Abstract
Recent investigations have shed light on the potential of seaweed, an abundant source of bioactive compounds, to mitigate and combat neurodegenerative diseases. In this comprehensive review, the accumulating evidence supporting the neuroprotective properties of seaweed-derived compounds is evaluated and their putative mechanisms of action are elucidated. The background of this review encompasses the general understanding of neurodegenerative diseases as debilitating conditions characterized by the progressive loss of nerve cell function and viability in the central nervous system. Furthermore, the global prevalence of these diseases, encompassing Alzheimer’s disease, Parkinson’s disease, and Huntington’s disease, and the persistent absence of effective treatments are emphasized. To address this critical issue, an innovative avenue of research is explored by investigating the potential of seaweed and its diverse array of bioactive compounds. By examining the available literature, the evidence supporting the neuroprotective effects of seaweed-derived compounds is consolidated. These bioactive constituents exhibit promising properties in preventing and mitigating neurodegeneration. Mechanistically, their actions involve intricate pathways that contribute to neuronal survival, reduction of oxidative stress, inhibition of neuroinflammation, and modulation of protein aggregation processes. This review provides a comprehensive analysis of the mechanisms underlying the neuroprotective effects of seaweed compounds. In conclusion, this review highlights the potential of seaweed as a valuable source of neuroprotective compounds and underscores the advancements made in this burgeoning field. The identification and elucidation of the mechanisms through which seaweed compounds exert their neuroprotective effects hold promise for the development of novel therapeutic interventions. These findings transcend disciplinary boundaries, offering insight into the potential application of seaweed-derived compounds as a valuable resource for combating neurodegenerative diseases across scientific domains.
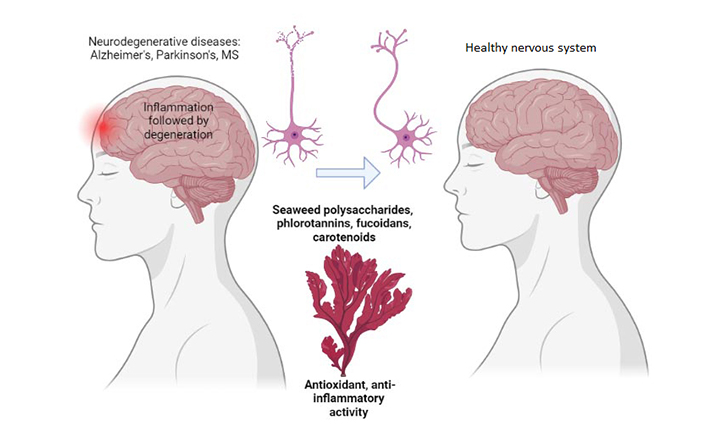
The power of marine algae for neuroprotection. MS: multiple sclerosis. Created with BioRender.com
Keywords
Neuroprotectant, neurodegenerative diseases, plant-derived compounds, polysaccharides, carotenoids, antioxidants, anti-inflammatory agentsIntroduction
Seaweed, commonly referred to as marine macroalgae, comprises a diverse group of multicellular plants thriving in marine environments. Throughout centuries, it has been a dietary staple in numerous Asian cultures, acclaimed for its manifold health benefits, including its potential neuroprotective effects [1, 2]. The investigation of seaweed and its bioactive compounds on brain health has yielded promising findings, prompting this manuscript to offer a comprehensive overview of the current understanding surrounding the potential advantages of seaweed compounds in the prevention and combat of neurodegenerative diseases (NDs) [3, 4].
Multiple studies have explored the impact of seaweed and its bioactive compounds on brain function, unveiling encouraging outcomes. Notably, a study conducted in Japan discovered that elderly individuals who regularly consumed seaweed exhibited a diminished risk of developing dementia compared to non-consumers [5]. Similarly, a study conducted in Korea demonstrated that a diet abundant in seaweed correlated with enhanced cognitive function among older adults [6].
The potential neuroprotective effects of seaweed compounds are often attributed to their antioxidative, anti-inflammatory, and anti-apoptotic properties [7]. These compounds have demonstrated the ability to scavenge reactive oxygen species (ROS), mitigate inflammation, and impede programmed cell death—factors crucial in the development and progression of NDs [8]. Moreover, apart from their direct influence on the brain, seaweed compounds may indirectly exert their neuroprotective effects by modulating the gut microbiota. Recent studies have implicated the gut microbiota in the pathogenesis of NDs, suggesting that interventions targeting this microbial ecosystem hold therapeutic potential. Seaweed compounds have exhibited the capacity to modulate the gut microbiota, implying additional avenues for their neuroprotective effects [9].
Although further research is imperative to comprehensively elucidate the mechanisms of action and clinical efficacy of seaweed compounds in preventing and treating NDs, the existing evidence is indeed promising. Seaweed represents a natural and sustainable reservoir of bioactive compounds, thus integrating it into the diet may present a safe and effective strategy for reducing the risk of NDs [3, 10].
