Abstract
Spinal cord injury (SCI) induces several destructive events that develop immediately after the primary insult. These phenomena increase tissue damage; that is why, numerous therapeutic approaches are studied in order to neutralize these destructive mechanisms. In line with this, several studies indicate that after injury, neural tissue could be protected by an adaptive immune response directed against self-antigens. Immunization with neural-derived peptides (INDP) reduces secondary degeneration of neurons after spinal cord insult and promotes a significant motor recovery. The combination of antioxidants or other immunomodulatory peptides after SCI can improve the protective effect induced by INDP. INDP in acute SCI is a promising strategy, so further studies should be addressed to be able to formulate the best strategy.
Keywords
A91, immunization, paraplegia, protective autoimmunityIntroduction
Spinal cord injury (SCI) is one of the most devastating phenomena comprising important neurological alterations. The incidence of this pathology in the United States ranges from 25 to 59 cases per million of individuals per year presenting an average of 40 per million [1]. The main causes include traffic accidents (38%), falls (31%) and sports (10–17%) [2].
Damage inflicted to the spinal cord results in permanent neurological deterioration of the motor, sensory and autonomic functions of the central nervous system (CNS). The final deterioration strongly depends on the extent of tissue damage which, in turn, depends on the intensity of the first injury mechanism and the secondary events developed immediately after the lesion [3]. Strategies aimed at neutralizing one or more of these secondary phenomena help to reduce the extent of damage (neuroprotection) and, consequently, promote better neurological recovery. Recent investigation has shown that modulating the immune system could be an innovative strategy to promote neuroprotection or even neuroregeneration [4, 5]. This therapeutic strategy has been achieved by immunizing with neural-derived peptides (INDP). To understand the bases of this therapy, in the first part, this manuscript will review the pathophysiology of SCI, and then the involvement of immune cells after injury will be described. Subsequently, the manuscript will explain how INDP modulates the immune system and promotes neuroprotection. Finally, this review will address the beneficial effects of INDP and its combination with other neuroprotective strategies after SCI.
Pathophysiology after SCI
After SCI, the damage is promoted by the primary and secondary mechanisms of lesion.
The primary damage is caused by the initial insult to the neural tissue, which could be induced by compression, contusion, or transection. This event triggers several destructive mechanisms that increase tissue damage.
Neurogenic shock, vascular insults, lipid peroxidation (LP), excitotoxicity, increased intracellular calcium, apoptosis, disturbance of mitochondrial function and inflammation are some of the secondary mechanisms of lesion. These phenomena strongly contribute to extend the damage to the surrounding neural tissue even further [6–8].
The neurogenic shock may exacerbate or promote neural tissue destruction. For instance, ischemia—the main consequence of neurogenic shock—results in the failure of important metabolic processes, thereby, inducing loss of energy and activating necrotic mechanisms. At the same time, ischemia promotes protease activation that degrade cytoplasmic membranes, as well as nuclear and cytoskeleton components [7, 9].
After ischemia, the reperfusion of neural tissue exacerbates the injury since; there is a large production of free radicals, mainly reactive oxygen species (ROS) [8, 10]. ROS are capable of attacking unsaturated fatty acids (mostly polyunsaturated) inducing a chain reaction and providing more free radicals; this process is known as LP [7, 11].
Excitotoxicity—another degenerative process—depends on the release and accumulation of excitatory neurotransmitters (i.e., glutamate) that produce direct or indirect damage to spinal cord tissue [12, 13]. This could be induced through the activation of N-methyl-D-aspartate (NMDA) receptors or by increasing intracellular calcium concentrations [8, 14].
Another dangerous phenomenon developed after injury is apoptosis. Cytokines, free radicals and excitotoxic molecules can trigger apoptosis, which induces cell death and then neurological deficit [7, 15].
Finally, a cellular inflammatory reaction also contributes to spinal cord damage. Inflammatory cells can induce the production of ROS and, consequently, contribute to LP.
One hour after injury, neutrophils reach the area [16, 17], increasing in number and peaking at 24 h after injury [17, 18]. Peripheral macrophages are present 24 h after insult and then increase until reaching their highest count between days four and seven [18, 19]. These cells can persist in the injured area even at chronic stages [20]. On the other hand, other inflammatory cells as microglia (resident macrophages) are activated within the epicenter of injury between three- and seven-days post-injury [21]. The presence of inflammatory cells at the lesion site has been strongly correlated with the amount of damage after injury [18, 22]. Previous studies have suggested that this inflammatory response is modulated by an autoimmune reaction against neural antigens, specifically against myelin basic protein (MBP), one of the most abundant and immunogenic proteins in the CNS [23–25]. In this autoimmune reaction, lymphocytes with the T helper (Th)1 phenotype prevail. If not regulated, these cells release pro-inflammatory cytokines that intensify the inflammatory response and increase tissue damage.
