in vivo and in vitro models of stroke [95].
Hypoglycemic agents: regulation of excitotoxicity
Hypoglycemic agents can have neuroprotective effects through the regulation of excitatory neurotransmitter glutamate and inhibitory neurotransmitter GABA, promoting glycogen synthesis, and inhibiting neuronal necrosis and apoptosis [96]. However, intensive glucose control post AIS was not beneficial in the SHINE trial, where 1,151 patients were randomised to receive continuous IV or subcutaneous insulin for up to 72 h within 12 h of AIS [55]. Treatment with intensive vs. standard glucose control did not result in a significant difference in functional outcome at 90 days but had an increased risk of severe hypoglycemic episodes [55].
Sulfonylureas
Glibenclamide is a sulfonylurea that inhibits the sulfonylurea receptor 1-transient receptor potential melastatin 4 (Sur1-Trpm4) channel and in some cases microglial Sur1-Kir6.2 channels. Several preclinical studies have found glibenclamide to be an effective treatment in rodent models of AIS, and retrospective cohort studies suggest that being on sulfonylureas and staying on it following brain ischemia may improve outcomes [56]. Prospective RCTs are needed to test the efficacy of glibenclamide in AIS. Glyburide is another anti-diabetic drug under the sulfonylurea class. The GAMES pilot study involving a small sample size of 10 administered a 3 mg dose of RP-1127 (Glyburide) to patients with severe stroke at high risk for swelling [57]. The GAMES-RP phase II RCT on 86 participants showed no significant difference in the primary efficacy outcome [58]. An exploratory analysis based on this clinical trial showed that IV glyburide had a significant effect on the BI (P = 0.03), and treated participants had lower plasma levels of MMP-9 (189 ng/mL vs. 376 ng/mL; P < 0.001) and decreased midline shift (4.7 mm vs. 9 mm; P < 0.001) and improved survival in participants ≤ 70 years of age with large hemispheric infarction.
Others
Statins
Statins are lipid lowering agents that upregulate endothelial NOS production, which increases cerebral blood flow, exerts anti-inflammatory effects, and may promote synaptogenesis and neurogenesis after AIS. According to the American Heart Association guidelines class IIa recommendations, among patients already taking statins at the time of AIS, a continuation of therapy during the acute period is reasonable [1]. However, the initiation of early statin therapy for its neuroprotective effect is still a topic of contention. In an RCT of 60 patients with cortical strokes, simvastatin within 3–12 h of symptom onset at a dose of 40 mg/day for one week followed by 20 mg/day until day 90 improved the neurological outcomes significantly by the third day, but there was a non-significant increase in mortality and a greater proportion of infections in the simvastatin group [59]. However, the ASSORT Trial involving 257 AIS and dyslipidemia patients did not show any superiority of early statin therapy within 24 h of admission compared with delayed statin therapy 7 days after admission on the degree of disability at 90 days after onset [60]. In this trial, possible reasons why no improvement in functional outcome was observed include the fact that patients included in the trial had relatively mild strokes with a median NIHSS of 3 and received less-intense statin regimens (atorvastatin 20 mg/day, pitavastatin 4 mg/day and rosuvastatin 5 mg/day). Future trials involving patients with more severe strokes and higher-intensity statins—atorvastatin 80 mg/day or rosuvastatin 20 mg/day or 40 mg/day can be considered. A meta-analysis of 22 observational studies on 17,554 patients showed that in-hospital statin use after IV thrombolysis was associated with a lower likelihood of symptomatic ICH, 3-month mortality, and 3-month favorable functional outcome (mRS score 0–1) but not pre-stroke statin use [61].
Cerebrolysin
Cerebrolysin is a mixture of low-molecular-weight peptides and amino acids derived from the porcine brain that has potential neuroprotective properties and neurotrophic activity. A meta-analysis of 6 RCTs showed that cerebrolysin had no significant effect on 90-day functional recovery on mRS, NIHSS, and BI scales [62]. Cerebrolysin probably has little or no beneficial effect on preventing all-cause death or reducing disability in AIS, and current evidence does not support its routine use.
Epo
Epo is a hormone that acts by binding to the Epo receptor (EpoR), and reduces BBB disruption after cerebral ischemia through reducing lipid peroxidation, downregulating the vascular endothelial growth factor receptor-2 (VEGFR-2) expression in the penumbra region, and decreasing MMP-2 and MMP-9 activity in ischemic microvessels, thus reducing apoptosis. Epo also regulates cell volume and neurogenesis in areas that suffered ischemic injury and reperfusion [97]. However, in the German multicentre Epo stroke RCT on 522 patients with middle cerebral artery stroke, IV human recombinant Epo showed no beneficial effects of Epo and increased overall death rate [67].