In summary, the introduction has established the overall context by introducing seaweed as a diverse group of marine macroalgae with a long history of being a dietary staple in Asian cultures. The specific context is presented by highlighting the potential neuroprotective effects of seaweed compounds, supported by studies indicating the lower risk of dementia and better cognitive function associated with seaweed consumption. The current problem addressed in this review is the need for a comprehensive understanding of the potential benefits of seaweed compounds in preventing and combating NDs. By exploring the antioxidative, anti-inflammatory, and anti-apoptotic properties of seaweed compounds, as well as their potential impact on the gut microbiota, this review aims to shed light on their mechanisms of action and therapeutic potential.
Seaweed compounds and their potential neuroprotective effects
Seaweed compounds have received increasing attention for their potential neuroprotective effects against NDs. Several studies have demonstrated the ability of seaweed compounds to protect brain cells from damage and degeneration, thus potentially slowing or preventing the progression of these diseases [11].
One of the main groups of seaweed compounds that have been studied for their neuroprotective effects are the polysaccharides. These complex carbohydrates have been shown to have antioxidant and anti-inflammatory properties, which can help to reduce oxidative stress and inflammation in the brain, two key contributors to NDs such as Alzheimer’s disease (AD) and Parkinson’s disease (PD) [12, 13].
AD has been linked to a deficiency in the neurotransmitter acetylcholine (ACh), as demonstrated by multiple neuropathological studies [14]. One of the most promising methods of treating the symptoms of AD is inhibiting the acetylcholinesterase enzyme (AChE), which breaks down ACh [15]. This pathology is associated with the enzymatic reaction of β-secretase (BACE1) on the amyloid precursor protein (APP) for the generation of neurotoxic amyloid β (Aβ) [16]. Several studies have reported AChE inhibitory activity in various species of marine algae.
Another group of seaweed compounds with potential neuroprotective effects is the phlorotannins. These polyphenolic compounds have been shown to have antioxidant and anti-inflammatory properties, as well as the ability to inhibit the formation of β-amyloid plaques, which are a hallmark of AD [17, 18].
Fucoidan is another bioactive compound found in seaweed that has been studied for its potential neuroprotective effects. Fucoidan has been shown to have anti-inflammatory and antioxidant properties, and it can also inhibit the formation of tau protein, which is another hallmark of AD [3, 11, 19].
Carotenoids, such as fucoxanthin, are also present in seaweed and have been shown to have neuroprotective effects. Fucoxanthin has been shown to have antioxidant properties and can reduce oxidative stress in the brain, which can help to protect against NDs, by both subsiding pro-inflammatory mediators and enhancing brain-derived neurotrophic factor (BDNF) [20, 21].
Furthermore, seaweed compounds may exert their neuroprotective effects by modulating the gut microbiota. The gut-brain axis is a bidirectional communication pathway between the gut and the brain, and recent studies have shown that modulating the gut microbiota can have positive effects on brain function and NDs. Seaweed compounds have been shown to modulate the gut microbiota, thus potentially exerting their neuroprotective effects through this mechanism [22, 23].
Thus, seaweed compounds have shown great potential for their neuroprotective effects against NDs. Their antioxidant, anti-inflammatory, and anti-amyloid properties, as well as their ability to modulate the gut microbiota, make them a promising natural compound for the prevention and treatment of NDs [24]. However, further studies are needed to fully elucidate the mechanisms of action and the clinical efficacy of these compounds (Table 1) [25, 26].