According to previous findings, regulation of this autoreactive response can promote neuroprotection or even neuroregeneration [26–29]. Therefore, the role of immune cells after SCI could be of relevant importance for establishing a therapeutic strategy.
Participation of immune cells after SCI
Several investigations have suggested that immune cells are capable of both, increasing or reducing tissue damage [25, 28, 30, 31]. This bi-directional effect depends on the final activation of microglial cells.
When microglia interact with molecules released after injury (lipids, complement molecules, ceruloplasmin, etc.), they acquire a destructive M1 phenotype [32–35]. These cells are characterized by releasing high levels of nitric oxide as well as pro-inflammatory substances such as tumor necrosis factor alpha (TNFα) and cyclooxygenase 2 (COX2) [32, 36–38].
These molecules intensify the inflammatory response, inducing a greater production of free radicals and proteases that, in turn, promote an increase in tissue destruction [35, 39, 40]. Under these circumstances, the microglia do not express major histocompatibility complex class II proteins (MHC-II) and, therefore, are unable to maintain an interaction with the adaptive immune system; an important condition for promoting neuroprotection [36, 41].
Additionally, T lymphocytes are recruited in small amounts and very late; thus, instead of controlling the immune response, the T cells collaborate to intensify the inflammatory reaction by releasing more pro-inflammatory cytokines [42].
On the other hand, if microglia interact with the adaptive immunity, specifically with T cells and/or T cell products [interferon-gamma (IFN-γ), interleukin-4 (IL-4)], they will be activated under an M2 phenotype assuming the properties of antigen-presenting cells leading to the expression of MHC-II and B7.2 proteins. As an antigen-presenting cell, these microglia can hold a dialog with T cells and are capable of both, releasing neurotrophic factors [brain derived neurotrophic factor (BDNF), neurotrophin-3 (NT-3), nerve growth factor (NGF)], and increasing the expression of glutamate transporters, thus facilitating the elimination of toxic compounds that endanger the tissue [43].
In this sense, a very interesting study demonstrated that autoimmune T cells could increase the ability of the microglia to remove glutamate without promoting nitric oxide production. This T-cell-mediated effect could not be achieved if activation of microglia is induced by lipopolysaccharides (LPS), but it was reproduced by the Th1 cytokine IFN-γ and significantly reduced by neutralizing anti-IFN-γ antibodies [44].
Another study showed that LPS or aggregated β-amyloid, confer a cytotoxic phenotype to microglia, while IFN-γ and IL-4 cytokines can make it neuroprotective [45]. Therefore, beneficial or harmful effects of immune response depends on how microglia are activated, and that a well-modulated T-cell-mediated response enables it to alleviate rather than exacerbate tissue damage.
Modulation of autoimmune response by INDP
It is realistic to envision that after SCI, the harmful effect exerted by immune cells could be reversed and modified to promote beneficial actions. To achieve this goal, it is crucial to avoid or diminish the activation of microglial cells towards an M1 phenotype.
In order to achieve this purpose, the therapeutic strategy should promote an earlier and larger arrival of T cells to the site of injury. This approach will induce the activation of microglia under the bases of a protective phenotype [36]. A simple way to make this possible is by immunizing with the same antigen that is inducing the autoreactive response: neural proteins. With this strategy (INDP), a large number of microglial cells will acquire the protective phenotype (M2) and then release molecules that, instead of increasing damage, will promote tissue protection (see Figure 1) [43–45].
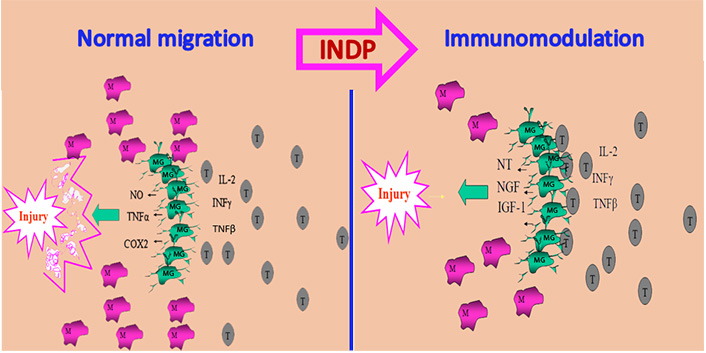
INDP promotes neuroprotection. After SCI microglia are activated as an M1 phenotype characterized by releasing cytotoxic substances and participating in tissue destruction. These cells are unable to interact with adaptive immunity, an important condition to promote neuroprotection. In the same circumstances, T lymphocytes are recruited in small amounts and very late, collaborating then, to intensify tissue damage. INDP induces an early and abundant recruitment of T cells allowing the activation of microglia under the M2 phenotype and promoting then neuroprotection. NO: nitric oxide; M: peripheral macrophages; MG: microglia; T: T lymphocytes; IL-2: interleukin-2; NT: neurotrophins; IGF-1: insulin-like growth factor-1
Note. Adapted from “Immunization with neural-derived peptides as a potential therapy in neurodegenerative diseases” by Mestre H, Ibarra A. In: Chang RCC, editor. Neurodegenerative diseases-processes, prevention, protection and monitoring. InTech; 2011 (https://www.intechopen.com/chapters/24852). CC BY.