3K3A-activated protein C
3K3A-activated protein C is a blood protease with anticoagulant and cell-signalling properties which mechanism of action is by the activation of protease-activated receptors (PAR) 1 and 3 via non-canonical cleavage [98]. 3K3A-activated protein C has been shown to stimulate neuronal production by the human neural stem and progenitor cells in vitro [99]. The RHAPSODY trial was a phase II trial tested the use of 3K3A-activated protein C in combination with TPA or mechanical thrombectomy in AIS, which found toxicity in 7% but the possible reduction in hemorrhagic rate [70].
Other compounds that have been studied for neuroprotection include DDFPe, otaplimastat, dapsone, and theophylline. DDFPe emulsion was reported to be highly efficient at oxygen and carbon dioxide transportation in vivo. A phase Ib/II RCT involving 24 patients showed that IV DDFPe appears to be safe at all doses tested, and clinical improvements in NIHSS score and mRS were significant but compromised by a small sample size [68]. Otaplimastat inhibits MMP in animal stroke models through the upregulation of metalloproteinase-1. In the SAFE-TPA RCT, IV otaplimastat as an adjunct therapy in AIS patients receiving recombinant TPA (rTPA) was shown to be feasible and safe [69], and its functional efficacy needs to be investigated in large trials. Dapsone inhibits apoptosis, and a recent animal study suggests that dapsone represses proapoptotic proteins c-Jun N-terminal kinases (JNK), phosphatase and tensin homolog deleted on chromosome 10 (PTEN), calpain, and caspase-3 of cerebral ischemia and activates pro-survival protein brain-derived neurotrophic factor (BDNF) [100]. A pilot clinical trial conducted on 30 patients with AIS involving the middle cerebral artery territory showed that one dose of dapsone 200 mg compared to a placebo achieved improvement in NIHSS, BI, and mRS, with no adverse reactions reported. However, a larger trial is needed to study the treatment effects of dapsone [71].
Others-vasoactive substances
Some neuroprotective agents exert neuroprotective effects by improving cerebral blood flow to ischemic tissues. These include piracetam, theophylline, and GTN, as discussed below.
Piracetam
Piracetam is a nootropic reported to increase cerebral blood flow in both infarcted and penumbral tissues. Although there is limited evidence regarding its neuroprotective mechanism, it has been suggested that its ability to localize polar heads in the phospholipid bilayer restores membrane fluidity and facilitates membrane-bound cell functions such as ATP production, thus preventing neuronal cell death [101]. A systematic review in 2012 on piracetam that included 3 RCTs showed that there is some suggestion (but no statistically significant result) of an unfavorable effect of piracetam on early death, but this may have been caused by baseline differences in stroke severity in the trials [63]. There is no definite evidence of a beneficial or harmful effect on death in AIS, and the routine use of piracetam in the management of AIS is not recommended.
Theophylline
Theophylline, derived from methylxanthine, works via smooth muscle relaxation, bronchial dilation, and CNS stimulant activities [64]. In the phase II clinical study done by Modrau et al. [64] 2020, theophylline was used as an additional thrombolytic therapy. It showed improvement in terms of NIHSS. However, major clinical improvement defined as ≥ 50% improvement in NIHSS score from baseline to 24 h follow-up, and infarct growth was not significantly different between the intervention group and the control group.
GTN
NO donors, like GTN, have vasodilatory actions and may reduce elevated blood pressure in acute stroke. A patient-level meta-analysis of 5 RCTs on 4,197 patients with acute or subacute stroke found that GTN lowered the blood pressure by 7.4/3.3 mmHg (0.99/0.44 kPa) but did not alter 90-day outcomes [65]. In a subgroup of patients who received GTN within 6 h of stroke onset, mRS improved (OR 0.52, 95% CI 0.34–0.78) and mortality was reduced (OR 0.32, 95% CI 0.14–0.78) compared to placebo [65]. However, the MR ASAP phase III RCT which tested transdermal GTN within 3 h of stroke onset vs. standard care was prematurely terminated due to safety concerns in patients with intracerebral hemorrhage, and no difference in death within 90 days or serious adverse events were observed [66].