Neuroprotective effects of some compounds extracted from seaweeds
Algae | Extracts or compounds | Activity | References |
---|---|---|---|
Agarum clathratum subsp. yakishiriense (P) | Ethyl acetate, n-butanol extracts, and crude extract | Neuronal protection from ischemic injury | [27] |
Alaria esculenta (P) (Figure 1a) | Methanol and water extract | The formation of amyloid fibrils by α-synuclein is inhibited by the extract fractions | [28] |
Amphiroa beauvoisii (R) (Figure 1b) | Aqueous and methanol extracts | Inhibiting AChE IC50 = 0.12 mg/mL | [29] |
Amphiroa bowerbankii (R) | Methanol extracts | AChE inhibition | [30] |
Amphiroa ephedraea (R) | Methanol extracts | AChE inhibition | [30] |
Asparagopsis armata (R) (Figure 1c) | Methanol extracts | AChE and BuChE inhibition | [31] |
Bifurcaria bifurcata (P) (Figure 1d) | Eleganolone, eleganonal (diterpenes) | Antioxidant and neuroprotective potential in PD | [7] |
Capsosiphon fulvescens (C) | Glycoproteins | Reduces aging-induced cognitive dysfunction | [32, 33] |
Caulerpa racemosa (C) (Figure 1e) | Methanolic extract | AChE inhibition | [30] |
C. racemosa (C) | Racemosins A and B | Neuro-protective activity | [34] |
Chondracanthus acicularis (R) (Figure 1f) | Carrageenan λ | Antioxidant activity | [35] |
Chondrus crispus (R) (Figure 1g) | Methanol extracts | Extract-mediated protection against PD | [36] |
Cladophora vagabunda (formerly Cladophora fascicularis) (C) | Methanol extracts | AChE inhibition | [3] |
Codium capitatum (C) | Methanol extracts | AChE inhibition | [30] |
C. capitatum (C) | Aqueous and methanolic extracts | AChE inhibition | [29] |
Codium duthieae (C) | Aqueous and methanolic extracts | AChE inhibition | [29] |
Codium tomentosum (C) (Figure 1h) | Dichloromethane extract | Antioxidant activity | [37] |
Cystoseira humilis (P) (Figure 1i) | Methanolic extract | AChE inhibition | [31] |
Dictyopteris undulata (P) | Sesquiterpene: zonarol | Antioxidant activity | [38] |
Ecklonia bicyclis (P) | Phlorotannins | Suppression of BACE1 activity | [39] |
Ecklonia cava subsp. stolonifera (formerly E. stolonifera) (P) | Fucosterol | Prevents cognitive dysfunction induced by soluble Aβ | [40] |
Ecklonia maxima (P) (Figure 1j) | Phlorotannin: eckmaxol | Anti-Aβ oligomer neuroprotective effect | [41, 42] |
Ecklonia radiata (P) | Fucofuroeckol-type phlorotannins | Exhibits a wider range of neuroprotective activity against both oxidative stress and Aβ exposure | [18] |
Eucheuma denticulatum (R) (Figure 1k) | Iota-carrageenan | Antioxidant activity | [43] |
Ericaria selaginoides (formerly Cystoseira tamariscifolia) (P) (Figure 1l) | Methanolic extract | AChE and BuChE inhibition | [31] |
Fucus vesiculosus (P) (Figure 2a) | Fucoidan | Prevents the loss of dopaminergic neurons | [44] |
F. vesiculosus (P) | Fucoidan | Antioxidant activity | [35] |
F. vesiculosus (P) | Fucoidan | Protective effect | [45] |
F. vesiculosus (P) | Fucoidan | At a concentration of 10 µmol/L, fucoidan inhibits the clustering of microglial cells induced by Aβ | [46] |
F. vesiculosus (P) | Phlorotannins | Suppressing the overproduction of intracellular ROS induced by hydrogen peroxide IC50 = 0.068 mg/mL | [47] |
F. vesiculosus (P) | Fucoidan | Neuroprotection against transient global cerebral ischemic injury | [48] |
Gelidiella acerosa (R) | Extracts obtained include petroleum ether, hexane, benzene, dichloromethane, chloroform, ethyl acetate, acetone, methanol, and water | AChE and BuChE inhibition | [49] |
G. acerosa (R) | Phytol | AChE and BuChE inhibition | [50] |
Gelidium amansii (R) | Ethanol extract | Neurogenesis (synaptogenesis promotion) | [51, 52] |
Gloiopeltis foliaceum (R) | Aqueous and methanolic extracts | AChE inhibition | [29] |