This strategy—now called protective autoimmunity—supposes modulating the immune response by boosting an autoreactive reaction. In preliminary studies, the activation of myelin-specific autoreactive lymphocytes was modulated by immunizing with MBP. This therapeutic strategy promoted an improved neuroprotection and motor recovery after acute SCI [46]. Subsequent investigations demonstrated that “protective autoimmunity” is in fact a T-cell-dependent reaction that is genetically determined [47] and triggered as a physiological response to CNS trauma [48].
The promising results of this therapeutic immunization encouraged the design of subsequent studies now aimed at avoiding the risk of inducing an autoimmune disease. As immunization with self-constituents could predispose to the development of such pathological event, the boosting and modulation of this protective response by immunizing with non-encephalitogenic MBP-derived peptides, was envisioned as a safe approach for clinical purposes.
Modulation of protective autoimmunity with no risk of autoimmune disease
The possibility of inducing an autoimmune disease after immunization with MBP is perhaps the main complication of this therapy; however, it can be solved by immunizing with a weak self-antigen such as an altered peptide ligand (APL).
APLs are analogs of immunogenic epitopes with one (or a few) substitution(s) at the amino-acid positions essential for contact with the T-cell receptor (TCR), permitting them to compete for TCR binding. APLs affect TCR signaling changing T-cell differentiation or inducing an anergy state [49].
Some APLs derived from MBP (G91, A96 and A91) have already been investigated in SCI models. For instance, immunization with G91 or A96 induced a significant neuroprotection and thus a reduced extent of paralysis in animals with acute SCI. Noteworthy, immunized animals did not present any clinical signs of experimental autoimmune encephalomyelitis (EAE). Despite these promissory results, the use of these peptides in clinical trials is not perceived to be early, as no further studies have been reported [50].
This is not the case for A91, a peptide derived from MBP (sequence 87–99), by replacing the lysine residue 91 with alanine. A91 is an APL that cross-reacts with the original encephalitogenic peptide but activating weak self-reacting T-cells. This activation induces autoimmunity without the risk of promoting EAE [51–53]. Immunization with this peptide inhibits EAE but neither causing anergy nor clonal deletion [51]. During antigenic presentation, A91 instead of inducing a Th1 response promotes a Th2 phenotype which could regulate the Th1 reaction observed in EAE. After SCI, A91-immunization has also yielded promising results.
INDP after SCI
A single immunization with A91-peptide improved the motor recovery and neuronal survival of spinal cord injured rats [52, 53]. In another study, it was shown that post-injury injection of bone marrow-derived dendritic cells pulsed with A91, induced a significant and pronounced recovery from SCI [54]. The beneficial effect was also morphologically evident. Subsequent studies exploring the mechanisms of action shown that, A91-immunization inhibits lipid peroxidation [28] and can reduce apoptosis [29]. These neuroprotective effects are likely induced due to their inhibitory actions on nitric oxide production and inducible nitric oxide synthase gene expression [55]. Besides A91-immunization also promotes a long-term production of BDNF and NT-3 and induces an anti-inflammatory environment in the acute phase of SCI, provoking the release of high amounts of IL-4 and interleukin-10 (IL-10) cytokines [56, 57]. This microenvironment favors neuroprotection.
On the other hand, in order to improve the beneficial actions of INDP, a recent study explored the effect of administrating a double INDP. The investigation also analyzed the possible development of EAE. The results showed that neither single nor double INDP-dose developed an autoimmune disease [53]. The reported data offered an important light for the use of self-peptides as a therapeutic strategy for inducing neuroprotection with no risk of disease. The beneficial effects of A91-immunization have consistently been observed in models of acute SCI; however, recent investigation has also shown that, it could be useful in chronic stages of injury. In this case, it has been reported the induction of neurogenesis and motor recovery when immunization is initiated in the chronic phase of SCI [5, 58].