Future directions
Neuroprotection therapy administered as early as possible after stroke onset would allow the extension of survival time of the penumbra, minimizing the final infarct size and increasing the time window for reperfusion therapy [102]. However, no agents are currently approved by the US Food and Drug Administration for neuroprotection in AIS, due to the lack of evidence of efficacy in human trials. There are several challenges and limitations of clinical trials that need to be addressed for further progress to be made. One point of utmost importance is in ensuring an optimal time window for the administration of neuroprotective agents, due to the rapid progression of ischemic insult in the penumbra. Currently, many clinical trials administer neuroprotective agents after 6 h of stroke onset, which may be past the optimal time window for neuroprotection. Certain receptors also have a short time window for neuroprotective efficacy to be achieved. For instance, one reason NMDA antagonists have been unsuccessful in clinical trials is due to immediate toxicity instead of prolonged recovery [103]. The role of NMDAR in cerebral ischemia is complex, and although the sharp rise in glutamate levels immediately post ischemia is believed to be excitotoxic, neurotransmission through NMDAR in the longer term may be active pro-survival transcription factors, reduce apoptosis and enhance neuronal survival. The exact duration where the role of NMDARs alter is uncertain, but this may contribute to the failure to observe benefits with NMDARs in clinical trials [104]. Many neuroprotective agents target molecular mechanisms that occur soon after ischemia begins, despite data indicating delayed patient presentation following AIS, and as such many pharmaceutical agents have failed [105]. Some neuroprotective agents like magnesium also require time to cross the BBB and hence may not achieve optimal neuroprotective concentration in the CSF in the effective time window. In the clinical trial setting, it may be challenging to ensure the timely administration of the test agent due to delays with recruitment. These delays resulted in an extension of the inclusion criteria to stroke onset of < 48 h compared to the original < 36 h in the MASTERS trial on MAPC to increase enrolment, which may have impacted the trial results [44]. Therefore, in the setting of AIS where timing is critical, the protocol of any RCT must align with the reperfusion pathways present at the clinical center in order to facilitate trial enrolment without affecting other time-based stroke therapies.
In addition to the administration of neuroprotective agents in the acute setting, longer courses of therapy post-AIS may also be effective. For instance, butylphthalide injection given for 2 weeks and memantine given for three months have shown positive effects in achieving improvement in neurological outcomes in RCTs [11, 79]. Repeat dosing may be explored for other agents and the measure of stroke outcome in future clinical trials can include the degree of neurological deficit measured over longer time periods, in addition to measurement of infarct volume or measurement of neurological outcomes in the short term, to increase the chances of detecting the efficacy of neuroprotective agents.
Many neuroprotective agents seem promising at the preclinical stage in animal trials but fail in clinical trials. This could be due to poor design of experimental models, for instance experimenting in young animals unable to mimic the presence of comorbidities in AIS in older humans. Hence, higher quality of preclinical models, such as through the inclusion of animal models with comorbidities and older age can better represent AIS in humans [7]. The translation of animal studies to human studies is also challenging, as the effective doses may differ. For example, although melatonin reduced infarct size, reduced edema, inflammatory, and apoptotic response in animal studies, RCTs in humans were largely negative. A dose equivalent calculation found that the doses used in animals were 5–15 fold higher than that of human RCTs [52]. Therefore, it is important to consider the appropriate dose as well as the timing of neuroprotective therapy when designing future RCTs, based on preclinical data. Improving the available animal models such as using aged models to account for the effects of aging, using models with co-morbidities such as diabetes, hypertension, or hyperlipidemia, and studying more clinically relevant outcomes such as functional outcomes rather than infarct volume alone may improve translation of findings from preclinical to clinical studies [105].
Furthermore, a single neuroprotective agent may not act on enough levels in the ischemic injury molecular cascade, which limits its efficacy in improving neurological outcomes. In animal models, atorvastatin with anti-inflammatory properties combined with probucol with antioxidant properties reduced brain water content and infarct size [106]. Currently, ongoing clinical trials on neuroprotective agents include pleiotropic agents that target multiple aspects of the neurodegenerative cascade and may have synergistic neuroprotective effects [79]. Studies reporting the efficacy of neuroprotective agents combined with reperfusion therapy such as IVT and EVT are currently scarce [107]. Animal studies reported promising findings, for example in rats recombinant TPA combined with optimized human apyrase may reduce hemorrhagic transformation after AIS [108]. Future RCTs should focus on this setting with the increasing clinical availability of reperfusion therapy. Other combination therapies can also be considered in certain situations. For instance, amyloid-beta (Aβ) plaque accumulation and amyloid oligomer mediated pathology is implicated in both stroke and Alzheimer’s disease, therefore both Aβ toxicity and neuroinflammation may be potential targets in both diseases [109]. Combination with non-pharmacological neuroprotective methods may also be beneficial. A systemic review of 15 studies reported that improved patient outcomes were present for combination treatments including uric acid with EVT and IVT, NA-1 and EVT, imatinib and IVT, remote ischemic preconditioning and IVT, and high-flow normobaric oxygen treatment after EVT [107]. In addition, therapeutic hypothermia in combination with other neuroprotectants also provided greater success and better patient outcomes compared to neuroprotective agent administration alone [110], and future studies can consider this combination of treatments.