Gloiopeltis furcata (R) | The compounds obtained consist of 2-(3-hydroxy-5-oxotetrahydrofuran-3-yl) acetic acid, glutaric acid, succinic acid, nicotinic acid, (E)-4-hydroxyhex-2-enoic acid, cholesterol, 7-hydroxycholesterol, uridine, glycerol, phlorotannin, and fatty acids | AChE and BuChE inhibition | [53] |
Gongolaria nodicaulis (formerly Cystoseira nodicaulis) (P) (Figure 2b) | Methanolic extract | AChE and BuChE inhibition | [31] |
Gongolaria usneoides (formerly Cystoseira usneoides) (P) (Figure 2c) | Methanolic extract | AChE and BuChE inhibition | [31] |
Gracilaria cornea (R) | Sulphated agaran | Neuroprotective effects in rat model PD | [54] |
Gracilaria edulis (R) | Methanol extracts | AChE inhibition | [55] |
Gracilaria gracilis (R) (Figure 2d) | Methanol extracts | AChE inhibition | [55] |
Gracilariopsis chorda (R) | Ethanol extracts | Ethanol extract exhibited the highest neuroprotective effects at a concentration of 15 µmol/L. At this concentration, the G. chorda extract significantly enhanced cell viability to 119.0% ± 3.2% and reduced cell death to 80.5% ± 10.3% | [56] |
G. chorda (R) | Ethanolic extract | Extract concentration-dependently increased neurite outgrowth | [57] |
Halimeda incrassata (C) | Water extracts | Neuroprotective and antioxidant properties | [58] |
Halimeda cuneata (C) | Methanol extracts | AChE inhibition | [30] |
H. cuneata (C) | Aqueous and methanol extracts | AChE inhibition | [29] |
Hypnea valentine (R) | Methanol extracts | AChE inhibition | [59] |
H. valentiae (R) | Methanol extracts | AChE inhibition | [59] |
Ishige okamurae (P) | Phlorotannin (6,6’-bieckol) | AChE inhibition | [60] |
I. okamurae (P) | Phlorotannin (DPHC) | The neuroprotective effect against hydrogen peroxide (H2O2)-induced oxidative stress in murine hippocampal neuronal cells was observed with an IC50 value of 50 µmol/L | [61] |
Kappaphycus alvarezii (R) (Figure 2e) | Ethanol extracts | Stimulates the extension of neurites in hippocampal neurons | [62] |
Marginariella boryana (P) | Sulfated fucans | Prevents the accumulation of Aβ | [63] |
Ochtodes secundiramea (R) | Dichloromethane and methanol extracts: Halogenated monoterpenes | AChE inhibition | [64] |
Padina australis (P) | Dichloromethane extract | AChE inhibition | [65] |
Padina gymnospora (P) (Figure 2f) | Methanol extracts | AChE inhibition | [55] |
P. gymnospora (P) | Acetone extracts | AChE and BuChE inhibition | [66] |
Padina pavonica (P) (Figure 2g) | Methanol extracts | Antioxidant activity on 6-OHDA-induced neurotoxicity in the human neuroblastoma cell line SH-SY5Y | [37] |
Padina tetrastromatica (P) | Fucoxanthin | Demonstrates antioxidant activity by effectively decreasing lipid peroxidation in rats, with an IC50 value of 0.83 μmol/L | [67] |
P. tetrastromatica (P) | Chloroform and ethanol extracts | The chloroform extract exhibited notable anticonvulsant activity at a dose of 600 mg/kg | [68] |
Papenfussiella lutea (P) | Sesquiterpenes | AChE inhibition | [69] |
Porphyra capensis (R) | Porphyran | Prevents loss of dopaminergic neurons | [70] |
Porphyra and Pyropia sp. (R) | Phycoerythrobilins | Antioxidant activity | [71] |
Pyropia haitanensis (R) | Porphyran | An agent that combats neurotoxicity induced by Aβ peptide in AD | [72] |
Pyropia yezoensis (formerly Porphyra yezoensis) (R) | Ethanol extracts | Increased neurite outgrowth at an optimal concentration of 15 µg/mL | [73] |
P. yezoensis (as Porphyra yezoensis) (R) | Oligo-porphyran | Agent with anti-neurotoxic properties suitable for preventing and treating a range of neurological disorders | [74] |
Rhodomela confervoides (R) | Bromophenol | Antioxidant action | [75] |
Rhodomelopsis africana (R) | Aqueous and methanol extracts | AChE inhibition | [29] |