According to these observations, A91-immunization looks like a promising therapy for acute SCI. Thereby, to formulate the best strategy, further investigation is necessary. In line with this, the effect of combining this immunological therapy with methylprednisolone (MP)—the only therapeutic agent currently available for SCI—has been studied. The investigation showed that, when INDP was delayed for 48 h after injury, its beneficial effect was not inhibited by the anti-inflammatory action of MP injected immediately after the injury [52]. Therefore, immunization with A91 is neuroprotective even if administered 48 h after injury. Therapeutic INDP appears to be a promising strategy that could be adapted for the treatment of SCI in humans, even if MP is used.
At the moment, the findings in the field have been consistent and reproducible, especially in experimental models of moderate SCI. Nevertheless, in models of severe injury A91-immunization was ineffective [59]. Future studies should be aimed to optimize the strategy in this type of lesions.
Improving the beneficial effect induced by INDP
Although the positive effect of the INDP has rendered significant results, the improvement of this strategy would yield a better outcome for SCI affected individuals. The main action of several mechanisms of damage takes place during the acute stage of lesion [8]. As INDP response develops up to a few days after SCI, the tissue is unprotected during this time, allowing the destructive action of these phenomena. Therefore, a combination of INDP with another neuroprotective strategy could improve the functional outcome. LP is one of the most destructive phenomena developed immediately after SCI [28]; thereby, the addition of an antioxidant could attain a better neuroprotective effect. Glutathione monoethyl ester (GSHE) is a cell-permeant derivative of glutathione (GSH), which is an antioxidant with protective effects after SCI [60, 61]. Aside from this, GSH supports the proliferation, growth and differentiation of immune cells [62]. Accordingly, the addition of GSHE to INDP could significantly improve neuroprotection. Research on the subject showed that the use of this combination strategy induced earlier, and better motor recovery as compared to immunization alone. This neurological improvement was significantly correlated with better axonal myelination as well as with a higher survival of rubrospinal neurons [53]. In the same investigation, the effect of A91-double immunization was also tested as a possible way to improve the beneficial effect exerted by INDP. Contrary to the expectations, double immunization with A91 abolished the protective effect induced by the single dose. It is worth mentioning that the failure in protection was not the consequence of developing an autoimmune disease such as EAE.
In recent studies, INDP was also combined with the implantation—in the site of injury—of a biocompatible matrix impregnated with bone marrow mesenchymal stem cells (MSCs). This combined strategy improved neuroprotection, enhanced motor recovery and supported axonal regeneration [63]. Another strategy that also provided exiting results was the combination of INDP with GSHE and the monocyte locomotion inhibitor factor (MLIF), two immunomodulatory peptides. This combined therapy induced better motor recovery, preservation of the medullary parenchyma, white matter, motoneurons, and axonal fibers, and reduced collagen deposition in the injured area compared to INDP alone [64].
According to current data, INDP alone or in combination with other neuroprotective strategies is a promising strategy; however, more carefully planned studies are needed to establish the efficacy of this therapy.
Conclusions
At present, numerous studies have provided valuable information regarding the capacity of immune cells to promote protection and restoration of neural tissue. In this context, INDP has been envisioned as a promising approach for SCI therapy. Neural-derived peptides have shown to effectively stimulate the action of protective autoimmunity with no risk of developing an autoimmune disease. On the other hand, the therapeutic window after SCI can accommodate INDP and MP administration. Finally, the addition of other neuroprotective strategies improves the protective effect induced by INDP. The use of neural-derived peptides in acute SCI is a promising strategy; therefore, further experimental investigation and even the beginning of preclinical studies are encouraged in order to formulate the best strategy.
Abbreviations
APL: | altered peptide ligand |
CNS: | central nervous system |
EAE: | experimental autoimmune encephalomyelitis |
GSHE: | glutathione monoethyl ester |
IFN-γ: | interferon-gamma |
INDP: | immunization with neural-derived peptides |
IL-4: | interleukin-4 |
LP: | lipid peroxidation |
MBP: | myelin basic protein |
MP: | methylprednisolone |
ROS: | reactive oxygen species |
SCI: | spinal cord injury |
TCR: | T-cell receptor |
Th: | T helper |
Declarations
Acknowledgments
We thank MSc Estefania de la Cruz Castillo for helping to review the article before submission.
Author contributions
APIG wrote the first draft of the manuscript; AI reviewed, contributed conception and design of the manuscript; all authors contributed to manuscript revision, read and approved the submitted version.
Conflicts of interest
The authors declare no conflict of interest.
Ethical approval
Not applicable.
Consent to participate
Not applicable.
Consent to publication
Not applicable.
Availability of data and materials
Not applicable.
Funding
Universidad Anáhuac México Campus Norte has funded AI [CSNBTBIBAJ 090812 960]. The funders had no role in data collection and analysis, decision to publish, or preparation of the manuscript.
Copyright
© The Author(s) 2021.