Conclusions
In this paper, the molecular mechanisms of different neuroprotective agents that have been used in human trials were discussed. Although most neuroprotective agents investigated thus far do not have well established efficacy in improving neurological outcomes in AIS patients, some agents appear promising, having shown positive outcomes in preliminary human trials. These drugs require further research to establish their efficacy in AIS patients. These include NMDA antagonist memantine, free radical scavenger edaravone, nerenitide NA-1, citicoline, statins, immune system modulators including dapsone and minocycline, and antioxidants like NAC, butylphthalide, and melatonin. Neuroprotection is important in improving AIS outcomes, and hence proper study design for translation into clinical trials is crucial. Currently, pre-clinical and clinical trial designs improved in administering treatment under optimal conditions to increase the chances of detecting the neuroprotective efficacy of these agents. In light of this, research on neuroprotective strategies holds much promise. With time, we can look forward to the development of neuroprotective agents that may ultimately prove to be effective in AIS.
Abbreviations
AIS: | acute ischemic stroke |
AMPA: | α-amino-3-hydroxy-5-methyl-4-isoxazolepropionic acid |
ARE: | antioxidant response element |
ASSORT: | administration of statin on acute ischemic stroke patient |
BBB: | blood-brain barrier |
BI: | Barthel Index |
CI: | confidence interval |
CNS: | central nervous system |
co-ultraPEALut: | co-ultramicronized palmitoylethanolamide/Luteolin |
DDFPe: | dodecafluoropentane |
EGCG: | epigallocatechin gallate |
Epo: | erythropoietin |
EVT: | endovascular therapy |
FAST-MAG: | field administration of stroke therapy-magnesium |
GABA: | gamma-aminobutyric acid |
GAMES: | glyburide advantage in malignant edema and stroke |
GAMES-RP: | glyburide advantage in malignant edema and stroke-remedy pharmaceuticals |
GTN: | glyceryl trinitrate |
HUK: | human urinary kallidinogenase |
IL-1β: | interleukin-1 beta |
IMAGES: | intravenous Magnesium efficacy in stroke |
IV: | intravenous |
IVT: | intravenous thrombolysis |
MAPC: | multipotent adult progenitor cells |
MASTERS: | multipotent adult progenitor cells in acute ischemic stroke |
MMP: | matrix metalloproteinase |
mRS: | modified Rankin score |
NAC: | N-acetylcysteine |
NIHSS: | National Institutes of Health Stroke Scale |
NMDA: | N-methyl-D-aspartate |
NMDAR: | N-methyl-D-aspartate receptor |
NO: | nitric oxide |
Nrf2: | nuclear factor erythroid 2-related factor 2 |
NXY: | disodium 2,4-sulphophenyl-N-tert-butylnitrone |
OR: | odds ratio |
RCT: | randomized controlled trial |
RESK: | re-evaluate the efficacy and safety of human urinary kallidinogenase |
SAFE-TPA: | safety and efficacy of otaplimastat in patients with acute ischemic stroke requiring tissue plasminogen activator |
SAINT: | stroke-acute ischemic disodium 2,4-sulphophenyl-N-tert-butylnitrone treatment trial |
SAVER: | surgical anterior ventricular restoration |
SHINE: | the stroke hyperglycemia insulin network effort |
TNF-α: | tumor necrosis factor-alpha |
TPA: | tissue plasminogen activator |
Declarations
Author contributions
GYYC: Conceptualization, Investigation, Data curation, Writing—original draft. JSYH: Conceptualization, Investigation, Validation, Writing—review & editing, Supervision. BYQT: Conceptualization, Investigation, Validation, Writing—review & editing, Supervision. SY: Data curation, Writing—original draft. EJ: Validation, Writing—review & editing, Supervision. LLLY: Validation, Writing—review & editing, Supervision.
Conflicts of interest
The authors declare that they have no conflicts of interest.
Ethical approval
Not applicable.
Consent to participate
Not applicable.
Consent to publication
Not applicable.
Availability of data and materials
Not applicable.
Funding
Not applicable.
Copyright
© The Author(s) 2023.