Saccharina japonica (P) | Fucoidan | Demonstrates a protective effect against neurotoxicity induced by MPTP. Moreover, it diminishes behavioral deficits and cell death while enhancing dopamine levels IC50 = 25 mg/kg, once per day in mice | [76] |
S. japonica (P) | Fucoidan | Inhibitory effect of fucoidan on nitric oxide production in lipopolysaccharide-activated primary microglia. The IC50 value for this inhibition is 125 μg/mL | [77] |
S. japonica (P) | Fucoidan | Antioxidative activity | [78] |
S. japonica (P) | Ethanolic extract | Promoted neurite outgrowth in a dose-dependent manner with optimal concentrations of 15 μg/mL | [52, 79] |
S. japonica (P) | Fucoidan | Reduced 6-OHDA and reduced the loss of dopaminergic in neurons IC50 = 20 mg/kg in rats | [80] |
Saccorhiza polyschides (P) (Figure 3a) | Methanol extracts | Displays antioxidant activity against 6-OHDA-induced neurotoxicity in the SH-SY5Y human neuroblastoma cell line | [37] |
Sargassum aquifolium (formerly Sargassum crassifolium) (P) | Crude extracts of fucoidan | Antioxidant and neuroprotective properties | [81] |
Sargassum fulvellum (P) | Pheophytin A | Stimulates neurite outgrowth, increasing it from 20% to 100% in the presence of 10 ng/mL of NGF. Additionally, it exhibits an activating effect with an IC50 value of 3.9 μg/mL in PC12 cells | [82] |
S. fulvellum (P) | Ethanol extracts | Induced dose-dependent promotion of neurite outgrowth, with optimal concentrations observed at 5 μg/mL | [83] |
Sargassum fusiforme (formerly Hijikia fusiformis) (P) | Fucoxanthins | Exhibits antioxidative activity by effectively scavenging DPPH radicals | [84] |
S. fusiforme (P) | Fucoidan | Shows potential in ameliorating learning and memory deficiencies and serves as a potential ingredient for the treatment of AD | [85] |
Sargassum horneri (P) | Total sterols and β-sitosterol | Antidepressant effect | [86] |
S. horneri (P) | Fucoxanthins | Attenuates Aβ oligomer-induced neuronal apoptosis in SH-SY5Y cells | [87] |
S. horneri (P) | Fucoxanthins | Fucoxanthin reduces H2O2-induced neuronal apoptosis in SH-SY5Y cells | [88] |
Sargassum macrocarpum (P) | Carotenoids | Enhance PC12 cell neurite outgrowth activity to 0.4 with an IC50 of 6.25 μg/mL | [89] |
S. macrocarpum (P) | Sargaquinoic acid | TrkA-MAPK pathway mediates the signaling process with an IC50 of 3 μg/mL | [90] |
S. macrocarpum (P) | Sargachromenol | Activate cAMP and MAPK pathways to enhance the survival of PC12 cells and promote neurite outgrowth, with an IC50 of 9 μmol/L | [91] |
Sargassum micracanthum (P) | Plastoquinones | Exhibit anti-oxidative activity by inhibiting lipid peroxidation, with an IC50 range of 0.95–44.3 μg/mL | [92] |
Sargassum muticum (P) (Figure 3b) | Methanolic extract | Demonstrate antioxidant activity against 6-OHDA-induced neurotoxicity in the human neuroblastoma cell line SH-SY5Y | [93] |
Sargassum polycystum (P) | Hexane, dichloromethane, and methanol extract | AChE inhibition | [65] |
Sargassum sagamianum (P) | Sesquiterpenes | AChE inhibition | [69] |
S. sagamianum (P) | Sargaquinoic acid and sargachromenol | AChE and BuChE inhibition | [94] |
Sargassum siliquastrum (P) | Fucoxanthin | Exhibit anti-oxidative activity by inhibiting hydrogen peroxide in vero cells, with an IC50 of 100 μmol/L | [95] |
S. siliquastrum (P) | Meroditerpenoids | These compounds demonstrated moderate to significant radical-scavenging activity while also displaying weak inhibitory effects on sortase A and isocitrate lyase | [96] |
Sargassum sp. (P) | Methanol extracts | AChE inhibition | [55] |
Sargassum swartzii (formerly Sargassum wightii) (P) | Alginic acid | The polysaccharides exhibited inhibitory activities against COX-2, 5-LOX, XO, and MPO in type II collagen-induced arthritic rats, with an IC50 of 100 mg/kg | [97] |
S. swartzii (formerly S. wightii) (P) | Petroleum ether, hexane, benzene, and dichloromethane extracts | AChE and BuChE inhibition | [98] |
Sargassum vulgare (P) | Methanolic extract | AChE inhibition | [31] |
Scytothamnus australis (P) | Sulfated fucans | Prevents the accumulation of Aβ | [63] |
Splachnidium rugosum (P) | Sulfated fucans | Inhibits the Aβ accumulation | [63] |
Turbinaria decurrens (P) | Fucoidan | Shows potential for a neuroprotective effect in PD | [99] |
Ulva australis (formerly Ulva pertusa) (C) | Sulfated polysaccharide (ulvan) | Scavenging activity for superoxide radicals | [100, 101] |
Ulva compressa (C) | Dichloromethane extract | Exhibits antioxidant activity against neurotoxicity induced by 6-OHDA in the human neuroblastoma cell line SH-SY5Y | [93] |
Ulva fasciata (C) | Methanolic extract | AChE inhibition | [30] |
U. fasciata (C) | 50% aqueous methanol extract | AChE inhibition | [29] |
Ulva prolifera (formerly Enteromorpha prolifera) (C) | Pheophorbide A | Displays antioxidant activity with an IC50 of 71.9 µmol/L | [102] |
Ulva reticulata (C) | Methanol extracts | AChE inhibition | [59] |
Undaria pinnatifida (P) | Ethanol extracts | Neurite outgrowth was enhanced in a manner that correlated with the dosage, reaching optimal levels at concentrations of 5 μg/mL | [79, 103] |
U. pinnatifida (P) | Ethanol extracts | The activities displayed encompass neurogenesis, neuroprotection, anti-inflammatory effects, and anti-Alzheimer’s properties | [104] |
U. pinnatifida (P) | Glycoprotein | The observed effects included neurogenesis, neuroprotection, anti-inflammatory properties, and anti-Alzheimer’s potential. Notably, significant inhibitory activities against AChE, BChE, and BACE1 were demonstrated, with IC50 values of 63.56 μg/mL, 99.03 μg/mL, and 73.35 μg/mL, respectively | [105] |
U. pinnatifida (P) | Sulfated fucans | It inhibits the buildup of Aβ | [63] |
Zonaria spiralis (P) | Spiralisone A and chromone 6 | It displayed inhibitory effects on CDK5/p25, CK1δ, and GSK3β kinases, with IC50 values of 10.0 μmol/L, < 10 μmol/L, and < 10 μmol/L, respectively | [106] |
C: Chlorophyta (green macroalgae); P: Phaeophyceae (brown macroalgae); R: Rhodophyta (red macroalgae); IC50: half maximal inhibitory concentration; BuChE: butyrylcholinesterase; DPHC: diphlorethohydroxycarmalol; 6-OHDA: 6-hydroxydopamine; MPTP: 1-methyl-4-phenyl-1,2,3,6-tetrahydropyridine; MAPK: mitogen-activated protein kinase; NGF: nerve growth factor; DPPH: 2,2-diphenyl-1-picrylhydrazyl; TrkA: tropomyosin receptor kinase A; cAMP: cyclic adenosine monophosphate; COX-2: cyclooxygenase-2; 5-LOX: 5-lipoxygenase; XO: xanthine oxidase; MPO: myeloperoxidase; CDK5: cyclin-dependent kinase 5; CK1δ: casein kinase 1; GSK3β: glycogen synthase kinase 3β
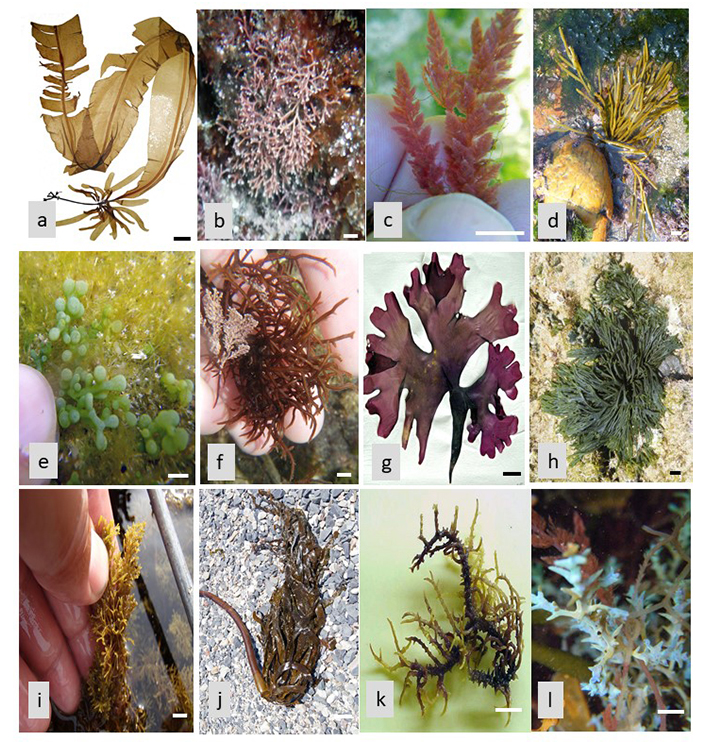
(a) Alaria esculenta; (b) Amphiroa beauvoisii; (c) Asparagopsis armata; (d) Bifurcaria bifurcata; (e) Caulerpa racemose; (f) Chondracanthus acicularis; (g) Chondrus crispus; (h) Codium tomentosum; (i) Cystoseira humilis; (j) Ecklonia maxima; (k) Eucheuma denticulatum; (l) Ericaria selaginoides. Scale bar = 1 cm
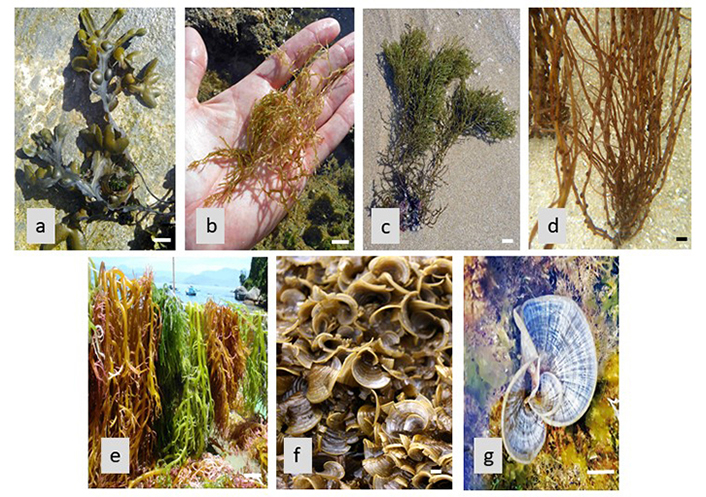
(a) Fucus vesiculosus; (b) Gongolaria nodicaulis; (c) Gongolaria usneoides; (d) Gracilaria gracilis; (e) Kappaphycus alvarezii; (f) Padina gymnospora; (g) Padina pavonica. Scale bar = 1 cm
Note. Figure 2b and 2c adapted from “Pioneering role of marine macroalgae in cosmeceuticals,” by Kalasariya HS, Pereira L, Patel NB. Phycology. 2022;2:172–203 (https://www.mdpi.com/2673-9410/2/1/10). CC BY. Figure 2d and 2e adapted from “The seaweed diet in prevention and treatment of the neurodegenerative diseases,” by Pereira L, Valado A. Mar Drugs. 2021;19:128 (https://www.mdpi.com/1660-3397/19/3/128/html). CC BY.
Antioxidant effects
Neurodegenerative disorders, including AD, PD, and Huntington’s disease, involve the gradual degeneration of nerve cells in the brain and nervous system. Oxidative stress, marked by an imbalance of ROS and inadequate detoxification mechanisms, is recognized as a significant contributor to this neuronal loss [107]. ROS are unstable molecules that can damage cells by reacting with lipids, proteins, and DNA. They are produced as a byproduct of normal cellular metabolism and can also be generated in response to environmental toxins and other stressors [108]. When the levels of ROS become too high, they can lead to oxidative stress, which can cause inflammation and damage to nerve cells, ultimately leading to their death [109].
Neurodegenerations are often associated with oxidative stress, a condition in which there is an imbalance between the production of ROS and the body’s ability to detoxify them [110]. Seaweed compounds have been shown to scavenge ROS, reducing oxidative stress and preventing damage to nerve cells [111]. For example, phlorotannins, a type of polyphenol found in brown seaweed, have been shown to have potent antioxidant effects and protect against oxidative stress-induced neurotoxicity [112].
Seaweed compounds, particularly phlorotannins, have been found to have potent antioxidant effects and can scavenge ROS, reducing oxidative stress and preventing damage to nerve cells [113]. Phlorotannins are a type of polyphenol found in brown seaweed, and they are known for their strong antioxidant properties [114]. Studies have shown that phlorotannins can protect against oxidative stress-induced neurotoxicity in cell culture and animal models. For example, one study found that treatment with phlorotannins from brown seaweed protected rat brain cells from oxidative stress-induced cell death [115]. Another study found that phlorotannins from brown seaweed improved cognitive function and reduced oxidative stress in mice with AD [116].
Overall, these findings suggest that seaweed compounds, particularly phlorotannins, have the potential to be used as therapeutic agents for the prevention and treatment of NDs associated with oxidative stress [23]. In the treatment of neurodegenerative disorders, cholinesterase (ChE) inhibitors have proven to be a successful approach for alleviating symptoms, although there exist various strategies to impede the progression of neurodegeneration. The isolation of phlorotannins from Ecklonia maxima revealed their capability to inhibit AChE activity. Among these compounds, dibenzo 1,4-dioxine-2,4,7,9-tetraol and eckol exhibited superior inhibitory effects on AChE compared to phloroglucinol. This enhanced potency can be attributed to their larger molecular size and increased number of hydroxyl groups, which modulate their interactions with AChE, ultimately leading to its blockade. These findings underscore the potential applications of Ecklonia maxima as a valuable ingredient that could be incorporated into food additives, serving as neuroprotective agents [4, 41, 42, 115].
Anti-inflammatory effects
Inflammation is a key contributor to the development and progression of NDs. Seaweed compounds have been shown to modulate the immune response, reducing inflammation, and preventing damage to nerve cells [117]. Fucoidan, a sulphated polysaccharide found in brown seaweed, has been shown to have potent anti-inflammatory effects and protect against inflammation-induced neurotoxicity [118].
Inflammation is a natural response of the body’s immune system to injury or infection. However, chronic inflammation can contribute to the development and progression of various diseases, including neurodegenerative disorders such as AD, PD, and multiple sclerosis (MS) [119, 120]. Chronic inflammation is associated with the activation of various immune cells and the release of pro-inflammatory cytokines, which can damage nerve cells and disrupt normal brain function [121, 122].
Seaweed, which is a rich source of bioactive compounds, has been studied for its potential anti-inflammatory effects. Fucoidan, a sulfated polysaccharide found in brown seaweed, has been shown to have potent anti-inflammatory properties. Fucoidan can modulate the immune response by inhibiting the activation of immune cells and reducing the production of pro-inflammatory cytokines [26, 123]. Studies have shown that fucoidan can protect against inflammation-induced neurotoxicity. For example, fucoidan treatment has been shown to reduce inflammation and protect against nerve cell damage in animal models of NDs such as AD and PD [19, 124].
In addition to fucoidan, other seaweed compounds such as phlorotannins and carotenoids have also been shown to have anti-inflammatory effects. These compounds can inhibit the activation of immune cells and reduce the production of pro-inflammatory cytokines, thereby reducing inflammation and protecting against NDs [117, 125]. Overall, the anti-inflammatory effects of seaweed compounds suggest that seaweed may have therapeutic potential for the prevention and treatment of NDs [126]. However, more research is needed to fully understand the mechanisms of action and potential benefits of seaweed compounds in the context of neuroinflammation and neurodegeneration [3].
Anti-apoptotic effects
Apoptosis, or programmed cell death, is a process that plays a role in the development and progression of NDs [127]. Apoptosis is a natural process that occurs in multicellular organisms to remove damaged or unnecessary cells. This process is crucial for the proper development and function of tissues, organs, and the immune system [128]. However, dysregulation of apoptosis can lead to various pathological conditions, including NDs such as AD, PD, and Huntington’s diseases. Seaweed compounds have been shown to inhibit apoptosis, preventing the death of nerve cells [129].
Anti-apoptotic effects refer to the ability of certain compounds to prevent or inhibit apoptosis. These compounds can target different components of the apoptotic pathway, including signaling molecules, transcription factors, and enzymes [130]. Seaweed compounds, specifically polysaccharides found in red seaweed, have been shown to possess anti-apoptotic effects. These compounds have been found to protect against apoptosis-induced neurotoxicity, which is the toxic effect on nerve cells caused by excessive apoptosis [131, 132].
Polysaccharides are complex carbohydrates that consist of many sugar units linked together. They are abundant in seaweed and have been shown to possess various biological activities, including antioxidant, anti-inflammatory, and immunomodulatory effects [133, 134]. Polysaccharides from red seaweed have been found to protect nerve cells against apoptosis by regulating the expression of apoptosis-related genes and modulating various signaling pathways [135]. In addition to red seaweed, other seaweed species have also been found to possess anti-apoptotic effects. For example, fucoidan, a sulfated polysaccharide found in brown seaweed, has been shown to protect against apoptosis-induced liver injury and promote the survival of liver cells [136].
The anti-apoptotic effects of seaweed compounds have great potential for the development of novel therapeutics for NDs and other pathological conditions associated with dysregulated apoptosis [137].
Conclusions
Seaweed and its bioactive compounds hold tremendous promise in the prevention and treatment of NDs. The available evidence points towards their potential therapeutic benefits, but further research is warranted to fully comprehend their mechanisms of action and establish their clinical efficacy [138]. Numerous studies have reported the antioxidant, anti-inflammatory, and neuroprotective properties of seaweed extracts and compounds, which could prove advantageous in impeding the progression or even preventing NDs such as AD, PD, and Huntington’s disease [139, 140]. Notably, certain seaweed-derived compounds like fucoidan and laminarin have demonstrated the ability to enhance cognitive function and memory in animal models of AD [141]. Despite these encouraging findings, it is important to acknowledge that the majority of research conducted on seaweed and NDs has relied on animal models or in vitro studies. Therefore, the next crucial step entails conducting more rigorous clinical trials to ascertain the safety and efficacy of seaweed-derived compounds in human subjects [142]. Such endeavors would bridge the gap between preclinical investigations and translational application, providing a more comprehensive understanding of the potential of seaweed as a therapeutic intervention for NDs.
In conclusion, while the existing evidence indicates the potential of seaweed and its bioactive compounds in the prevention and treatment of NDs, further research is imperative to unravel their effects and determine their clinical utility [143]. As experts in the field, we emphasize the need for concerted efforts to refine theoretical frameworks and methodological approaches, which will pave the way for a deeper comprehension of the importance of this research. The theoretical implications and translational applications of this study extend beyond the realms of neurodegeneration, providing valuable insights into the broader domain of natural compounds as potential therapeutic agents. By continuously advancing our knowledge in this area, we can unlock the full therapeutic potential of seaweed and contribute to the development of innovative strategies for combating NDs.
Abbreviations
AChE: | acetylcholinesterase enzyme |
AD: | Alzheimer’s disease |
Aβ: | amyloid β |
BACE1: | enzymatic reaction of β-secretase |
NDs: | neurodegenerative diseases |
PD: | Parkinson’s disease |
ROS: | reactive oxygen species |
Declarations
Author contributions
LP and AV: Conceptualization, Writing—original draft, Writing—review & editing. Both authors read and approved the submitted version.
Conflicts of interest
The authors declare that they have no conflicts of interest.
Ethical approval
Not applicable.
Consent to participate
Not applicable.
Consent to publication
Not applicable.
Availability of data and materials
Not applicable.
Funding
Not applicable.
Copyright
© The Author(s) 2023.